Bidirectional regulation of glial potassium buffering – glioprotection versus neuroprotection
Figures
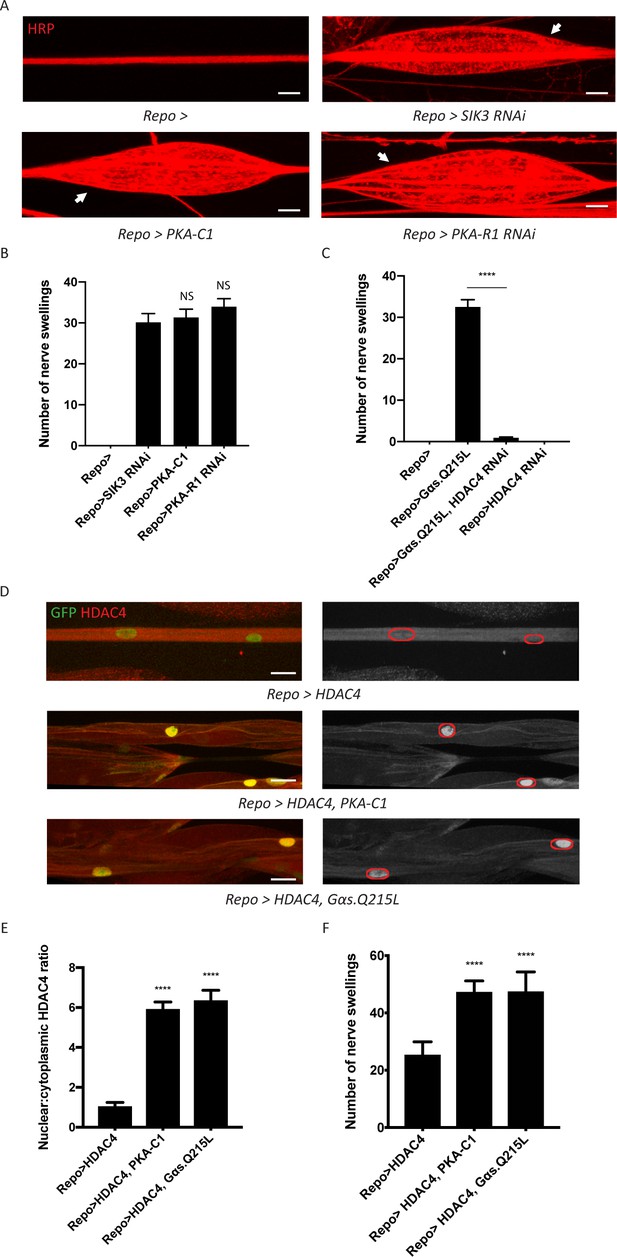
A G-protein-coupled receptor-protein kinase A (GPCR-PKA) signaling axis regulates salt inducible kinase 3 (SIK3)-mediated glial K+ buffering.
(A) Representative images of larval peripheral nerves stained for the nerve membrane marker HRP. Repo-GAL4, a pan-glial driver, was used to express LexA RNAi (control; abbreviated as Repo>), SIK3 RNAi (Repo>SIK3 RNAi), PKA-R1 RNAi (Repo>PKA-R1 RNAi), or a UAS-PKA-C1 transgene (Repo>PKA-C1). PKA hyperactivity, induced by either glial overexpression of its catalytic subunit (PKA-C1) or knockdown of a regulatory subunit (PKA-R1), causes localized nerve swellings (arrow) that resemble defects caused by loss of SIK3 from glia. Control larvae do not display swellings. Scale bars 20 μm. (B) Quantification of nerve swellings in (A). n ≥ 30. One-way ANOVA with Tukey's multiple comparisons; NS = not significant, p>0.05. (C) Quantification of nerve swellings per animal in control, larvae with glial expression of constitutively activated Gαs protein, HDAC4 RNAi, or co-expression of HDAC4 RNAi and Gαs. Constitutively activated Gαs in glia (Repo>Gsα. Q215L) causes nerve swellings that resemble (A); swellings are suppressed by loss of HDAC4 from glia (Repo>Gsα. Q215L, HDAC4 RNAi). Glial knockdown of HDAC4 (Repo>HDAC4 RNAi) does not result in nerve swellings. n ≥ 30. One-way ANOVA with Tukey's multiple comparisons; ****, p<0.0001. (D) Representative images of larval peripheral nerves demonstrating the effects of Gαs and PKA activation on HDAC4 localization in glia. Left: glial nuclei (green) and HDAC4 (red). Right: grayscale images show HDAC4 staining; glial nuclei are outlined. Scale bars 15 μm. (E) Quantification of nucleo:cytoplasmic ratio of HDAC4 for genotypes in (D). n ≥ 20. Data are presented as fold changes relative to Repo>HDAC4. One-way ANOVA with Tukey's multiple comparisons; ****, p<0.0001. (F) Quantification of number of nerve swellings per animal for genotypes in (D). n ≥ 20. One-way ANOVA with Tukey's multiple comparisons; ****, p<0.0001. Data are mean ± SEM.
