Evolutionary dynamics of transposable elements in bdelloid rotifers
Figures
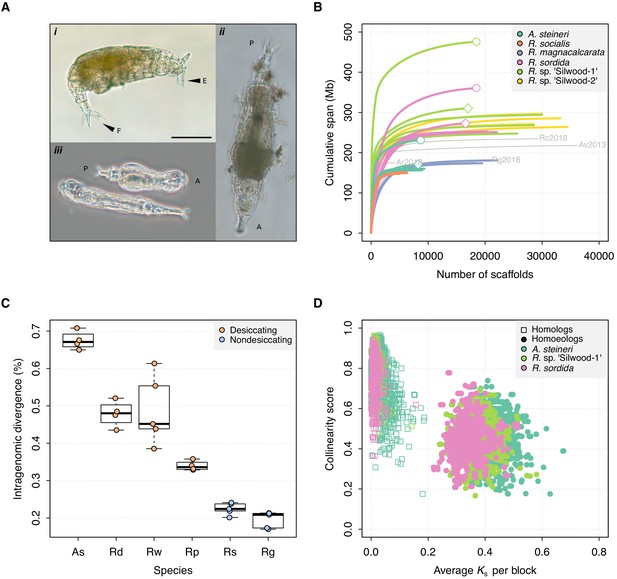
Genome properties of sequenced rotifers.
(A) Bdelloid rotifer morphology; scale bar indicates 100 µm. (i) Individual from an undescribed species of Rotaria (R. sp. ‘Silwood-1’), showing eyes (E) and foot (F) with two spurs and three toes. (ii) Further image of R. sp. ‘Silwood-1’ with anterior–posterior (A–P) axis marked. (iii) Two individuals of A. steineri in phase contrast illumination. (B) Cumulative assembly span for six bdelloid species with population genomics data (n > 2). 10x Genomics haploid (‘pseudohap’) and diploid (‘megabubbles’) assemblies for A. steineri, R. sordida and R. sp. ‘Silwood-1’ are indicated with diamond and circle symbols, respectively. The four previously published genomes for A. vaga (‘Av2013’, GenBank accession GCA_000513175.1) and A. ricciae (‘Ar2018’, GCA_900240375.1), R. macrura (‘Rc2018’, GCA_900239685.1) and R. magnacalcarata (‘Rg2018’, GCA_900239745.1) are indicated in grey, for comparison. (C) Intragenomic homologous divergence (i.e. heterozygosity), measured as the number of SNPs detected in coding regions (CDS). Boxplots show the median (band), interquartile range (box) and minimum/maximum values (whiskers). Underlying data are shown as jittered points. Desiccation-tolerant species are in orange, intolerant species in blue. Species abbreviations: As, A. steineri; Rd, R. sordida; Rw, R. sp. ‘Silwood-1’; Rp, Rotaria sp. ‘Silwood-2’; Rg, R. magnacalcarata; Rs, R. socialis. (D) Genome structure in A. steineri, R. sordida and R. sp. ‘Silwood-1’ haplotype-resolved (‘megabubbles’) assemblies. Each point represents a collinear block of genes, plotted by average pairwise synonymous (KS, X-axis) and collinearity score (see Materials and methods and S1 note) on the Y-axis. Separation into two distinct clusters representing homologous (squares) and homoeologous (circles) relationships among gene copies is consistent with ancestral tetraploidy, with homoeologous copies derived from a putative ancient genome duplication.
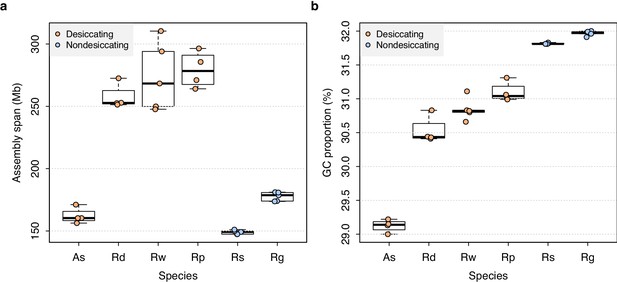
Genome characteristics for bdelloid samples.
Boxplots are shown for (a) genome assembly span and (b) GC proportion (percent G + C nucleotides). Data shown for species with n ≥ 3 individuals. Boxplots show the median (band), interquartile range (box), and minimum/maximum values (whiskers). Underlying data are shown as jittered points.
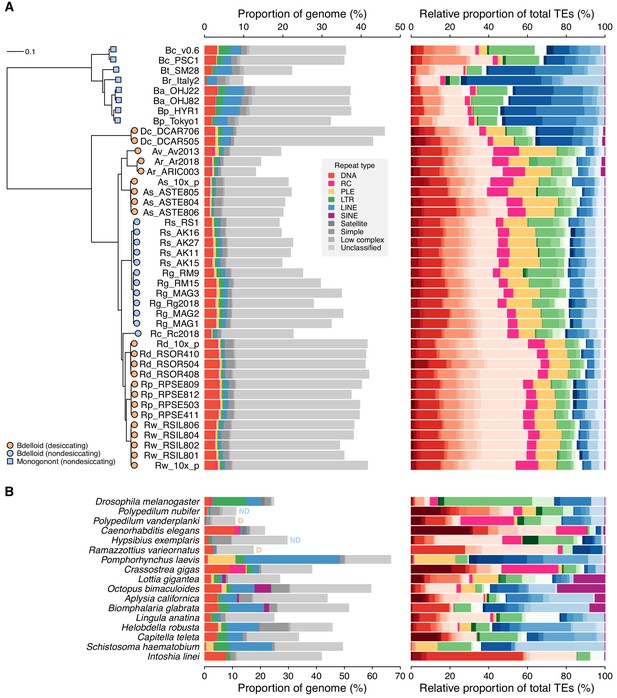
Repeat content and diversity in rotifer genomes.
(A) Maximum likelihood phylogeny of eight monogonont (square symbols on tips) and 34 bdelloid (circles) genomes based on the concatenated alignment of a subset of core eukaryotic (BUSCO) proteins. Orange and blue tip colours indicate desiccating and nondesiccating taxa, respectively. Scale bar represents 0.1 amino acid substitutions per site. Species codes in tip names are: Bc, Brachionus calyciflorus; Br, B. rotundiformis; Bt, B. sp. ‘Tiscar’; Bp, B. plicatilis HYR1; Ba, B. asplanchnoidis; Dc, Didymodactylos carnosus; Av, Adineta vaga; Ar, A. ricciae; As, A. steineri; Rs, Rotaria socialis; Rg, R. magnacalcarata; Rc, R. macrura; Rd, R. sordida; Rw, R. sp. ‘Silwood-1’; Rp, R. sp. ‘Silwood-2’. Repeat content is shown as the genome proportion (%) broken down by TE superfamily (middle panel), and relative proportion (%) of total known (i.e. classified) TEs (right panel), where colours represent TE superfamilies (see legend) and shades of colour represent different TE families within each superfamily. (B) Equivalent repeat content analysis in 17 protostome animal genomes, including the model species D. melanogaster and C. elegans, the recently published acanthocephalan rotifer P. laevis, and selected other species from across the protostome group. Two further examples of desiccating (orange ‘D’) and nondesiccating (blue ‘ND’) species pairs are shown: the insects P. nubifer and P. vanderplanki and the tardigrades H. exemplaris and R. varieornatus.
-
Figure 2—source data 1
Repeat content data for rotifers and other animals.
- https://cdn.elifesciences.org/articles/63194/elife-63194-fig2-data1-v3.xlsx
-
Figure 2—source data 2
Protein alignments and tree files for rotifer and protostome genomes.
- https://cdn.elifesciences.org/articles/63194/elife-63194-fig2-data2-v3.zip
-
Figure 2—source data 3
Genome properties of 17 protostome animals included in the study.
