MicroRNAs mediate precise control of spinal interneuron populations to exert delicate sensory-to-motor outputs
Figures
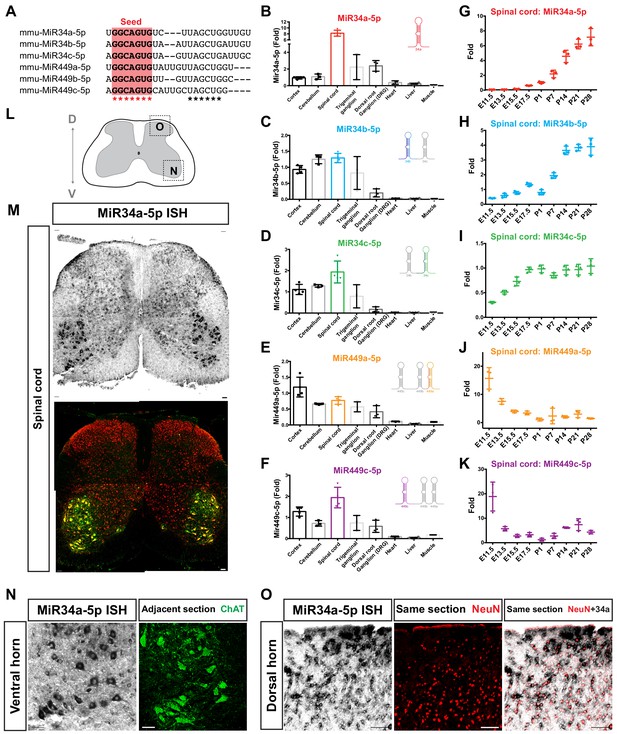
MiR34/449 miRNAs are expressed in the CNS and show dynamic expression profiles in the developing spinal cord.
(A) Sequence alignment of mouse MiR34/449 miRNAs with asterisks indicating identical or conserved nucleotides. The seed sequences are highlighted in red. (B–F) Expression levels of MiR34/449 (MiR34a-5p, MiR34b-5p, MiR34c-5p, MiR449a-5p, and MiR449c-5p), as measured by RT-qPCR in multiple tissues of adult wild-type (WT) mice at postnatal day P50. The MiR34/449 miRNAs were first normalized against ubiquitously expressed MiR16 in the corresponding samples (delta Ct). The relative level of each miRNA was then normalized with that of cortex (delta-delta Ct) and is represented as fold-change (data represent mean ± SD from N ≥ 3 independent biological samples). (G–K) Expression levels of MiR34/449 miRNAs, as determined by RT-qPCR at various developmental time-points in the spinal cord. The relative level of each miRNA was first normalized against the ubiquitously expressed MiR16 (delta Ct), and then further normalized against that at the P1 time-point (delta-delta Ct) and is represented as fold-change (data represent mean ± SD from N = 3 independent biological samples). Note that MiR34/449 miRNAs show dynamic expression profiles during spinal cord development, with both MiR34a-5p and MiR34b/c-5p showing increasing levels, whereas MiR449a-5p and MiR449c-5p show decreasing levels. (L) Schematic illustration of a transverse spinal section across the dorso-ventral axis. (M) In situ hybridization (ISH) of MiR34a-5p with immunostaining for NeuN/ChAT in adjacent sections of the lumbar spinal cord at P20. (N and O) High-magnification images of the ventral (N) and dorsal (O) spinal cord, showing expression of MiR34a-5p in ventral MNs and dorsal INs. Scale bars represent 100 μm in (M) and 50 μm in (N) and (O).
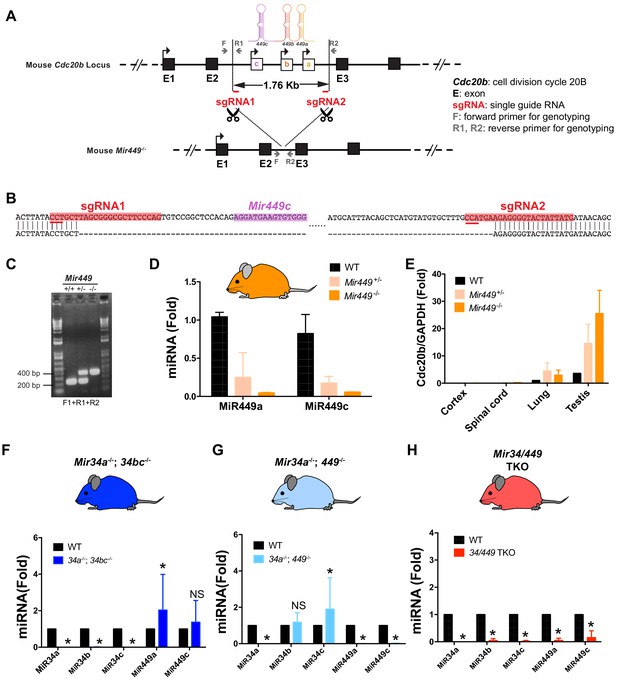
Generation and genotyping analyses of Mir449 KO mice by a CRISPR-Cas9 approach.
(A) Illustration of the sgRNA target sites for deleting the Mir449 cluster (Mir449a/b/c) embedded in the host gene Cdc20b. Around 1.76 Kb of the DNA sequence was removed by the CRISPR-Cas9-mediated method, and genotyping primers designed to distinguish WT and KO alleles are shown in the diagram. (B) After CRISPR-Cas9-mediated Mir449 deletion, the DNA sequence was verified as shown. The two sgRNA sequences are highlighted by red boxes, and the PAM sites are indicated by red bars. (C) Genotyping analyses of DNA isolated from mouse tails. (D) Expression of MiR449a and MiR449c was undetectable in Mir449-/- mice, as determined by RT-qPCR of spinal cord tissues from littermate-controlled WT, Mir449+/-, and Mir449-/- mice at E10.5 (WT and Mir449-/-, n = 2; Mir449+/-, n = 3). Relative levels of each miRNA are normalized against the value for WT and are represented as fold-change. (E) The expression of Mir449 cluster and its host gene Cdc20b in various tissues. Relative levels are normalized to values for lung tissue and are represented as fold-change (WT tissues, n = 1; Mir449+/- tissues, n = 2, Mir449-/- tissues, n = 3). Data are presented as mean ± SD. (F–H) Expression levels of MiR34/449 miRNAs (MiR34a-5p, MiR34b-5p, MiR34c-5p, MiR449a-5p, and MiR449c-5p) in spinal cord of postnatal day P14 WT and indicated KO mice, as measured by RT-qPCR. Relative levels of each miRNA are normalized with the respective value for WT and are represented as fold-change (n = 5). Data are presented as mean ± SD. Student’s t test. * denotes p<0.05; ‘NS’ denotes ‘not significant’.
