Multiscale analysis reveals that diet-dependent midgut plasticity emerges from alterations in both stem cell niche coupling and enterocyte size
Figures
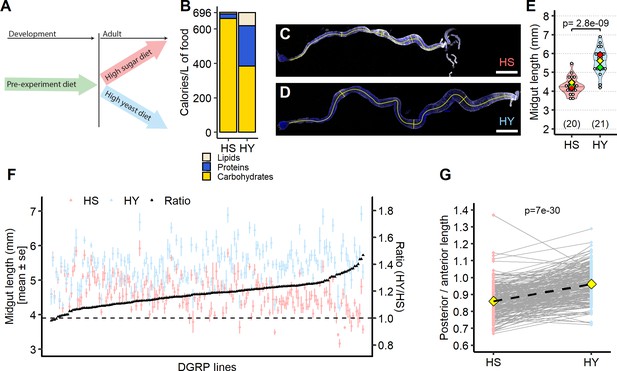
Diet composition affects size and regional allometry of the midgut.
(A) Illustration of general dietary treatment design. Flies were reared on pre-experiment diet during development. At eclosion, flies were allocated to either high sugar (HS) or high yeast (HY) before midgut dissection at 5 days post eclosion. (B) Nutritional composition (proteins, carbohydrates, and lipids) of the two isocaloric diets used as a basis for this study as calories per liter of food: enriched in sugars (HS) or yeast (HY). (C–E) Canton-S (Cs) flies fed on HS diet (C) have shorter midguts than flies on HY (D). Quantification of midgut length for HS vs. HY at 5 days post eclosion (E). (F) Midgut length response to diet is strongly variable across the Drosophila Genetic Reference Panel (DGRP), with HY being generally longer than HS (i.e., the ratio length on HY/length on HS is between 1 and 1.4). (G) Midgut resizing is allometric between regions of the midgut. Posterior midguts of flies fed HY diet exhibit a greater increase than anterior regions. For the violin/dot plots shown in this figure, white dots represent single midgut measurements. Lozenges represent mean of repeats. Violin plots are color coded according to diets (HS = red, HY = light blue throughout the article). Numbers in parentheses at the bottom of charts indicate sample size. Additional information on the statistics can be found in Supplementary file 2. Scale bars are 500 µm for all images.
-
Figure 1—source data 1
Numeric data for Figure 1B.
- https://cdn.elifesciences.org/articles/64125/elife-64125-fig1-data1-v2.csv
-
Figure 1—source data 2
Numeric data for Figure 1F and G.
- https://cdn.elifesciences.org/articles/64125/elife-64125-fig1-data2-v2.csv
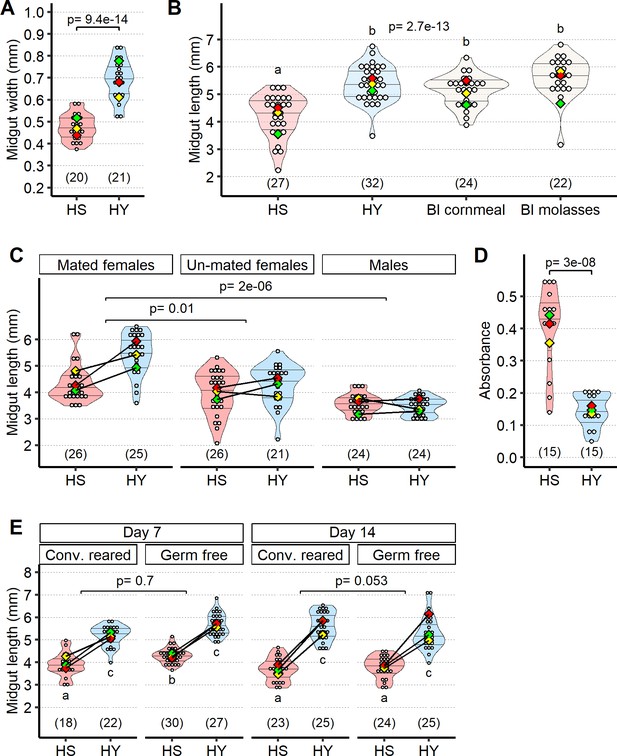
Diet composition affects midgut size independently of microbiota.