- https://cdn.elifesciences.org/articles/63194/elife-63194-fig2-data3-v3.xlsx
-
Figure 2—source data 4
Model output for phylogenetic models testing for significant shift in TE frequency on bdelloid stem branch compared to background, and MEDUSA-like test for significant shifts in rate of TE evolution on phylogenetic tree of bdelloids, monogononts, and outgroups.
- https://cdn.elifesciences.org/articles/63194/elife-63194-fig2-data4-v3.docx
-
Figure 2—source data 5
Posterior mean and 95% credible intervals for the effect of desiccation ability on TE load from a phylogenetic linear model.
- https://cdn.elifesciences.org/articles/63194/elife-63194-fig2-data5-v3.docx
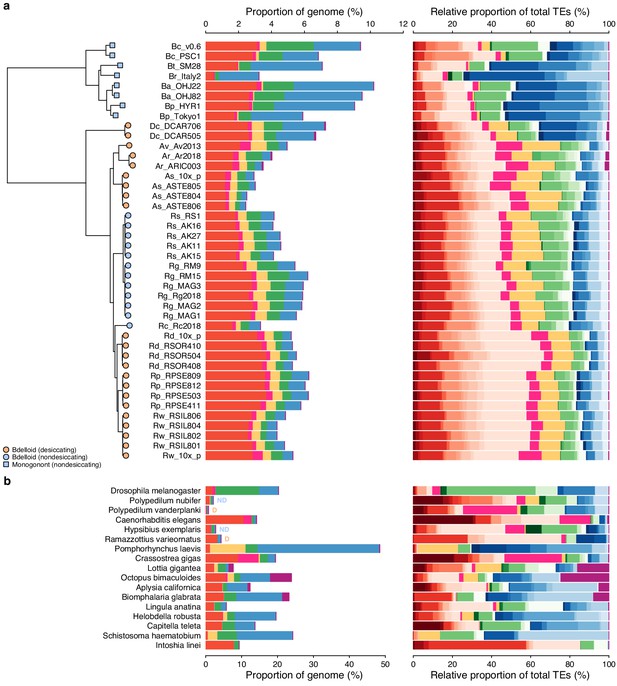
Repeat content and diversity in 42 rotifer and 17 protostome animal genomes.
Plot is the same as in main text but data for satellite, simple, low complexity, and unclassified repeats are omitted.
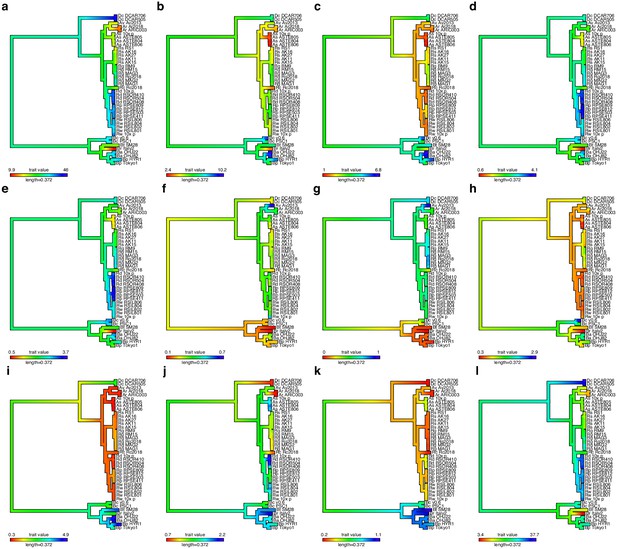
TE content mapped as continuous trait onto rotifer phylogeny.
Plots a–l show total repeats (including unknown), known TEs (i.e. that are classified into one of the major class I or II superfamilies), total class I, total class II, DNA transposons rolling circles, PLEs, LTRs, LINEs simple repeats, low complexity repeats, and unclassified (unknown) repeats, respectively. Repeat content is mapped as a percentage of genome span, with blue indicating a relative increase and red indicating a relative decrease in TE type along a given branch of the phylogeny.
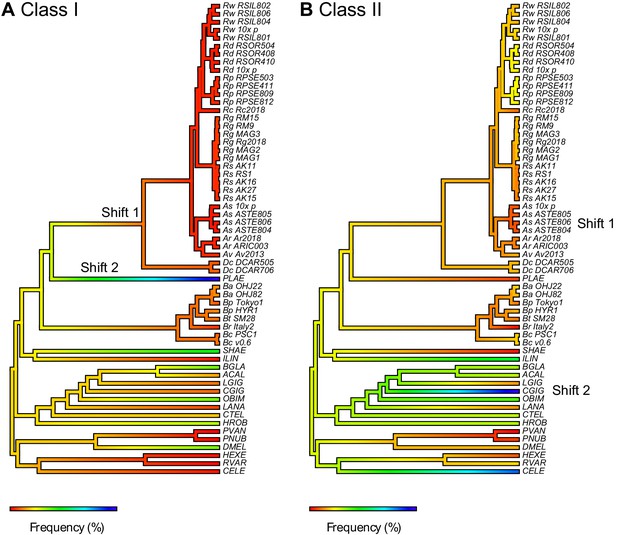
TE content mapped as a continuous trait onto protostome phylogeny.
Frequency of (A) Class I and (B) Class II TEs shown separately. Repeat content is mapped as a percentage of genome span, with blue indicating a relative increase and red indicating a relative decrease in TE type along a given branch of the phylogeny. Significant shifts in the frequency or rate of change are indicated on branches with yellow stars (see Figure 2—source data 4 for details on model output).
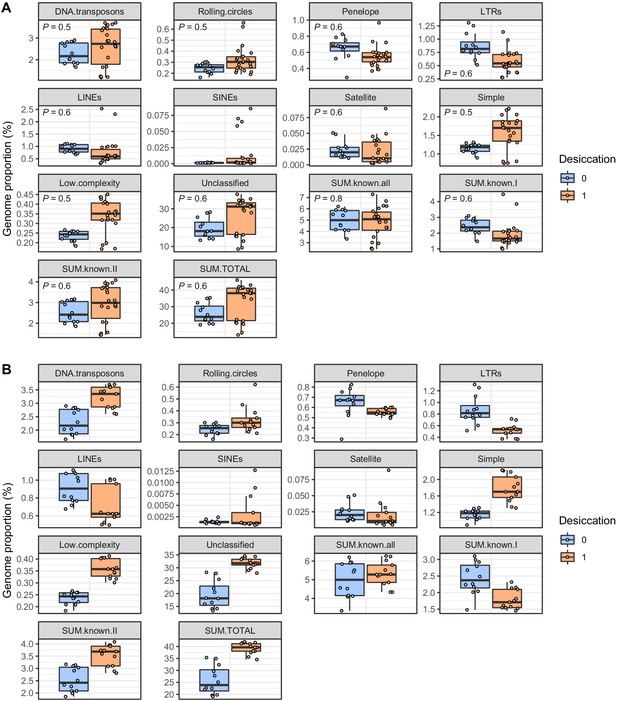
Boxplots showing the raw data for repeat content in desiccating versus nondesiccating bdelloids, shown for (A) all bdelloids and (B) Rotaria lineages only.
Data is plotted as the proportion (%) of genome span accounted for each repeat type. FDR-adjusted p-values for phylogenetic linear models testing for significant differences between desiccating and nondesiccating species (accounting for phylogeny) are shown for each TE subclass in panel A (all nonsignificant). Desiccation tolerance indicated in blue (nondesiccating) and orange (desiccating). Boxplots show the median (band), interquartile range (box), and minimum/maximum values (whiskers). Underlying data are shown as jittered points. Summed categories defined as follows: ‘Known all’, all classified TEs (i.e. satellite, simple, low complexity, and unclassified excluded); ‘Known I’, class I retrotransposon families; ‘Known II’, class II DNA transposon families; ‘Total’, grand total of all repeats.