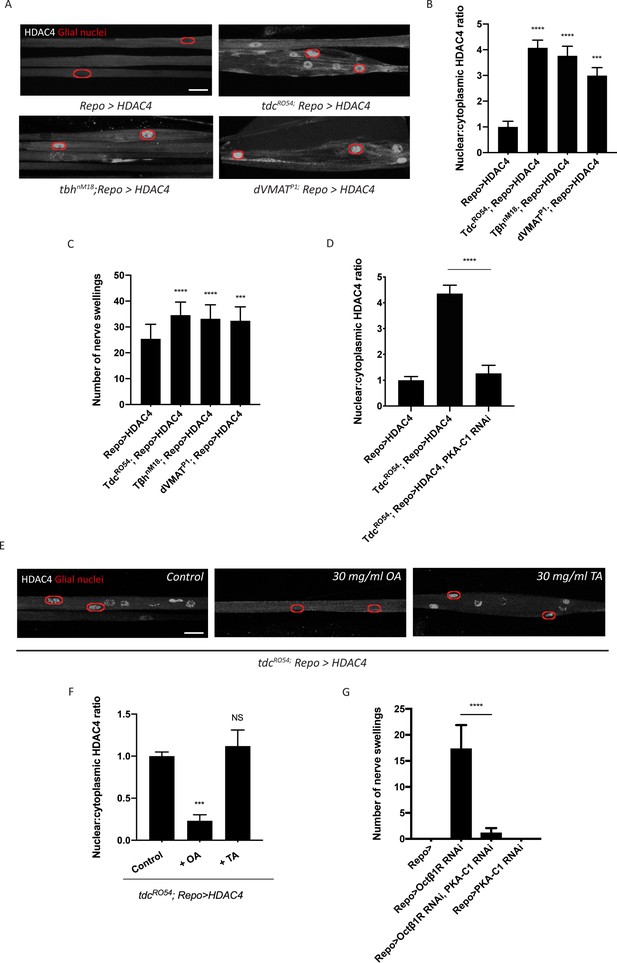
Octopamine is required to activate salt inducible kinase 3 (SIK3)-regulated K+ buffering program in glia.
(A) Representative images of peripheral nerves showing aberrant HDAC4 localization in octopamine synthesis and transport mutants. Grayscale images show HDAC4 staining; glial nuclei are outlined in red. Scale bars 15 μm. (B) Quantification of nucleo:cytoplasmic ratio of HDAC4 for genotypes in (A). n ≥ 20. Data are presented as fold changes relative to Repo>HDAC4. One-way ANOVA with Tukey's multiple comparisons; ***, p<0.001; ****, p<0.0001. (C) Quantification of number of nerve swellings per animal in HDAC4 overexpressing control larvae (Repo>HDAC4) and octopamine mutants. n ≥ 20 larvae per genotype. One-way ANOVA with Tukey's multiple comparisons; ***, p<0.001. ****, p<0.0001. (D) Quantification of HDAC4 localization in octopamine synthesis mutants, mutants with glial expression of LexA RNAi as control or PKA-C1 RNAi. Octopamine synthesis mutants (tdc2R054) exhibit HDAC4 accumulation in glial nuclei; this nuclear localization is rescued by abolishing protein kinase A (PKA) catalytic activity in glia (Repo>PKA-C1 RNAi). One-way ANOVA with Tukey's multiple comparisons; ****, p<0.0001. (E) Representative images of nerves demonstrating the effect of 30 mg/ml octopamine or 30 mg/ml tyramine on octopamine synthesis mutant glia in an ex vivo assay. Scale bars 15 μm. (F) Quantification of HDAC4 nucleo:cytoplasmic ratio for genotypes in (D). Octopamine (30 mg/ml), but not TA (30 mg/ml) or KCl (500 mM), suppresses HDAC4 nuclear localization in glia. n ≥ 15. One-way ANOVA with Tukey's multiple comparisons; ***, p<0.001; NS = not significant, p>0.05. (G) Quantification of number of nerve swellings per animal in larvae with glial expression of LexA RNAi as control (Repo>), Octβ1R RNAi, PKA-C1 RNAi, or co-expression of Octβ1R RNAi and PKA-C1 RNAi. n ≥ 20. Student's t test; ****, p<0.0001. Data are mean ± SEM.