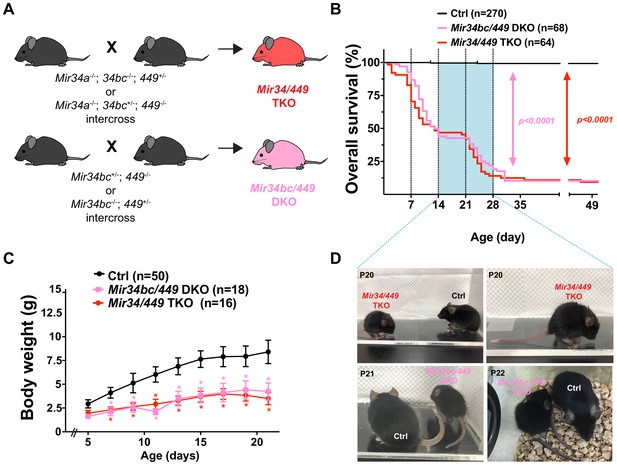
Mir34bc/449 DKO and Mir34/449 TKO mice exhibit marked postnatal lethality and survivors present profound neurological disorders.
(A) Experimental strategy to generate the Mir34/449 TKO or Mir34bc/449 DKO mice. (B) Survival rates of Mir34bc/449 DKO and Mir34/449 TKO mice compared to littermates (Ctrl; all littermates of various genotypes have been pooled), and compared by log-rank test. Both Mir34bc/449 DKO and Mir34/449 TKO mice show similar mortality levels in the first 4 weeks. (C) Mir34bc/449 DKO and Mir34/449 TKO mice display reduced body weight compared to all Ctrl mice. Data are presented as mean ± SD. Two-way ANOVA with Tukey’s multiple comparisons test (for each day); * denotes p<0.05. (D) Side and top views of Mir34bc/449 DKO or Mir34/449 TKO mice exhibiting abnormal body posture compared with the Ctrl, such as back-arching, curled body, hind tiptoe walking, and stimulus-induced hypersensitivity (see videos for details).
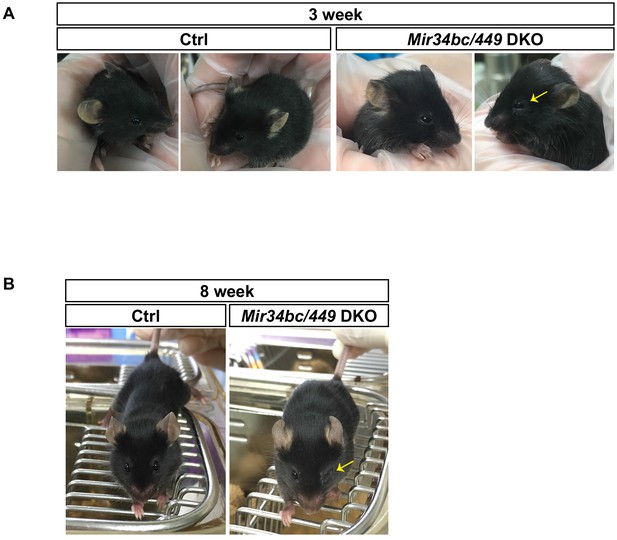
Blepharoptosis phenotype of MiR34/449-deficient mice.
Representative photos illustrating the blepharoptosis (ptosis; drooping of the upper eyelids) phenotype of the Mir34bc/449 DKO mice at 3 weeks old (A) and 8 weeks old (B). The Mir34bc/449 DKO and Mir34/449 TKO mice typically developed this phenotype for one eye only (occurrence ~8.8% for observed mutant mice), and only a very small proportion of the mutant mice exhibited this defect in both eyes. Arrows point to the affected side.
Peculiar walking pattern in the MiR34/449 mutant mice.
Jumpy and rushed movement in the MiR34/449 mutant mice.
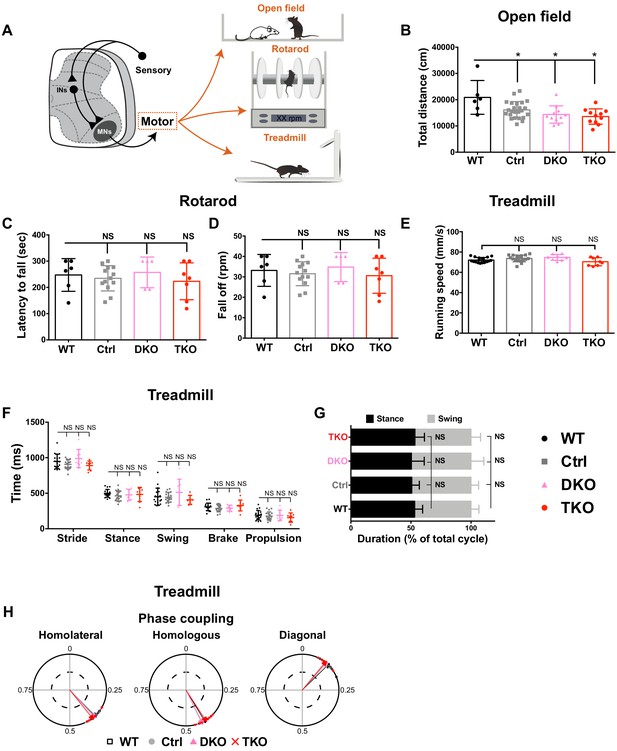
MiR34/449-deficient mice display overt jumpy pattern with no obvious deficit in basic motor function.