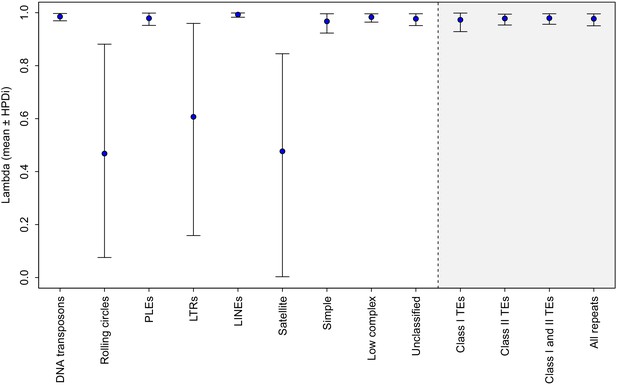
Phylogenetic signal (λ) in TE load variation among rotifer lineages, defined as the proportion of the total variance in TE load attributable to the phylogeny (de Villemereuil and Nakagawa, 2014).
The value of λ indicates how well the phylogeny predicts patterns of covariance among species (Pagel, 1994). Values closer to one indicate a stronger phylogenetic effect, consistent with TE content evolving according to neutral genetic drift (i.e. under a constant-variance Brownian motion model). Error bars show the 95% credible interval around the mean value of λ from 2000 stored samples of the posterior.
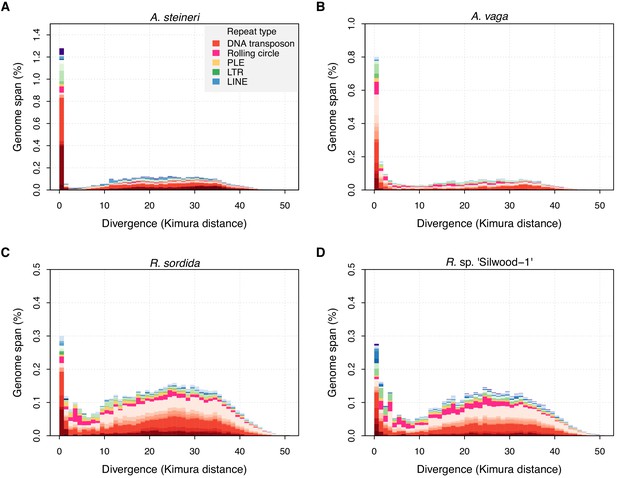
TE divergence landscapes for selected genomes.
The X-axes show the level of divergence (Kimura substitution level, CpG adjusted) between each identified TE copy and the consensus sequence for that TE family (the inferred ancestral copy). Thus, if newly arising TE copies evolve neutrally, the amount of divergence is a proxy for the time since its duplication, with older copies accumulating more substitutions and appearing further to the right. The Y-axis shows the proportion of the genome occupied by each bin. Colours represent TE superfamilies (see legend) and shades of colour represent different TE families within each superfamily. Data are shown for the 10x Genomics diploid assemblies of A. steineri, R. sp. ‘Silwood-1’ and R. sordida compared to the published genome of A. vaga. Note different scales on some Y-axes.
-
Figure 3—source data 1
Mapping results for species with available RNA-seq data, showing read coverage for annotated TEs based on RNA-seq data mapped to the corresponding genome.
- https://cdn.elifesciences.org/articles/63194/elife-63194-fig3-data1-v3.xlsx
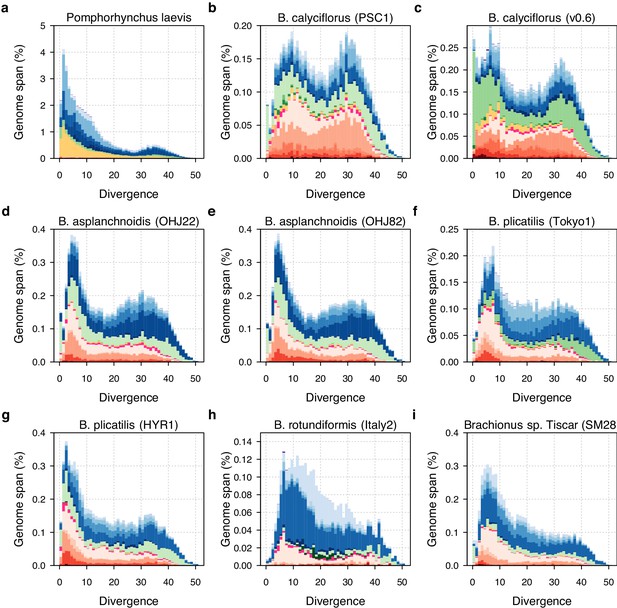
TE divergence landscapes for individual Brachionus genomes.
The X-axis shows the level of divergence (Kimura substitution level, CpG adjusted) between each identified TE copy and the computed consensus sequence for that TE family (inferred ancestral copy). Thus, if newly arising TE copies evolve neutrally, the amount of divergence is a proxy for the time since duplication for each copy, with older copies accumulating more substitutions and appearing further to the right on the X-axis. The Y-axis shows the proportion of the genome occupied by each TE divergence category, broken down by family where shades of colour indicate TE superfamily (red, DNA transposons; pink, rolling circles; yellow, PLEs; green, LTRs; blue, LINEs; purple, SINEs; see legend in main text).
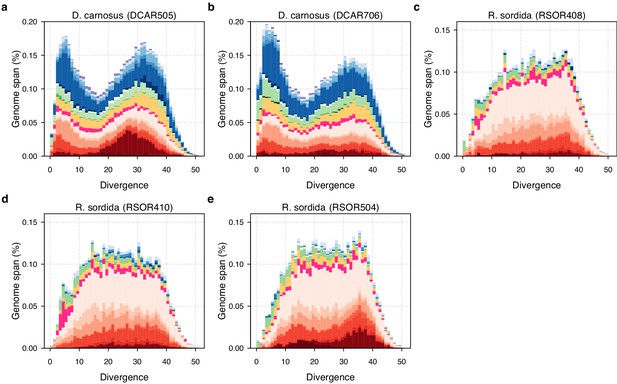
TE divergence landscapes for individual D. carnosus and R. sordida genomes.
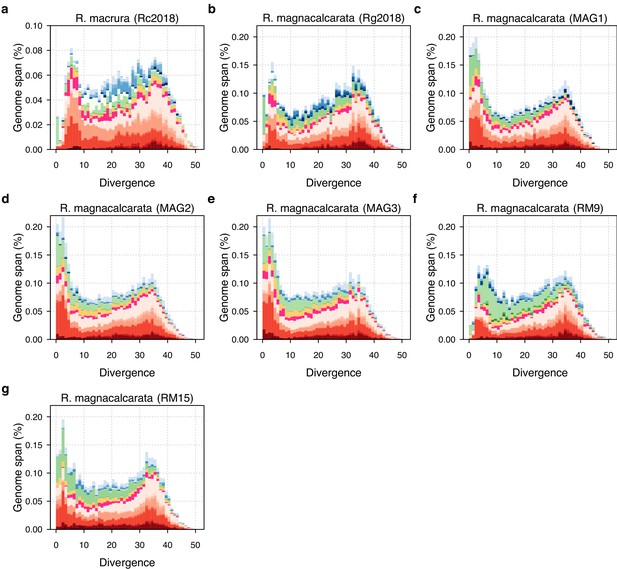
TE divergence landscapes for individual R. macrura and R. magnacalcarata genomes.