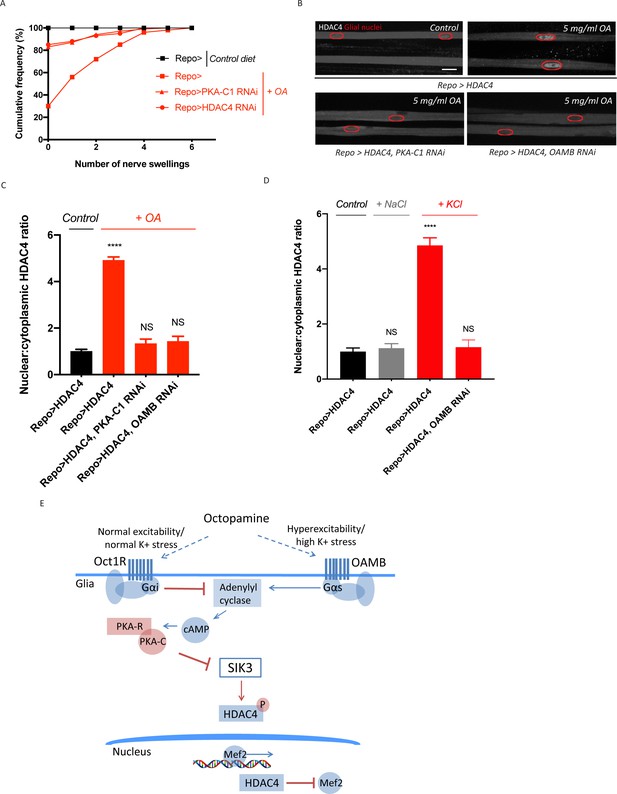
Excess octopamine inhibits salt inducible kinase 3 (SIK3) signaling to downregulate glial K+ buffering.
(A) Frequency of occurrence of nerve swellings in high octopamine-fed larvae with glial expression of LexA RNAi (Repo>), PKA-C1 RNAi, or HDAC4 RNAi. Larvae raised on control diet do not develop swellings; high octopamine (5 mg/ml) diet, but not high TA (5 mg/ml) diet, increases the percent of larvae exhibiting nerve swellings; this effect is blocked by loss of PKA-C1 or HDAC4 from glia. n ≥ 100. (B) Representative images of peripheral nerves demonstrating the effect of high octopamine diet on glial HDAC4 localization. High octopamine diet enhances HDAC4 nuclear localization in control larvae (Repo>HDAC4); this effect is blocked by glial knockdown of PKA-C1 (Repo> PKA-C1 RNAi) or OAMB (Repo>OAMB RNAi). Grayscale images show HDAC4 staining; glial nuclei are outlined in red. Scale bars 15 μm. High octopamine diet promotes nuclear localization of HDAC4 specifically in wrapping glia, as shown in Figure 3—figure supplement 1. (C) Quantification of HDAC4 nucleo:cytoplasmic ratio for genotypes in (B). n ≥ 30. Data are presented as fold changes relative to Repo>HDAC4. One-way ANOVA with Tukey's multiple comparisons; ****, p<0.0001. NS = not significant, p>0.05. (D) Quantification of HDAC4 localization in larvae raised on high salt diets. KCl-rich (200 mM) diet, but not NaCl-rich (200 mM) diet, promotes nuclear shuttling of HDAC4 in control larvae (Repo>HDAC4); this re-localization is inhibited by glial knockdown of OAMB (Repo>OAMB RNAi). n ≥ 20. One-way ANOVA with Tukey's multiple comparisons; ****, p<0.0001; NS, p>0.05. (E) Schematic model of octopamine exerting dual effects on SIK3-mediated glial K+ buffering: in response to K+ stress, different levels of octopamine act through receptors with antagonistic functions to differentially regulate SIK3-mediated glial K+ buffering. Data are mean ± SEM.