(A) Schematic illustration of the behavioral tests conducted to assess motor function. (B) Quantification of locomotor activity in an open-field arena (assessed according to total distance traveled) for WT, Ctrl and indicated Mir34/449 KO mice (WT: n = 6, Ctrl: n = 24, Mir34bc/449 DKO: n = 11, Mir34/449 TKO: n = 11). (C and D) Locomotor coordination on an accelerating rotarod, displayed as latency to fall (C) and the rotation speed at which mice fell off (D). There were no differences between MiR34/449 mutants and WT/Ctrl mice (WT: n = 6, Ctrl: n = 13, Mir34bc/449 DKO: n = 5, Mir34/449 TKO: n = 7). (E–H) Limb coordination and gait analyses were assayed by treadmill walking. The MiR34/449 mutants and WT/Ctrl mice have comparable running speeds (E), temporal parameters of the hindlimb (stride, stance, swing, brake, propulsion time; F), and ratios of swing and stance phases during the gait cycle (G). Limb coordination (homolateral, homologous, and diagonal phase coupling) is displayed as circular plots (H). Each symbol represents one trial from an individual mouse, and the arrow vectors represent the mean phases from all experiments for each group of genotyped mice. The length of the vector indicates the concentration of phase values around the mean (WT: n = 18, Ctrl: n = 18, Mir34bc/449 DKO: n = 8, Mir34/449 TKO: n = 8). No significant differences were observed among MiR34/449 mutant mice and Ctrl/WT mice. Data are presented as mean ± SD; one-way ANOVA with Tukey’s multiple comparisons test; * denotes p<0.05; ‘NS’ denotes ‘not significant’.
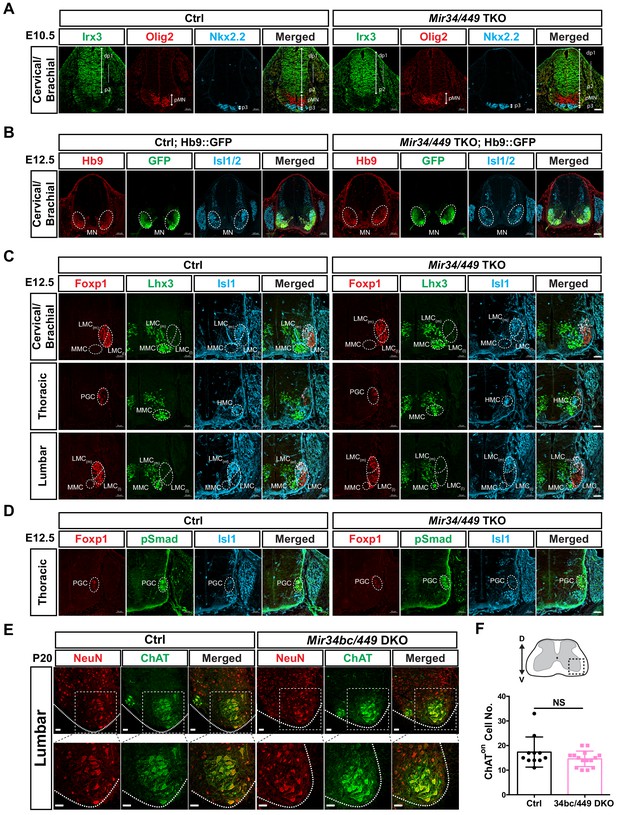
MN development may not be grossly affected in the spinal cord of Mir34/449 KO mice.
(A) Immunostaining for Irx3, Olig2, and Nkx2.2 reveals no obvious progenitor domain change in the cervical/brachial spinal cord section at E10.5 for the Mir34/449 TKO line compared to Ctrl embryos (pMN, motor neuron progenitor; p0 ~3, ventral progenitor domains; pd1 ~6, dorsal progenitor domains). (B) MiR34/449 mutant mice were crossed with the MN reporter Hb9::GFP and then immunostained for Hb9 and Isl1/2. The distribution of MN domain is normal in cervical/brachial spinal cord sections at E12.5 for the Mir34/449 TKO line compared to Ctrl. Dashed lines demarcate the MN region in the ventral spinal cord. (C and D) Immunostaining reveals comparable distributions and cell numbers of MMC (medial motor column; Lhx3+), LMC(m) (medial division of lateral motor column; FoxP1on, Isl1on), LMC(l) (lateral division of lateral motor column; FoxP1on, Isl1off), HMC (hypaxial motor column; Lhx3off, Isl1on), and PGC (preganglionic motor column; FoxP1on, pSmadon) MNs in the indicated segments of spinal cord sections from E12.5 embryos. (E) Immunostaining for pan-neuronal marker NeuN and mature MN marker ChAT in lumbar hemi-ventral horn sections of Ctrl and Mir34bc/449 DKO mice at P20. (F) Quantification of ChATon cell numbers from (E). Data are presented as mean ± SD. Student t test. ‘NS’ denotes ‘not significant’. Scale bars are 50 μm in (A, C, D, E) and 100 μm in (B).
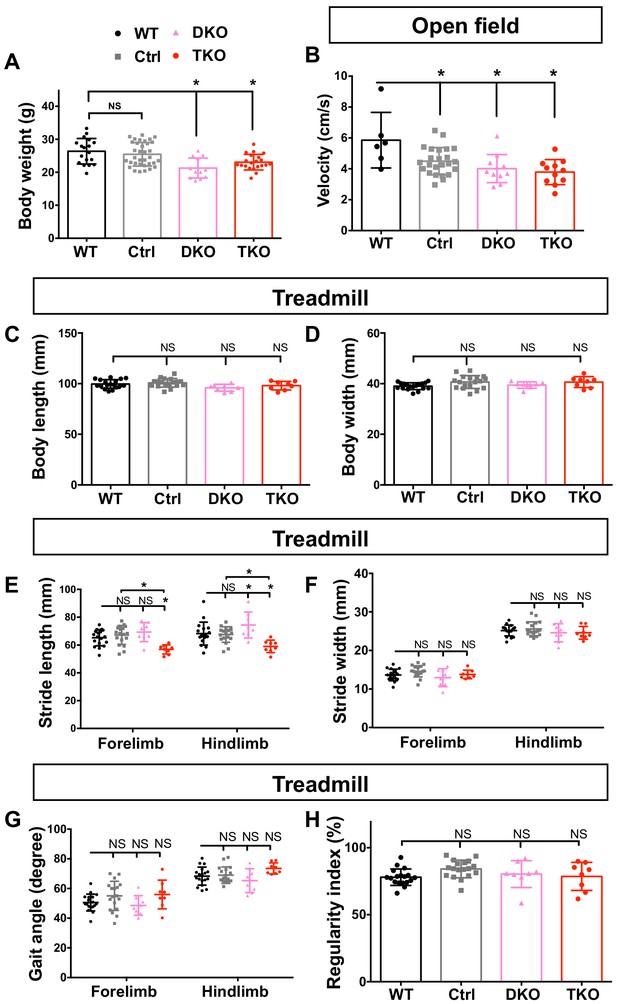
MiR34/449-deficient mice display subtle change of hindlimb moving pattern.