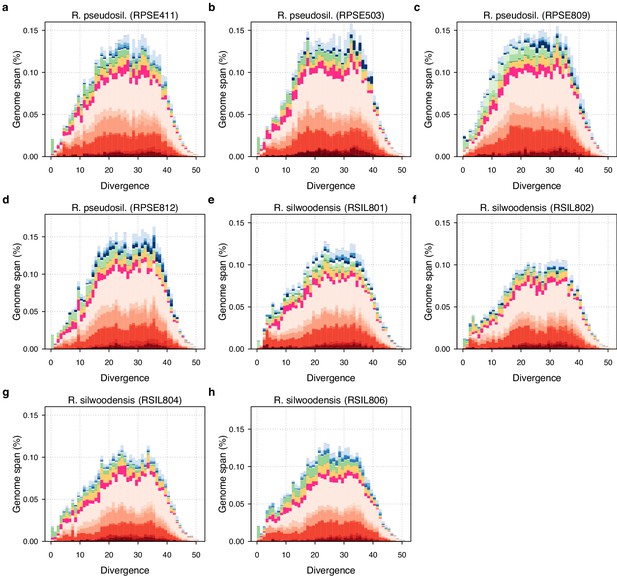
TE divergence landscapes for individual R. sp. ‘Silwood-1’ and R. sp. ‘Silwood-2’ genomes.
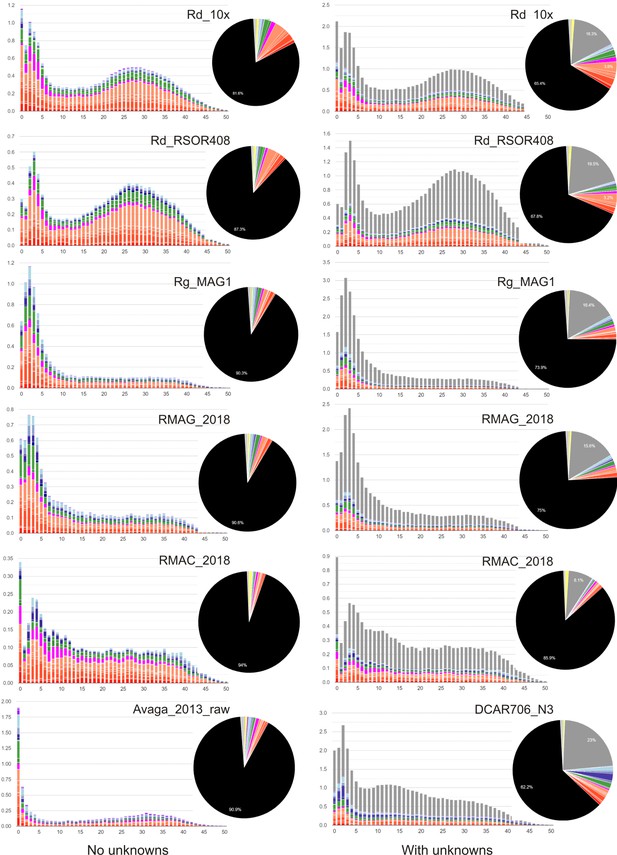
TE divergence landscapes for selected species constructed using REPET (unfiltered, with default parameters).
Landscapes and axes are arranged as previously. Pie charts show TE superfamilies occupying ≥2% of a given genome. Colour scheme from RepeatMasker v.4.1.0: orange, cut-and-paste DNA TEs; magenta, rolling-circle DNA TEs; green, LTR-Rs; dark blue, LINEs; light blue, PLEs; yellow, simple repeats; grey, unknown (unclassified). Note a greater proportion of repeats at lower divergence values (K-distance <5).
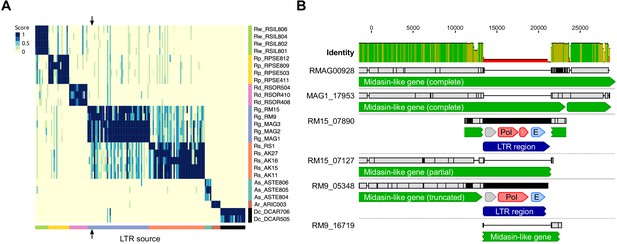
LTR insertion-site polymorphism in bdelloid species.
(A) Columns represent 161 LTR-Rs identified across bdelloid samples, arranged by genome of origin (see colours at bottom and side). Support for the presence of a given LTR-R at a specific insertion site in each genome is scored from 0 (absent, yellow) to 1 (present, dark blue), where a score <0.5 is strong evidence for absence (see Materials and methods for details). Arrows demark the column corresponding to the LTR-R example shown in (B). (B) Nucleotide alignment of region around an LTR-R insertion (blue) identified in RM9 (scaffold 05348) and RM15 (scaffold 07890), alongside their putative homologous scaffolds (scaffolds 16719 and 07127 respectively) that do not show the insertion. Scaffolds from Rg2018 (RMAG00928) and MAG1 are also shown for comparison. Predicted CDS with similarity to Pol and Env proteins are shown in red and light blue. The LTR-R is most likely a member of the TelKA family, based on sequence similarity.
-
Figure 4—source data 1
LTR-tag fasta file and mapping data.
- https://cdn.elifesciences.org/articles/63194/elife-63194-fig4-data1-v3.zip
-
Figure 4—source data 2
Analysis of recombination in LTR-tag presence/absence data.
- https://cdn.elifesciences.org/articles/63194/elife-63194-fig4-data2-v3.docx
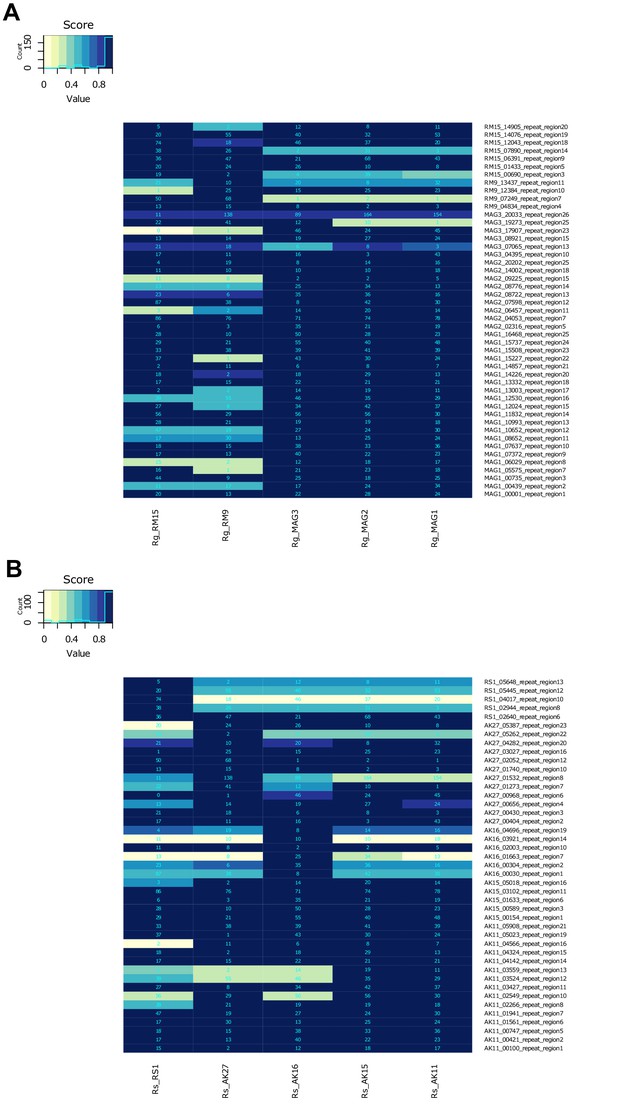
LTR-R polymorphism details.
Data are shown for (A) R. magnacalcarata and (B) R. socialis. Numbers in cells indicate the number of reads mapped to the LTR-tag location (see Materials and methods).