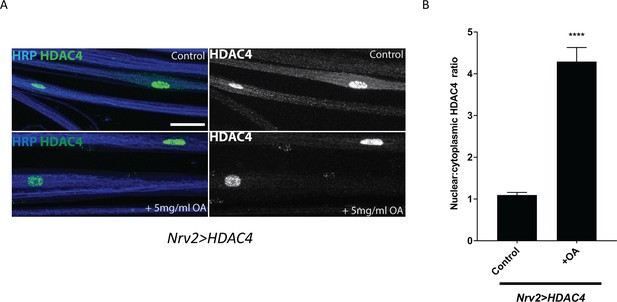
Excess octopamine in wrapping glia downregulates glial K+ buffering.
(A) Representative images of peripheral nerves showing HDAC4 localization in wrapping glia (Nrv2>HDAC4) of larvae raised on a normal or a high-octopamine diet. High octopamine leads to a high nucleo:cytoplasmic ratio of HDAC4. Grayscale images show HDAC4 staining. Scale bars 15 μm. (B) Quantification of HDAC4 nucleo:cytoplamic ratio for genotypes in (A). n ≥ 12. Data are presented as fold changes relative to Nrv2>HDAC4 + normal diet. Student's t test; ****, p<0.0001.
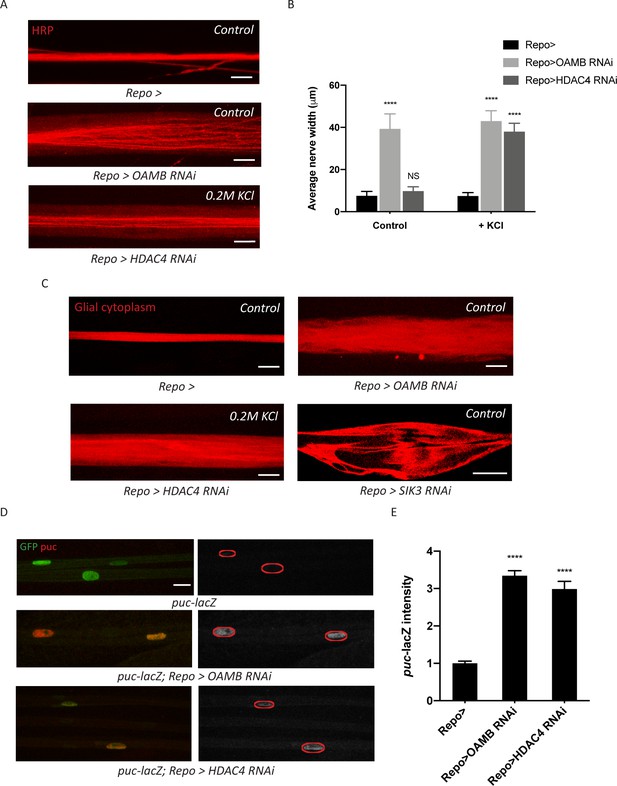
Glia with enhanced K+ buffering capacity undergo swelling and stress responses.
(A) Representative images of HRP-stained peripheral nerves demonstrating ‘non-localized’ edema in larvae with glial expression of LexA RNAi (Repo>), OAMB RNAi, or HDAC4 RNAi. Scale bars 20 μm. (B) Quantification of average nerve width for genotypes in (A). Loss of OAMB from glia causes ‘non-localized’ edema that results in a uniform increase in nerve width along the entire nerve; HDAC4 knockdown in glia induces a similar phenotype when larvae were fed a KCl-rich (200 mM) diet. n ≥ 15. Two-way ANOVA with Tukey's multiple comparisons; ****, p<0.0001; NS = not significant, p>0.05. (C) Representative thin optical sections of larval peripheral nerves with RFP-labeled (red) glial cytoplasm. (D) Representative images of larval peripheral nerves stained for c-Jun N-terminal kinase (JNK) pathway activity reporter puc-lacZ. Left: glial nuclei (green) and puc (red). Right: grayscale images show puc-lacZ staining; glial nuclei are outlined. Scale bars 15 μm. (E) Quantification of puc-lacZ signals in glia for genotypes in (C). Loss of OAMB or HDAC4 from glia dramatically increases puc expression in glia. n ≥ 20. One-way ANOVA with Tukey's multiple comparisons; ****, p<0.0001. Data are presented as fold changes relative to puc-lacZ; Repo>. Data are mean ± SEM.