(A) Body weight of mice before being subjected to behavioral assays. MiR34/449 mutant mice have moderately lower body weights compared to WT/Ctrl. (B) Locomotor activity in an open-field arena (assessed by average velocity) for WT, Ctrl, and the indicated Mir34/449 KO mice. Ctrl and MiR34/449 mutant mice exhibit reduced velocity in the arena relative to WT, which may represent lower locomotor activity or reflect reduced body weight. (C–H) Parameters measured during treadmill walking. The MiR34/449 mutant and WT/Ctrl mice exhibited comparable body length (C), body width (D), stride width (F), and gait angles for both forelimb and hindlimb (G). The Mir34/449 TKO mutant mice showed subtle differences in stride length (E) relative to WT/Ctrl. The regularity index (H) is represented as percentage values and was used for gait evaluation, with all four groups exhibiting normal patterns. Data are presented as mean ± SD. One-way ANOVA with Tukey’s multiple comparisons test. * denotes p<0.05; ‘NS’ denotes ‘not significant’.
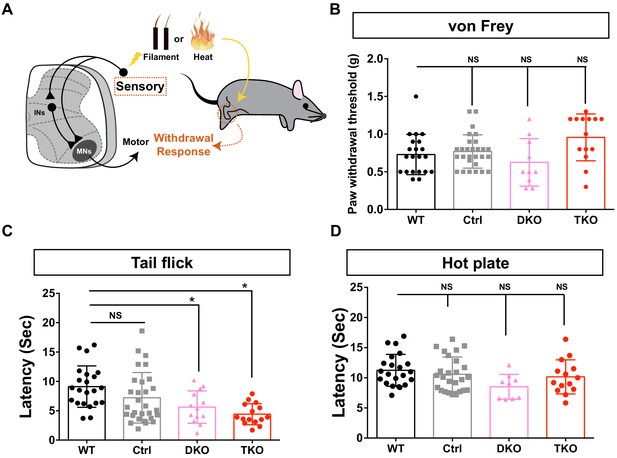
Mir34/449 KO mice exhibit hypersensitivity in response to thermal stimulation.
(A) Schematic illustration of the sensory assays. (B) A von Frey test revealed comparable thresholds for inducing a mechanical pain response among 4-month-old WT, Ctrl, and MiR34/449 mutant mice (WT: n = 21, Ctrl: n = 28, Mir34bc/449 DKO: n = 10, Mir34/449 TKO: n = 14). Data are represented as mean threshold (grams of force) ± SD. (C) MiR34/449 mutant mice exhibit greater heat pain sensitivity compared to WT in a tail-flick test. Ctrl mice also showed a reduced latency in response to the heat stimulus relative to WT, but it is not statistically significant (WT: n = 22, Ctrl: n = 29, Mir34bc/449 DKO: n = 13, Mir34/449 TKO: n = 15). (D) Heat thresholds to induce a thermal pain response (hot-plate set at 55°C) are presented as the latency to licking/flicking the hind paw or jumping behavior. The MiR34/449 mutant mice display a subtle but not statistically significant difference in heat sensitivity compared to WT/Ctrl (WT: n = 22, Ctrl: n = 26, Mir34bc/449 DKO: n = 9, Mir34/449 TKO: n = 14). Data are presented as mean ± SD; one-way ANOVA with Tukey’s multiple comparisons test; * denotes p<0.05; ‘NS’ denotes ‘not significant’.
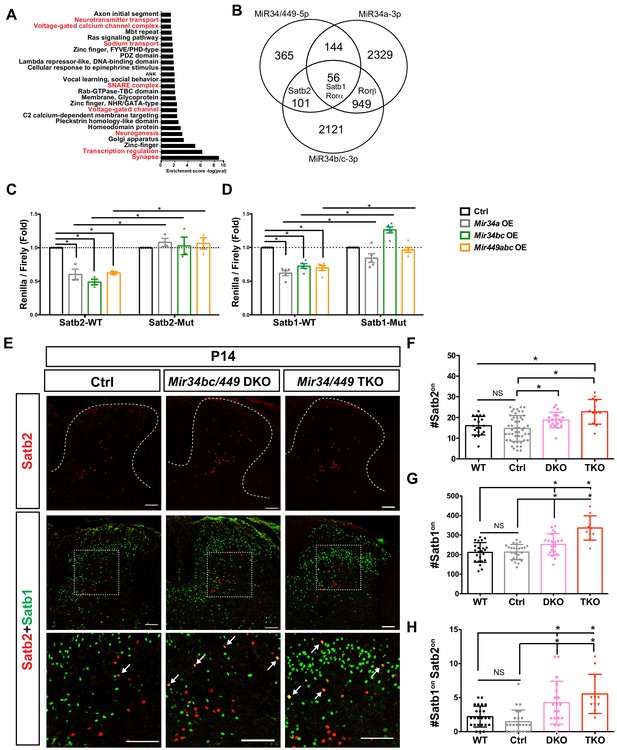
Satb1 and Satb2 are direct targets of MiR34/449 miRNAs.