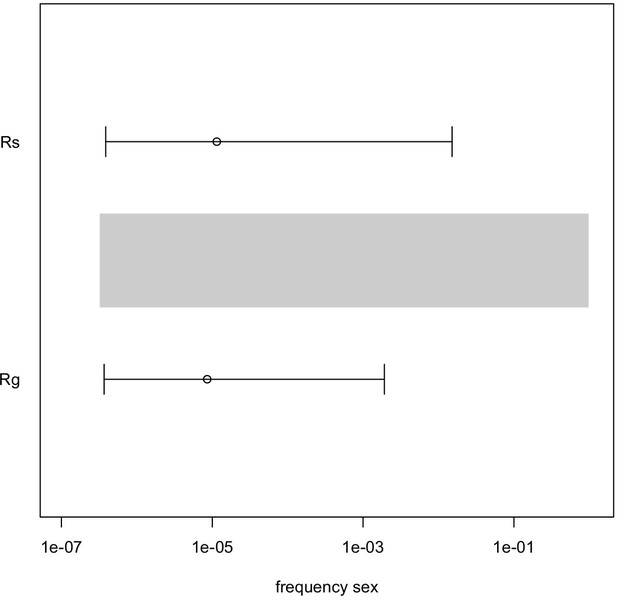
The median and 95% Highest Posterior Density interval of the frequency of sexual recombination affecting the presence/absence of LTR polymorphisms in R. magnacalcarata and R. socialis.
The grey bar shows the prior distribution used in simulations for the Approximate Bayesian Computation analysis. Confidence limits are broad but encompass the lowest range of the prior and hence cannot reject the hypothesis that recombination is absent for these loci.
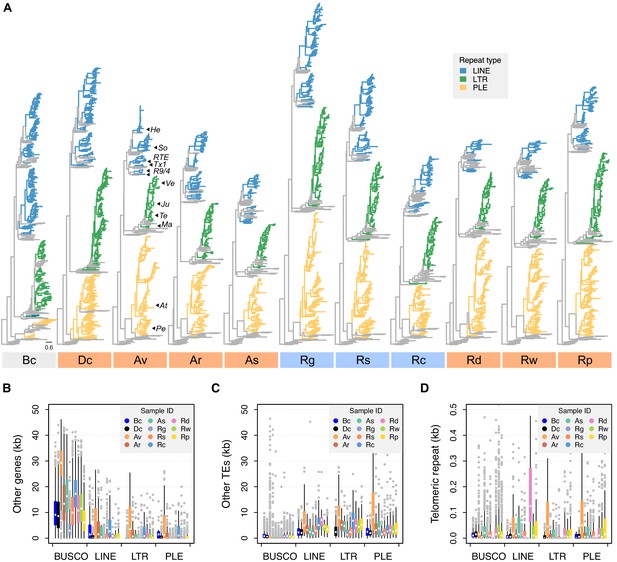
Phylogenetic diversity and genomic context of reverse transcriptase (RT) genes.
(A) For each phylogeny, coloured branches represent identified rotifer-encoded RT copies and grey branches represent the core RT sequences from which the hidden Markov model (HMM) was built (see Figure 5—figure supplement 1 for core RT tree details). Colours indicate the major superfamilies. Previously characterized retrotransposons are indicated on the A. vaga tree (He, Hebe; So, Soliton; RTE, RTE; Tx1, Tx1; R9/4, R9 and R4; Ve, Vesta; Ju, Juno; Te, TelKA; Ma, Mag; At, Athena; Pe, Penelope). All phylogenies are rooted on the branch separating the bacterial retrons. Scale bar represents 0.6 amino acid substitutions per site. Desiccating and nondesiccating species are indicated with orange and blue, as previously. Species codes: Bc, B. calyciflorus PSC1; Dc, D. carnosus DCAR706, Av, A. vaga Av2013; Ar, A. ricciae ARIC003; As, A. steineri ASTE805; Rg, R. magnacalcarata MAG3; Rs, R. socialis AK11; Rc, R. macrura Rc2018; Rd, R. sordida RSOR408; Rw, R. sp. ‘Silwood-1’ RSIL806; Rp, R. sp. ‘Silwood-2’ RPSE503. The genomic context in which RT genes reside is then described based on proximity to three other features: (B) other genes (that do not overlap with any TE annotation), (C) other TEs, and (D) telomeric repeats (‘TGTGGG’; that do not overlap with any coding region) as identified in A. vaga. For each plot, a 50 kb window is drawn around the focal TE and the total span (kb) of each feature within the window is counted, broken down per sample ID (coloured boxes, see legend) per TE superfamily (X-axis groups). Boxplots show the median (band), interquartile range (box) and minimum/maximum values (whiskers; outliers are shown in grey). The equivalent data for BUSCO genes (metazoan set) are also shown for comparison. The same set of individuals are shown in (B–D) as for (A). Average values (mean ± SD) across monogononts and bdelloids (desiccating and nondesiccating) are provided in Figure 5—source data 4.
-
Figure 5—source data 1
Reverse-transcriptase alignments and phylogenies.
- https://cdn.elifesciences.org/articles/63194/elife-63194-fig5-data1-v3.zip
-
Figure 5—source data 2
Identification of putative telomeric repeats in rotifer genomes.
- https://cdn.elifesciences.org/articles/63194/elife-63194-fig5-data2-v3.docx
-
Figure 5—source data 3
Posterior mean and 95% credible intervals for the effects of asexuality and desiccation ability on the density of three features (other genes, other TEs and telomeric repeats) surrounding LINE, LTR, and PLE class I TEs, compared to BUSCO genes.
- https://cdn.elifesciences.org/articles/63194/elife-63194-fig5-data3-v3.docx
-
Figure 5—source data 4
Mean and standard deviation (SD) for the span (kb) of features ‘other genes’, ‘other TEs’ and ‘telomeric repeats’ occurring in 50 kb windows around genes of type BUSCO, LINE, LTR, or PLE, averaged across monogononts versus bdelloids and desiccating versus nondesiccating bdelloids.
- https://cdn.elifesciences.org/articles/63194/elife-63194-fig5-data4-v3.docx
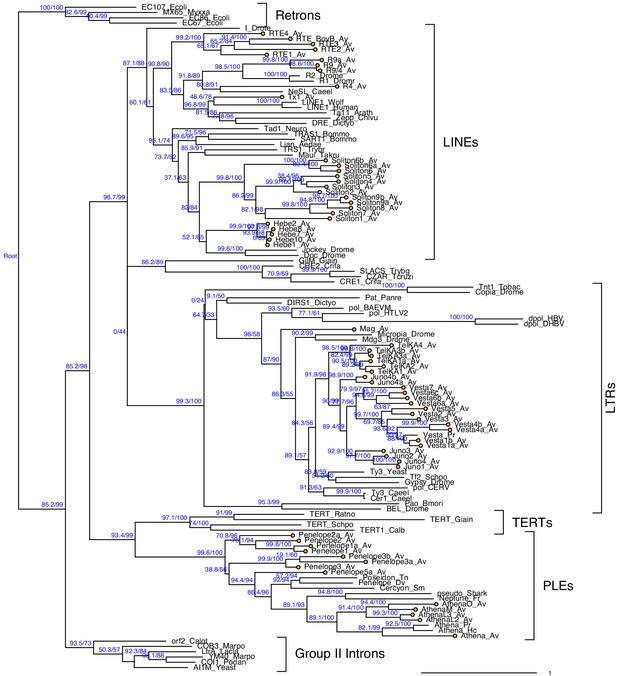
Maximum likelihood phylogeny for diverse reverse transcriptase domains from across the tree of life.