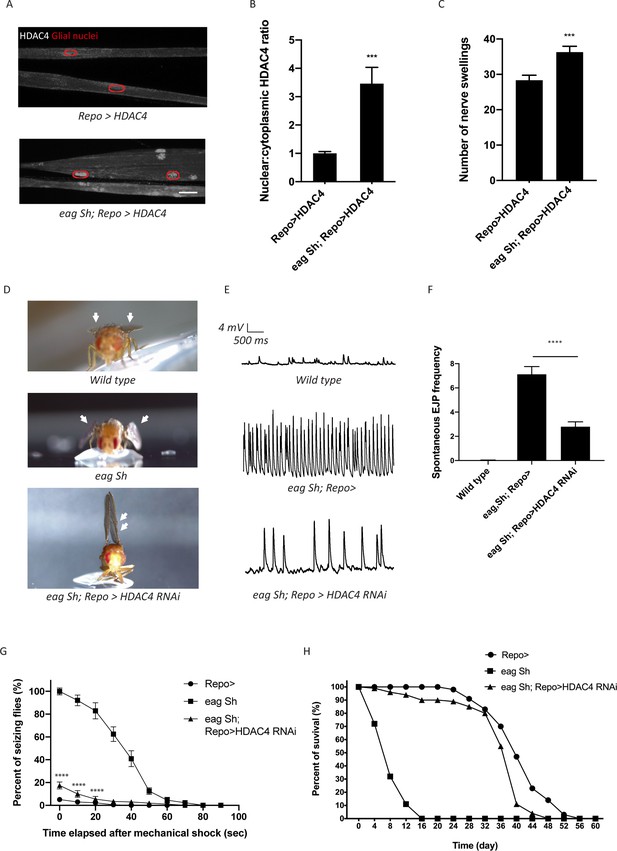
Enhanced glial K+ buffering suppresses hyperexcitability in eag,Sh mutant.
(A) Representative images of peripheral nerves demonstrating aberrant HDAC4 localization in eag,Sh mutant. Grayscale images show HDAC4 staining; glial nuclei are outlined in red. Scale bars 15 μm. (B) Quantification of HDAC4 nucleo:cytoplasmic ratio for genotypes in (A). n ≥ 20. Data are presented as fold changes relative to Repo>HDAC4. Student's t test; ***, p<0.001. (C) Quantification of nerve swellings in HDAC4-overexpressing control and eag shaker mutant. n ≥ 20. Student's t test; ***, p<0.001. (D) Representative images of wing morphology in control, eag,Sh mutants, and mutants with glial knockdown of HDAC4. Eag,Sh mutants exhibit down-turned wings (arrow) that are not observed in control flies; this phenotype is suppressed by glial-specific inhibition of HDAC4 (Repo>HDAC4 RNAi). (E) Representative physiological traces recorded from larval neuromuscular junctions (NMJs) for genotypes in (D). Control larvae (Repo>) only exhibit miniature excitatory junction potentials (mEJPs); eag,Sh exhibit spontaneous evoked junction potentials (EJPs). These spontaneous EJPs are suppressed by glial expression of HDAC4 RNAi. (F) Quantification of frequency of spontaneous EJPs for genotypes in (D). A minimum of 50 consecutive events were analyzed over a passive recording window (up to 75 consecutive events or 120 s, whichever happened first), and events with amplitudes ≥4 mV were considered as spontaneous EJPs. n = 8 for Repo>; n = 8 for eag,Sh; n = 8 for eag,Sh; Repo>HDAC4 RNAi. One-way ANOVA with Tukey's multiple comparisons; p<0.0001. (G) Time course of vortex-induced seizure behaviors for genotypes in (D). n ≥ 5 groups of 10 flies per genotype per time point. Two-tailed Student's t test; ****, p<0.0001. (H) Life span analysis of genotypes in (D). n ≥ 50. Data are mean ± SEM.