(A) Strategy to identify potential MiR34/449 targets in the spinal cord. Predicted targets of MiR34/449 miRNAs by TargetScan were subjected to gene ontology analysis. Potential pathways related to the neural behavior phenotype are highlight with red color (B) Predicted targets of MiR34/449 miRNAs were filtered based on having both miRNA (MiR34/449-5p) and miRNA* (MiR34b/c-3p and MiR34a-3p) targeting sites, and spinal INs were selected for further study. (C–D) Co-expression of the luciferase construct with MiR34/449 plasmids in HEK293T cells silences the reporter carrying the intact MiR34/449 target sites, whereas Mir34/449 plasmids fail to silence Satb2-Mut (C) and Satb1-Mut (D) luciferase constructs. Transfection of the backbone plasmid served as the control (Ctrl). OE, overexpression. N = 3 in (C) and five in (D). (E) Immunostainings for Satb2 (red) and Satb1 (green) in the dorsal and intermediate spinal cord of cervical/brachial sections at P14 revealed significantly increased numbers of Satb2on and Satb1on cells. Lower panels are higher magnifications of the boxed areas in the respective middle panels. Double-positive cells are indicated with arrows. (F–H) Quantification of Satb2on (F), Satb1on (G), and Satb2on;Satb1on double-positive INs (H) from (E). Each dot represents the quantification from one hemi-section. Data are presented as mean ± SD. One-way ANOVA with Tukey’s multiple comparisons test. * denotes p<0.05; ‘NS’ denotes ‘not significant’. Scale bars represent 100 μm.
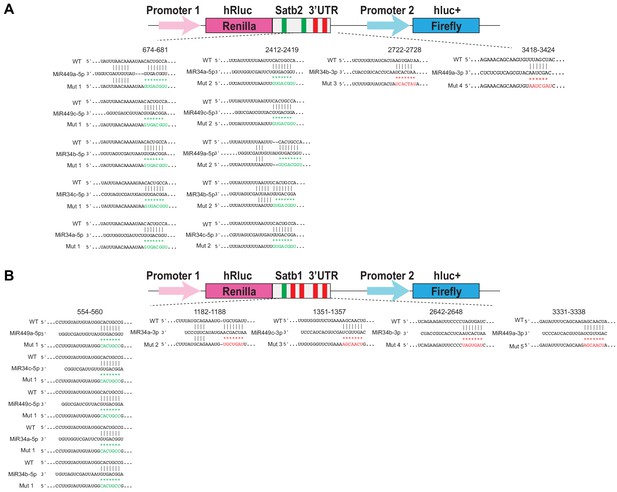
Luciferase reporter and mutant construction.
(A–B) Schematic illustration of the luciferase reporters harboring the putative MiR34/449 targeting sequence of the Satb2 (A) and Satb1 (B) 3ʹ UTRs. The reporters were constructed with either a control 3ʹ UTR (WT) or a 3ʹ UTR sequence in which all potential target sites of MiR34/449 miRNAs were mutated (Mut; target sites for MiR34/449-5p and MiR34/449-3p are shown in green and red, respectively).
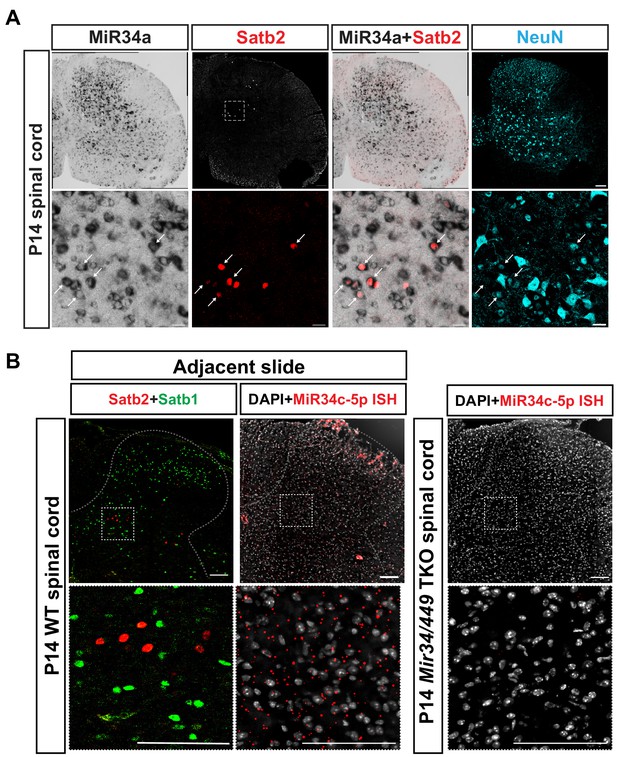
Expression of MiR34a-5p and MiR34c-5p in the postnatal spinal cord.
(A) In situ hybridization of MiR34a-5p together with Satb2 and NeuN immunostaining of cervical/brachial spinal cord sections at P14. Boxed areas depicted in the upper panels are shown with higher magnification in the respective lower panels. Arrows indicate expression of MiR34a-5p in Satb2on INs. Scale bars are 100 μm and 20 μm in the upper and lower panels, respectively. (B) Detection of MiR34c-5p (probe sequence could not differentiate MiR34b/c) with miRNAscope technology revealed its expression in the spinal cord at P14 (central panel). Immunostaining for Satb1 and Satb2 on the adjacent slide is shown in the left panel. Note that the Mir34/449 TKO section showed undetectable MiR34b/c signal (right panel), supporting the specificity of the probe used in this study. Boxed areas depicted in the upper panels are shown with higher magnification in the respective lower panels. Scale bars are 100 μm.
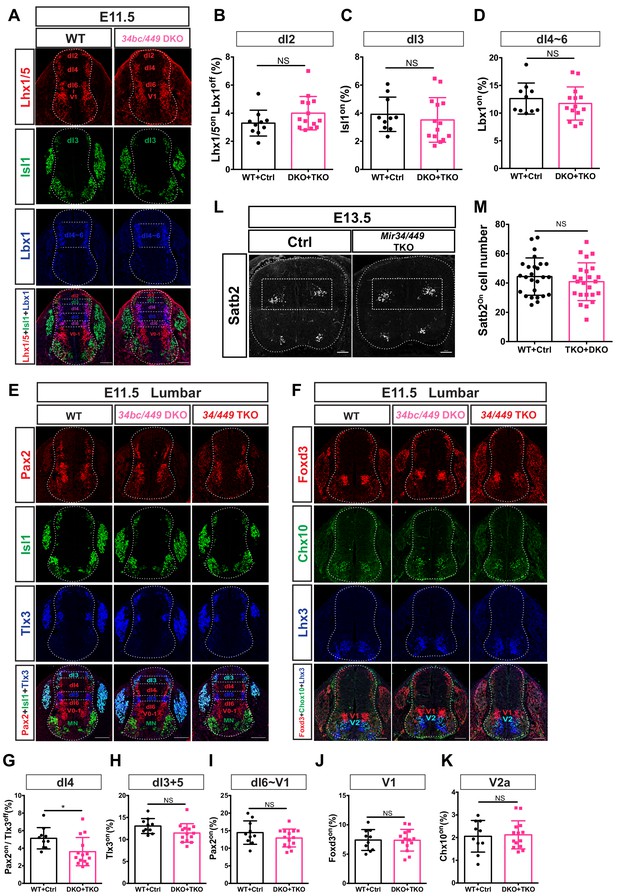
Spinal IN development may not be grossly affected in the MiR34/449 mutant mice.