Major RT clades are indicated with labels. Sequences from the bdelloid A. vaga are indicated with orange tip labels and the suffix ‘Av’ (see Arkhipova et al., 2003; Flot et al., 2013 for further details). Phylogeny is rooted on branch leading to bacterial retrons. Numbers on branches represent branching support (%) based on 1000 SH-aLRT and 5000 UFBoot samples, respectively. Scale bar indicates one amino acid substitution per site.
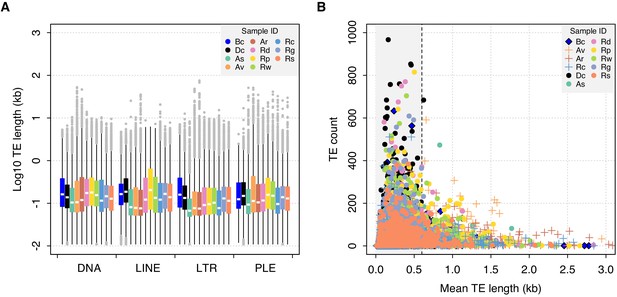
TE length dynamics.
(A) Distribution of TE length for selected syndermatan samples decomposed into the major TE superfamilies (DNA transposons, LINE, LTR, and PLE retrotransposons). Boxplots show the median (band), interquartile range (box) and minimum/maximum values (whiskers; outliers are shown in grey). Species codes: Bc, B. calyciflorus PSC1 (monogonont); Dc, D. carnosus DCAR706; As, A. steineri ASTE804; Av, A. vaga Av2013; Ar, A. ricciae Ar2018; Rd, R. sordida RSOR408; Rp, R. sp. ‘Silwood-2’ RPSE411; Rw, R. sp. ‘Silwood-1’ RSIL801 (desiccating bdelloids); Rc, R. macrura Rc2018; Rg, R. magnacalcarata MAG1; Rs, R. socialis AK11 (nondesiccating bdelloids). An equivalent plot including the acanthocephalan P. laevis is shown in Figure 6—figure supplement 1. (B) Relationship between mean TE length per TE family (X-axis) and copy number (i.e. the number of TEs identified within each family; Y-axis). The same set of individuals are shown as for (A). A dashed line is drawn at 0.6 kb, given as the length threshold below which the rate of homologous ectopic recombination is negligible in mice.
-
Figure 6—source data 1
TE length raw data.
- https://cdn.elifesciences.org/articles/63194/elife-63194-fig6-data1-v3.zip
-
Figure 6—source data 2
Posterior mean and 95% credible intervals for the effects of asexuality desiccation ability on TE length.
- https://cdn.elifesciences.org/articles/63194/elife-63194-fig6-data2-v3.docx
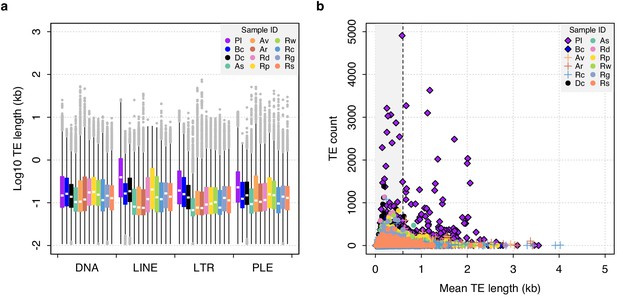
Distribution of TE lengths including the acanthocephalan P. laevis.
Boxplots show the median (white band), interquartile range (box) and minimum/maximum values (whiskers; outliers shown in grey).
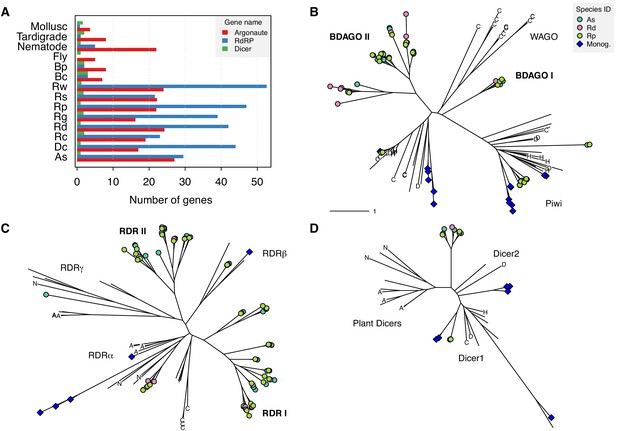
Expansion of TE silencing pathways in bdelloid rotifers.
(A) Copy number variation for RNAi gene families Argonaute (Ago/Piwi, red), RNA-dependent RNA polymerase (RdRP, blue) and Dicer (green) in bdelloids compared to other protostome groups. Proteins are identified based on the presence of key identifying domains (see Materials and methods). Species codes for rotifers: Bc, B. calyciflorus; Bp, B. plicatilis HYR1; Dc, D. carnosus; As, A. steineri; Rg, R. magnacalcarata; Rs, R. socialis; Rc, R. macrura; Rd, R. sordida; Rw, R. sp. ‘Silwood-1’; Rp, R. sp. ‘Silwood-2’. Maximum likelihood unrooted phylogenies are then shown for (B) Argonaute, (C) RdRP and (D) Dicer gene copies identified in A. steineri, R. sordida and R. sp. ‘Silwood-1’ 10x haploid assemblies, aligned with orthologs from representative species from across the eukaryotes. Blue symbols indicate copies identified in the monogonont B. plicatilis, and letters on tips show selected reference species to aid visual orientation: ‘C’, C. elegans; ‘H’, human; ‘D’, D. melanogaster; ‘N’ N. crassa; ‘A’, A. thaliana. Some clade names are also shown where relevant; ‘WAGO’ indicates the worm-specific cluster of Ago genes in the Argonaute phylogeny. ‘BDAGO I/II’ and ‘RDR I/II’ indicate putative bdelloid-specific clades of Argonaute and RdRP proteins, respectively.
-
Figure 7—source data 1
RNAi gene family expansions.
- https://cdn.elifesciences.org/articles/63194/elife-63194-fig7-data1-v3.xlsx
-
Figure 7—source data 2
Argonaute, Dicer, and RdRP alignments and phylogenies.
- https://cdn.elifesciences.org/articles/63194/elife-63194-fig7-data2-v3.zip
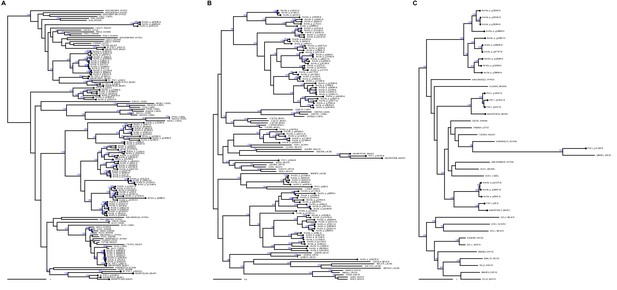
Argonaute, RdRP, and Dicer phylogeny details.
Bdelloid copies are indicated with tip labels. Numbers on branches represent branching support (%) based on 5000 UFBoot samples, respectively. Scale bar indicates one amino acid substitution per site.