(A) Canton-S (Cs) flies fed high-sugar (HS) diet have narrower midguts than those fed high-yeast (HY) diet. Width was measured in three points along the midgut (regions 2–4, as visible in the yellow annotation in Figure 1C and D) and the sum of these three measurements was used as proxy for midgut width. Measurements are from the same guts as in Figure 1E. (B) Length of midguts on HY diet is similar to standard diets used for Drosophila research (Bloomington [Bl] cornmeal and Bl molasses). (C) Unmated females and mated males have lower response to diet compared to mated female flies. Statistics: comparison of the interaction between diet and mating status/sex. Results in A–C were obtained from 5-day-old flies shifted on experimental diets at eclosion. (D) Feeding assay shows higher dietary intake on HS than on HY diet. Absorbance measured after 1 day of assay, each day along a 5-day period from eclosion, for a total of five times per condition/repeat. (E) Microbes are not required for the difference in size observed between HS- and HY-fed flies. Germ-free flies exhibit similar diet-induced increase in size as conventionally reared flies at both 7 and 14 days post eclosion (statistics on top of violin plot: comparison of the interaction between diets and conv. reared/germ-free treatment). Of note, at 7 days post eclosion we observed longer guts in germ-free flies compared to conventionally reared flies (significant on HS diet); this difference was lost at 14 days post eclosion (post hoc Tukey test from Generalized Linear Mixed Model [GLMM] summarized by letter at the bottom of chart). For the violin/dot plots shown in this figure, white dots represent single measurements. Lozenges represent mean of repeats. Black lines connecting means visualize the interaction between diets. Numbers in parentheses at the bottom of charts indicate sample size. Additional information on the statistics can be found in Supplementary file 2.
-
Figure 1—figure supplement 1—source data 1
Numeric data for Figure 1E and Figure 1—figure supplement 1A.
- https://cdn.elifesciences.org/articles/64125/elife-64125-fig1-figsupp1-data1-v2.csv
-
Figure 1—figure supplement 1—source data 2
Numeric data for Figure 1—figure supplement 1B.
- https://cdn.elifesciences.org/articles/64125/elife-64125-fig1-figsupp1-data2-v2.csv
-
Figure 1—figure supplement 1—source data 3
Numeric data for Figure 1—figure supplement 1C.
- https://cdn.elifesciences.org/articles/64125/elife-64125-fig1-figsupp1-data3-v2.csv
-
Figure 1—figure supplement 1—source data 4
Numeric data for Figure 1—figure supplement 1D.
- https://cdn.elifesciences.org/articles/64125/elife-64125-fig1-figsupp1-data4-v2.csv
-
Figure 1—figure supplement 1—source data 5
Numeric data for Figure 1—figure supplement 1E.
- https://cdn.elifesciences.org/articles/64125/elife-64125-fig1-figsupp1-data5-v2.csv
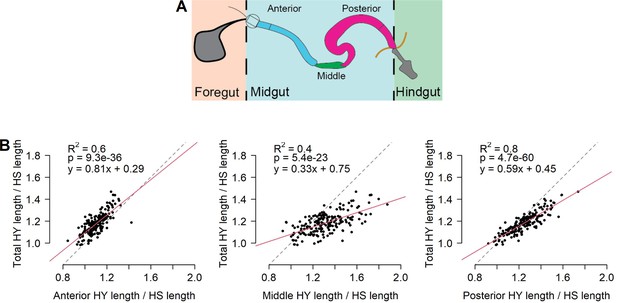
Different regions of the midgut respond variably to diet composition.
(A) Scheme depicting regional organization of the gut. The gut comprises three main anatomical regions: foregut (comprising the crop), midgut, and hindgut. The midgut itself can be divided into anterior (blue), middle (green), and posterior (purple). Additional subregions have been described (Buchon et al., 2013; Marianes and Spradling, 2013). (B) All regions of the midgut (x-axis) respond variably to diet composition, but the response of the posterior midgut length more closely reflects the response of the total midgut length. Red lines represent linear regression and black dashed lines are the lines of equivalence.
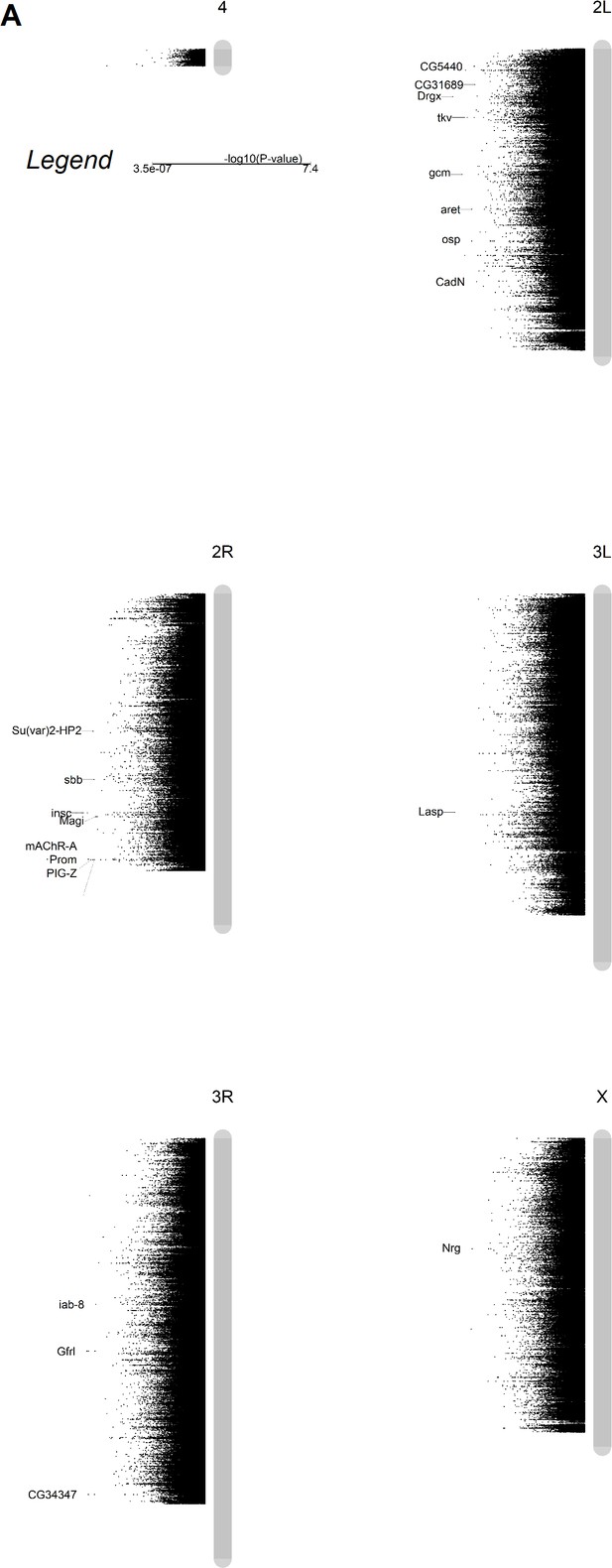
Genome-wide association identifies genes underlying natural variation in the midgut response to diet composition.