(A) Immunostainings for Lhx1/5, Isl1, and Lbx1 revealed no obvious dorsal interneuron (dI) domain change in Mir34bc/449 DKO embryos compared to WT for lumbar spinal cord sections at E11.5. Lhx1/5 (red) labels dI2, dI4, and dI6~V1; Isl1 (green) labels dI3 and MNs; Lbx1 (blue) labels dI4 ~6. (B–D) Quantification of cell numbers from the indicated dI domains in the developing spinal cord, normalized against total cell number (DAPI+) and represented as percentages. (E) Immunostaining for Pax2, Isl1, and Tlx3 to reveal dorsal interneuron (dI) domains in lumbar spinal cord sections at E11.5. Pax2 (red) labels dI4 and dI6~V1; Isl1 (green) labels dI3 and MNs; Tlx3 (blue) labels dI3 and dI5. (F) Immunostaining for Foxd3, Chx10, and Lhx3 to reveal ventral (V) interneuron domains in lumbar spinal cord sections at E11.5. Foxd3 (red) labels dI2 and V1; Chx10 (green) labels V2a; Lhx3 (blue) labels V2 and MN. (G–I) Quantification of cell numbers from the indicated dI domains in the developing spinal cord in (E), normalized against the total cell number (DAPI+) and represented as a percentage. Each dot represents quantification from one hemi-section. The MiR34/449 mutant mice showed comparable dI3+5 (H) and dI6~V1 (I) domain cell counts to that of WT/Ctrl, whereas cell counts of the dI4 (G) domain in mutant mice are reduced relative to WT/Ctrl. (J–K) Quantification of cell numbers from the indicated ventral domains in the developing spinal cord in (F), normalized against the total cell number (DAPI+) and represented as a percentage. The MiR34/449 mutant mice showed comparable V1 (J) and V2a (K) domain cell counts relative to WT/Ctrl. (L) Immunostaining of Satb2 reveals no obvious difference in number of Satb2on INs in the cervical/brachial spinal cord section at E13.5. (M) Quantification of Satb2on INs from (L). Data are presented as mean ± SD. Student t test. * denotes p<0.05; ‘NS’ denotes ‘not significant’. Each dot represents the quantification from one hemi-section (B–D, G–K). Scale bars are 100 μm.
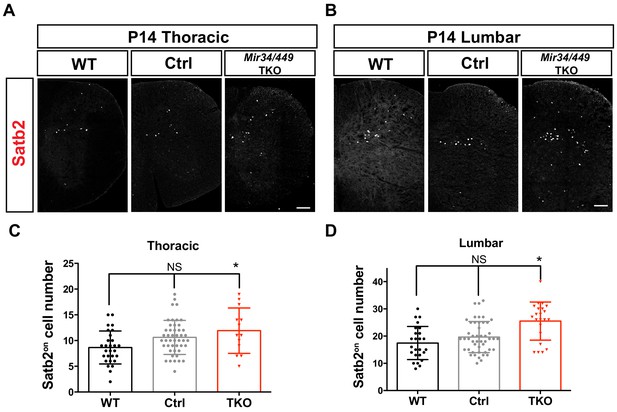
Numbers of Satb2on INs are increased in both the thoracic and lumbar regions of the spinal cord.
(A–B) Immunostaining of Satb2 reveals increased numbers of Satb2on INs in MiR34/449 mutant mice compared to Ctrl mice in thoracic (A) and lumbar (B) spinal cord sections at P14. (C–D) Quantification of Satb2on interneurons from (A) and (B). Data are presented as mean ± SD. Each dot represents the quantification from one hemi-section. One-way ANOVA with Tukey’s multiple comparisons test. * denotes p<0.05; ‘NS’ denotes ‘not significant’.
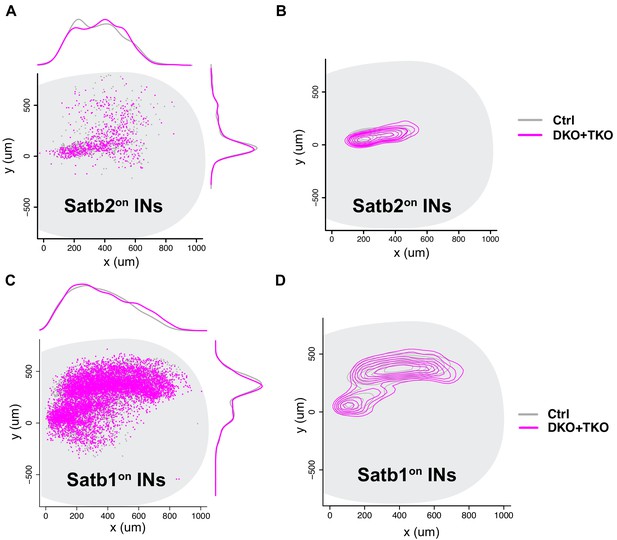
Spatial distribution of Satb2on INs.
(A and C) Comparison of the spatial distribution of individual Satb2on (A) and Satb1on (C) INs in the cervical/brachial spinal cord of indicated mice (Ctrl, gray; Mir34bc/449 DKO and Mir34/449 TKO, magenta). Frequency distributions along the medio-lateral (top) and dorso-ventral (right) axes represent non-linear regressions. (B and D) Contour density plots of Satb2on (B) and Satb1on (D) INs reveal that Satb2on INs (B) are mainly located in the medial spinal cord of both MiR34/449 mutant and Ctrl spinal cord. Satb1on INs (D) presented two major localizations, that is, in the intermediate and dorsal spinal cord, in both MiR34/449 mutant and Ctrl mice. Contour lines represent density at the 30th–90th percentiles. Data from more that 25 sections of at least nine independent spinal cords.