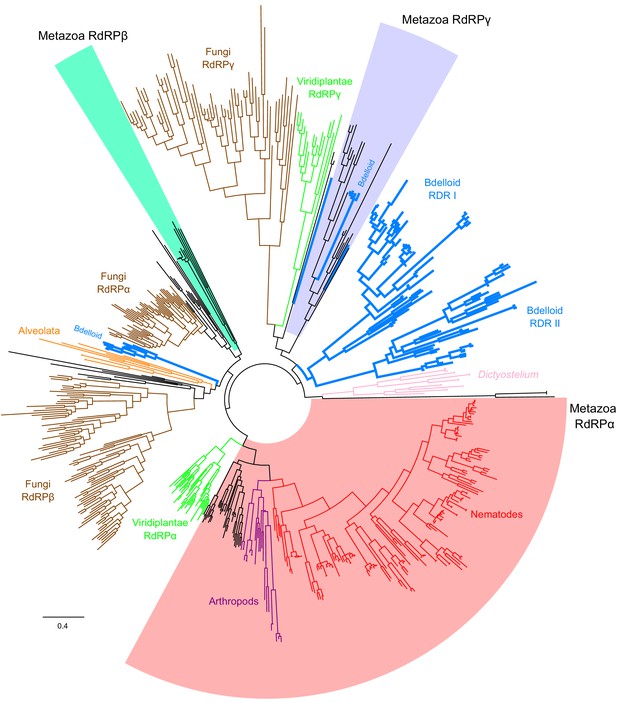
Extended RdRP phylogeny.
The core alignment and clade annotations are from Pinzón et al., 2019 with bdelloid copies from three species A. steineri, R. sordida, and R. sp. ‘Silwood-1’ 10x haploid assemblies inserted using HMMER ‘hmmalign’. Bdelloid copies are indicated on the tree with thicker branches coloured blue. The metazoan clades of RdRPα, β, and γ are shaded with red, green, and blue, respectively. Note that the large diversity of nematode RdRPs (red branches) is mostly due to extensive taxon sampling from within the nematodes (58 species); in contrast, the bdelloid copies are sampled from three species. Scale bar indicates 0.4 amino acid substitutions per site.
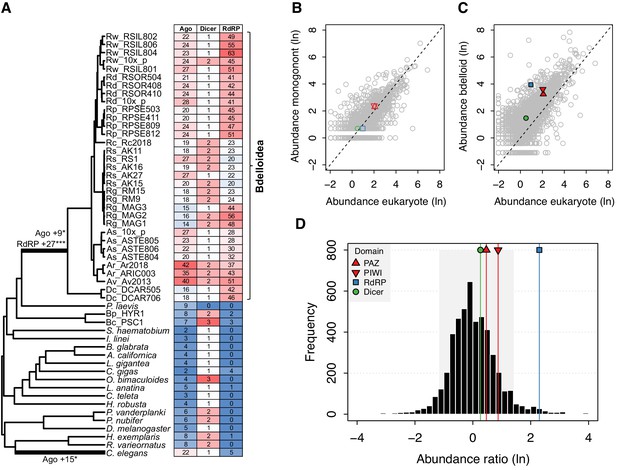
Evidence for significant expansion of Argonaute and RdRP gene families in bdelloids.
(A) Evidence for significant expansion of Argonaute (+9 copies; p<0.05) and RdRP (+27 copies; p<0.001) on the stem branch leading to the bdelloid clade. A significant expansion of Argonaute genes (+15 copies; p<0.05) is also found on the branch leading to the nematode C. elegans, corresponding to the nematode WAGO genes. Phylogeny is based on the concatenated alignment of a subset of core eukaryotic (BUSCO) proteins for this set of 59 taxa (Figure 2—source datas 3 and 4). Numbers of Ago, Dicer, and RdRP proteins (based on HMM hits of key domains to predicted proteomes) are shown in the table, shaded by their relative abundance. The relatively lower numbers seen for R. socialis and some R. magnacalcarata individuals is probably an artefact of assembly ‘collapse’ due to low heterozygosity in these genomes. (B) Comparative protein-domain abundance plot. Each point represents a Pfam domain ID, with (loge) average abundance (i.e. count) in the reference eukaryote set shown on the X-axis and (loge) abundance in the monogonont B. plicatilis on the Y-axis. The positions of the PAZ and PIWI (key domains of Argonaute; red up/down triangles), RdRP (blue square), and Dicer (green circle) domains are highlighted. Dashed line indicates the 1-to-1 relationship. (C) Equivalent plot for bdelloids, where the Y-axis shows the (loge) average abundance for the A. steineri, R. sordida, and R. sp. ‘Silwood-1’ 10x haploid assemblies. Note that the average abundance for all Pfam entries is shifted above the 1-to-1 line due to the ancient genome duplication in all bdelloids, such that many genes are found in double copy (i.e. homoeologs) even in a ‘haploid’ representation. (D) Comparative protein-domain abundance plot for bdelloids versus eukaryotes (see Materials and methods). Entries to the right of the mean of the distribution are overrepresented in bdelloids with respect to eukaryotes. The shaded area represents the 5% and 95% quantiles of the distribution, and the scores for the PAZ, PIWI, Dicer, and RdRP domains are indicated (see legend).
-
Figure 8—source data 1
CAFE model fitting birth rate model to gene family evolution of RNAi pathways.
- https://cdn.elifesciences.org/articles/63194/elife-63194-fig8-data1-v3.docx
-
Figure 8—source data 2
Pfam count data.
- https://cdn.elifesciences.org/articles/63194/elife-63194-fig8-data2-v3.txt.zip
Tables
Assembly statistics for 1 monogonont and 30 bdelloid rotifer reference assemblies presented in this study.
Sample ID | Species name | SZ (Mb) | NN | N50 (kb) | L50 | AU (kb) | GC (%) | Gaps (kb) | Coverage (X) | Genome BUSCO score | CDS | Proteome BUSCO score | GenBank accession |
---|---|---|---|---|---|---|---|---|---|---|---|---|---|
Bc_PSC1 | Brachionus calyciflorus (Monogonont) | 116.7 | 14,869 | 18.5 | 1692 | 26.6 | 25.6 | 78 | 186 | C:96%[S:93%,D:3%],F:2% | 24,404 | C:98%[S:93%,D:5%],F:1% | GCA_905250105.1 |
Ar_ARIC003 | Adineta ricciae | 135.6 | 4302 | 283.8 | 129 | 388 | 35.5 | 65 | 89 | C:97%[S:58%,D:39%],F:2% | 49,015 | C:97%[S:52%,D:45%],F:1% | GCA_905250025.1 |
As_10x_p | Adineta steineri | 171.1 | 8257 | 200.1 | 163 | 394.5 | 29 | 206 | 198 | C:95%[S:67%,D:33%],F:2% | 50,321 | C:97%[S:58%,D:38%],F:2% | GCA_905250115.1 |
As_ASTE804 | Adineta steineri | 160.3 | 9359 | 158.1 | 265 | 214.9 | 29.1 | 152 | 62 | C:95%[S:74%,D:22%],F:2% | 47,222 | C:98%[S:74%,D:24%],F:2% | GCA_905250045.1 |
As_ASTE805 | Adineta steineri | 156.3 | 9008 | 169.6 | 245 | 226.4 | 29.2 | 129 | 65 | C:98%[S:77%,D:21%],F:1% | 43,986 | C:99%[S:72%,D:26%],F:1% | GCA_905250065.1 |
As_ASTE806 | Adineta steineri | 160.3 | 7597 | 168.2 | 257 | 222.5 | 29.2 | 145 | 82 | C:96%[S:72%,D:24%],F:2% | 45,930 | C:98%[S:74%,D:24%],F:2% | GCA_905250035.1 |
Dc_DCAR505 | Didymodactylos carnosus | 323.6 | 87,048 | 7.8 | 11,656 | 10.5 | 33.5 | 41 | 21 | C:86%[S:69%,D:17%],F:8% | 46,286 | C:88%[S:71%,D:18%],F:9% | GCA_905249995.1 |
Dc_DCAR706 | Didymodactylos carnosus | 368.8 | 78,356 | 12 | 7695 | 19.1 | 33.5 | 13 | 76 | C:95%[S:70%,D:25%],F:2% | 46,863 | C:95%[S:71%,D:25%],F:2% | GCA_905250885.1 |
Rd_10x_p | Rotaria sordida | 272.5 | 16,571 | 64.5 | 843 | 193.8 | 30.8 | 395 | 91 | C:94%[S:77%,D:19%],F:2% | 44,299 | C:95%[S:69%,D:26%],F:2% | GCA_905250005.1 |
Rd_RSOR408 | Rotaria sordida | 252.9 | 20,315 | 57.6 | 1246 | 75.5 | 30.4 | 291 | 39 | C:94%[S:76%,D:19%],F:3% | 40,501 | C:97%[S:73%,D:24%],F:2% | GCA_905250875.1 |
Rd_RSOR410 | Rotaria sordida | 252.6 | 19,518 | 60.9 | 1179 | 80 | 30.4 | 252 | 42 | C:95%[S:77%,D:18%],F:3% | 40,474 | C:98%[S:74%,D:24%],F:2% | GCA_905251635.1 |
Rd_RSOR504 | Rotaria sordida | 251.3 | 22,067 | 53.1 | 1338 | 69.8 | 30.4 | 369 | 39 | C:94%[S:78%,D:16%],F:3% | 41,085 | C:96%[S:73%,D:23%],F:3% | GCA_905252715.1 |
Rg_MAG1 | Rotaria magnacalcarata | 178.7 | 19,184 | 42 | 1077 | 62.4 | 32 | 402 | 58 | C:97%[S:81%,D:16%],F:1% | 40,318 | C:99%[S:76%,D:22%],F:1% | GCA_905261645.1 |
Rg_MAG2 | Rotaria magnacalcarata | 181.1 | 22,216 | 39.7 | 1141 | 61 | 32 | 433 | 63 | C:98%[S:81%,D:17%],F:1% | 40,289 | C:99%[S:74%,D:26%],F:0% | GCA_905273325.1 |
Rg_MAG3 | Rotaria magnacalcarata | 180.9 | 22,132 | 40.7 | 1142 | 60 | 32 | 508 | 60 | C:96%[S:80%,D:17%],F:1% | 40,740 | C:99%[S:77%,D:22%],F:0% | GCA_905319835.1 |
Rg_RM15 | Rotaria magnacalcarata | 174 | 18,391 | 46.5 | 966 | 67.3 | 32 | 430 | 55 | C:96%[S:80%,D:16%],F:2% | 38,283 | C:99%[S:77%,D:22%],F:1% | GCA_905321285.1 |
Rg_RM9 | Rotaria magnacalcarata | 173.8 | 19,520 | 44 | 999 | 64.7 | 31.9 | 594 | 51 | C:96%[S:80%,D:16%],F:1% | 38,404 | C:98%[S:76%,D:22%],F:1% | GCA_905321535.1 |
Rp_RPSE411 | Rotaria sp. ‘Silwood-2’ | 296.5 | 30,050 | 102.5 | 381 | 691.1 | 31 | 247 | 35 | C:93%,[S:72%,D:21%],F:4% | 48,378 | C:95%[S:72%,D:23%],F:4% | GCA_905329745.1 |
Rp_RPSE503 | Rotaria sp. ‘Silwood-2’ | 285.6 | 33,174 | 78.3 | 449 | 627.2 | 31.3 | 446 | 34 | C:91%,[S:75%,D:17%],F:5% | 48,269 | C:92%[S:72%,D:20%],F:7% | GCA_905330235.1 |
Rp_RPSE809 | Rotaria sp. ‘Silwood-2’ | 271.1 | 28,589 | 101.6 | 350 | 681.4 | 31 | 377 | 27 | C:93%,[S:76%,D:17%],F:4% | 47,010 | C:95%[S:74%,D:22%],F:4% | GCA_905330535.1 |
Rp_RPSE812 | Rotaria sp. ‘Silwood-2’ | 264.1 | 34,498 | 80.8 | 403 | 616.2 | 31.1 | 428 | 27 | C:89%,[S:74%,D:15%],F:8% | 47,040 | C:90%[S:73%,D:17%],F:8% | GCA_905330805.1 |
Rs_AK11 | Rotaria socialis | 149.2 | 6303 | 111.3 | 370 | 150.2 | 31.8 | 442 | 39 | C:97%,[S:80%,D:17%],F:1% | 34,844 | C:99%[S:75%,D:24%],F:1% | GCA_905331015.1 |
Rs_AK15 | Rotaria socialis | 147.4 | 5030 | 134.7 | 305 | 177.6 | 31.8 | 423 | 37 | C:96%,[S:79%,D:18%],F:1% | 34,140 | C:98%[S:76%,D:23%],F:1% | GCA_905331295.1 |
Rs_AK16 | Rotaria socialis | 147.4 | 4720 | 139.5 | 296 | 180.3 | 31.8 | 332 | 43 | C:97%,[S:80%,D:18%],F:0% | 33,717 | C:99%[S:76%,D:23%],F:1% | GCA_905331475.1 |
Rs_AK27 | Rotaria socialis | 149.9 | 5952 | 123.7 | 343 | 159.8 | 31.8 | 458 | 36 | C:97%,[S:80%,D:17%],F:0% | 34,369 | C:99%[S:75%,D:24%],F:1% | GCA_905331485.1 |
Rs_RS1 | Rotaria socialis | 151.1 | 6254 | 124.9 | 334 | 166.2 | 31.8 | 490 | 40 | C:97%,[S:80%,D:17%],F:0% | 33,937 | C:99%[S:77%,D:22%],F:1% | GCA_905331495.1 |
Rw_10x_p | Rotaria sp. ‘Silwood-1’ | 310.4 | 16,995 | 211.8 | 211 | 126.2 | 31.1 | 534 | 53 | C:95%,[S:76%,D:20%],F:1% | 44,241 | C:97%[S:73%,D:24%],F:1% | GCA_905250055.1 |
Rw_RSIL801 | Rotaria sp. ‘Silwood-1’ | 268.4 | 28,548 | 136.5 | 288 | 687.3 | 30.8 | 472 | 45 | C:94%,[S:77%,D:17%],F:4% | 41,574 | C:95%[S:75%,D:21%],F:5% | GCA_905331515.1 |
Rw_RSIL802 | Rotaria sp. ‘Silwood-1’ | 249.9 | 21,286 | 153.4 | 238 | 702.3 | 30.7 | 451 | 42 | C:92%,[S:76%,D:16%],F:4% | 39,577 | C:94%[S:76%,D:18%],F:4% | GCA_905331505.1 |
Rw_RSIL804 | Rotaria sp. ‘Silwood-1’ | 247.6 | 25,643 | 118.3 | 287 | 660.4 | 30.8 | 667 | 34 | C:94%,[S:78%,D:16%],F:3% | 41,139 | C:96%[S:78%,D:19%],F:3% | GCA_905331525.1 |
Rw_RSIL806 | Rotaria sp. ‘Silwood-1’ | 294.1 | 29,968 | 132.4 | 333 | 681.9 | 30.8 | 500 | 31 | C:95%,[S:79%,D:16%],F:2% | 48,259 | C:97%[S:78%,D:19%],F:2% | GCA_905331535.1 |
-
Sequence statistics codes: SZ, total sequence length (Mb); NN, number of sequences; N50, N50 scaffold length (kb); L50, N50 index; AU, expected scaffold size (area under ‘Nx’ curve, kb). BUSCO score based on eukaryote set (n = 303); BUSCO codes: C, complete; S, complete and single copy; D, complete and duplicated; F, fragmented.
-
Table 1—source data 1
Sample information and data counts.
- https://cdn.elifesciences.org/articles/63194/elife-63194-table1-data1-v3.xlsx
-
Table 1—source data 2
Assembly statistics for 30 maximum-haplotig assemblies.
- https://cdn.elifesciences.org/articles/63194/elife-63194-table1-data2-v3.xlsx