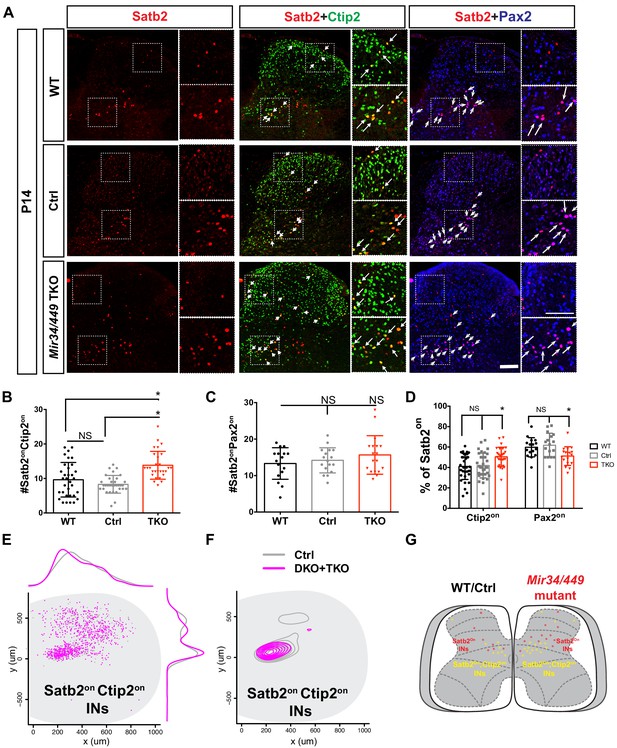
MiR34/449 tunes optimal Satb2 expression in the Ctip2 IN subpopulation.
(A) Immunostaining for Satb2, Ctip2, and Pax2 in dorsal and intermediate spinal cord at P14. Note that Satb2on INs display two molecular identities, i.e., the Ctip2on or Pax2on subpopulations. Comparison with WT/Ctrl reveals an increase in the cell number of Satb2on;Ctip2on INs in the intermediate spinal cord of cervical/brachial sections upon MiR34/449 deletion. Boxed areas indicate higher magnification regions from the intermediate and dorsal regions of each image. Arrows highlight the presence of dual-labeled Satb2on;Ctip2on cells (yellow) or dual-labeled Satb2on;Pax2on cells (magenta) among the Satb2on population. (B–C) Quantifications of cell number from (A). (D) Quantifications of the Satb2on;Ctip2on and Satb2on;Pax2on subpopulations were normalized against total Satb2on cell numbers and are shown as a percentage. (E) Comparison of the spatial distribution of individual Satb2on;Ctip2on INs in the cervical/brachial spinal cord of indicated mice (Ctrl, grey; Mir34bc/449 DKO and Mir34/449 TKO, magenta). Frequency distributions along the medio-lateral (top) and dorso-ventral (right) axes represent non-linear regressions. (F) A contour density plot of Satb2on;Ctip2on INs indicates that Satb2on;Ctip2on INs are mainly located in the medial spinal cord of both MiR34/449 mutant and Ctrl mice. Contour lines represent density at the 30th–90th percentiles. Data were analyzed from more than 25 sections of at least five independent spinal cords in (E) and (F). (G) Schematic illustrating the increased number of Satb2on;Ctip2on INs in the MiR34/449 mutant spinal cord. Data are presented as mean ± SD. One-way ANOVA with Tukey’s multiple comparisons test. * denotes p<0.05; ‘NS’ denotes ‘not significant’. Scale bars represent 100 μm.
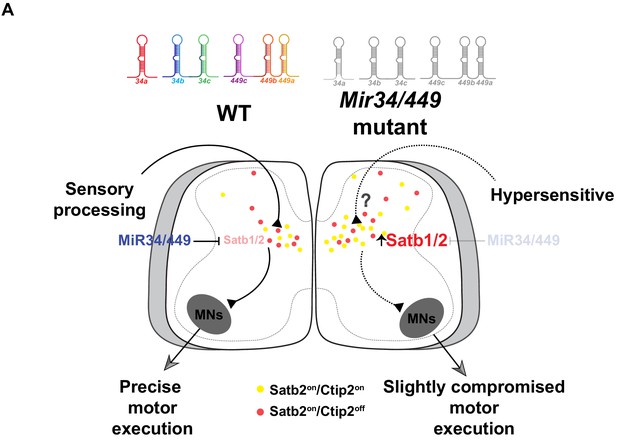
MiR34/449 miRNAs mediate control of proper interneuron populations to exert precise core sensory-to-motor spinal outputs.
Summary diagram of the increased numbers of Satb1/2on INs, and specifically the Satb2 on;Ctip2on subpopulation, in MiR34/449 mutant mice and the possible changes in circuitry that result from MiR34/449 depletion in the spinal cord.
Tables
Reagent type (species) or resource | Designation | Source or reference | Identifiers | Additional information |
---|---|---|---|---|
Antibody | Rabbit monoclonal anti-Satb2 | Abcam | Cat# ab92446, RRID:AB_10563678 | IHC (1:500) |
Antibody | Guinea pig polyclonal anti-Satb2 | Synaptic Systems | Cat# 327004, RRID:AB_2620070 | IHC (1:500) |
Antibody | Goat polyclonal anti-ChAT | Millipore/Sigma | Cat# AB144P, RRID:AB_2079751 | IHC (1:100) |
Antibody | Sheep anti-GFP | Bio-Rad | Cat# 4745–1051, RRID:AB_619712 | IHC (1:2000) |
Antibody | Mouse monoclonal anti-NeuN | Millipore | Cat# MAB377, RRID:AB_2298772 | IHC (1:500) |
Antibody | Rabbit polyclonal anti-Irx3 | T. Jessell (Columbia, New York) | N/A | IHC (1:5000) |
Antibody | Rabbit polyclonal anti-pSmad1/5/8 | Merck | Cat# AB3848, RRID:AB_177439 | IHC (1:500) |
Antibody | Guinea pig anti-Olig2 | T. Jessell (Columbia, New York) | N/A | IHC (1:10000) |
Antibody | Rabbit polyclonal anti-Olig2 | Millipore | Cat# AB9610; RRID:AB_570666 | IHC (1:5000) |
Antibody | Mouse monoclonal anti-Nkx2.2 | T. Jessell (Columbia, New York) | N/A | IHC (1:10000) |
Antibody | Guinea pig anti-Hb9 | H. Wichterle (Columbia, New York) | N/A | IHC (1:1000) |
Antibody | Rabbit polyclonal anti-Lhx3 | Abcam | Cat# ab14555, RRID:AB_301332 | IHC (1:2000) |
Antibody | Rabbit polyclonal anti-Foxp1 | Abcam | Cat# ab16645, RRID:AB_732428 | IHC (1:10000) |
Antibody | Guinea pig anti-Foxp1 | T. Jessell (Columbia, New York) | N/A | IHC (1:20000) |
Antibody | Mouse monoclonal anti-Isl1/2 | DSHB | Cat# 39.4D5, RRID:AB_2314683 | IHC (1:1000) |
Antibody | Goat polyclonal anti-Isl1 | Neuromics | Cat# GT15051, RRID:AB_2126323 | IHC (1:100) |
Antibody | Mouse monoclonal anti-Isl1 | DSHB | Cat# 39.3F7, RRID:AB_1157901 | IHC (1:100) |
Antibody | Mouse monoclonal anti-Lhx1/5 | DSHB | Cat# 4F2, RRID:AB_531784 | IHC (1:1000) |
Antibody | Guinea pig anti-Lbx1 | T. Müller (MDC, Berlin) | N/A | IHC (1:10000) |
Antibody | Guinea pig anti-Tlx3 | T. Müller (MDC, Berlin) | N/A | IHC (1:10000) |
Antibody | Guinea pig anti-Foxd3 | T. Müller (MDC, Berlin) | N/A | IHC (1:5000) |
Antibody | Rabbit polyclonal anti-Pax2 | BioLegend/Covance | Cat# 901002, RRID:AB_2734656 | IHC (1:1000) |
Antibody | Rabbit polyclonal anti-Pax2 | Thermo Fisher Scientific | Cat# 71–6000, RRID:AB_2533990 | IHC (1:1000) |
Antibody | Sheep polyclonal anti-Chx10 | Exalpha Biologicals | Cat# X1179P RRID:AB_2567565 | IHC (1:100) |
Antibody | Rat monoclonal anti-Ctip2 | Abcam | Cat# ab18465, RRID:AB_2064130 | IHC (1:500) |
Antibody | Goat anti-Pax2 | R and D Systems | Cat# AF3364, RRID:AB_10889828 | IHC (1:500) |
Antibody | Rabbit polyclonal anti-Satb1 | Novus | Cat# NBP2-15108 | IHC (1:1000) |
Cell line (human) | HEK293T | Sigma-Aldrich | Cat# 12022001 | |
Genetic reagent (M. musculus) | Hb9::GFP | The Jackson Laboratory | Cat# JAX: 005029, RRID:IMSR_JAX: 005029 | |
Genetic reagent (M. musculus) | Mir34afl/fl | The Jackson Laboratory | Cat# JAX:018545, RRID:IMSR_JAX:018545 | |
Genetic reagent (M. musculus) | Mir34bc-/- | The Jackson Laboratory | Cat# JAX:018546, RRID:IMSR_JAX:018546 | |
Genetic reagent (M. musculus) | Mir34a-/- | This paper | N/A | |
Genetic reagent (M. musculus) | Mir449-/- | This paper | N/A | |
Genetic reagent (M. musculus) | Mir34a-/-; Mir34bc-/- | This paper | N/A | |
Genetic reagent (M. musculus) | Mir34a-/-; Mir449-/- | This paper | N/A | |
Genetic reagent (M. musculus) | Mir34bc-/-; Mir449-/- | This paper | N/A | |
Genetic reagent (M. musculus) | Mir34a-/-; Mir34bc-/-; Mir449-/- | This paper | N/A | |
Genetic reagent (M. musculus) | E2a::Cre | The Jackson Laboratory | Cat# JAX:003724, RRID:IMSR_JAX:003724 | |
Recombinant DNA | psiCHECK-2 vector | Promega | Cat# C8021, RRID:Addgene_106979 | |
Recombinant DNA | psiCHECK-2-Satb2-3’UTR_WT | This paper | N/A | |
Recombinant DNA | psiCHECK-2-Satb2-3’UTR_Mut | This paper | N/A | |
Recombinant DNA | pENTR/D-TOPO-Mir34a | This paper | N/A | |
Recombinant DNA | pENTR/D-TOPO-Mir34bc | This paper | N/A | |
Recombinant DNA | pENTR/D-TOPO-Mir449abc | This paper | N/A | |
Recombinant DNA | p2Lox-Mir34a | This paper | N/A | |
Recombinant DNA | p2Lox-Mir34bc | This paper | N/A | |
Recombinant DNA | p2Lox-Mir449abc | This paper | N/A | |
Software, algorithm | Adobe Illustrator 2020 | Adobe | https://www.adobe.com | |
Software, algorithm | Adobe Photoshop 2020 | Adobe | https://www.adobe.com | |
Software, algorithm | Image J | NIH | https://imagej.net/ RRID:SCR_003070 | |
Software, algorithm | Imaris 9.5.1 | Bitplane | http://www.bitplane.com/imaris/imaris RRID:SCR_007370 | |
Software, algorithm | ZEN | Carl Zeiss | RRID:SCR_013672 | |
Software, algorithm | Prism 6 | GraphPad | https://www.graphpad.com RRID:SCR_005375 | |
Software, algorithm | TreadScan4.0 | CleverSystems | N/A | |
Software, algorithm | R and RStudio | The R foundation | https://www.r-project.org/ https://www.rstudio.com RRID:SCR_000432 |
Additional files
-
Supplementary file 1
Primers, genotypes of mouse littermate controls, and gene list used in this study are provided in the tables.
- https://cdn.elifesciences.org/articles/63768/elife-63768-supp1-v2.docx
-
Transparent reporting form
- https://cdn.elifesciences.org/articles/63768/elife-63768-transrepform-v2.docx