(A) Variation in impact of diet on midgut length in the Drosophila Genetic Reference Panel (DGRP) maps to genes with functions connected to epithelial turnover. The Manhattan plot summarizes the p-value per chromosomal locus (gray bars) associated with Genome-Wide Association Study (GWAS) analysis. Highlighted genes have been selected based on their statistical significance, their function, and the effect of the genetic variation (e.g., nonsynonymous mutation, etc.). More details in Figure 3—source data 1 and Figure 3—source data 2.
-
Figure 1—figure supplement 3—source data 1
Summary of GWAS analysis, shown in Figure 1—figure supplement 3A.
- https://cdn.elifesciences.org/articles/64125/elife-64125-fig1-figsupp3-data1-v2.csv
-
Figure 1—figure supplement 3—source data 2
Complete result of GWAS analysis, shown in Figure 1—figure supplement 3A.
- https://cdn.elifesciences.org/articles/64125/elife-64125-fig1-figsupp3-data2-v2.zip
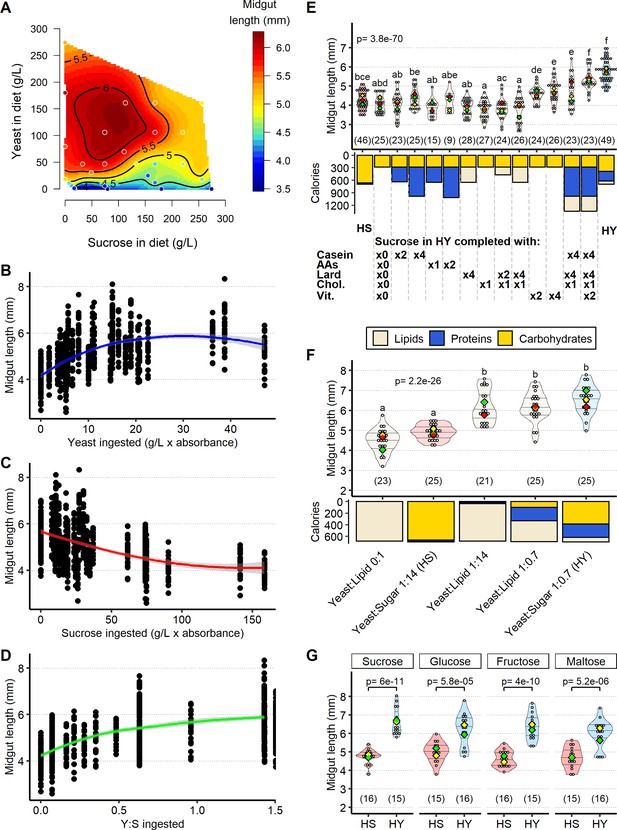
Sugar opposes yeast-induced increase of midgut length.
(A) Midgut length is maximized at specific points in diet space. Adult flies were maintained for 5 days from eclosion on one of 28 diets based on different caloric concentration and yeast to sucrose ratios (see Figure 2—figure supplement 1A for scheme on diets used and sample size). The list of recipes can be found in Supplementary file 1. The figure shows contours of a thin-plate spline (Generalized Additive Model) of length (mm, coded by colors) as a function of yeast and sucrose in diet. Colored dots represent mean of samples in a particular diet. (B–D) Yeast and sucrose have mutually opposite impacts on midgut length. Plots show an increase in midgut length with increased amount of yeast ingested (B); a decrease in midgut length with increased amount of sucrose ingested (C); and an increase in midgut length with ratio of yeast to sucrose ingested (D). (E) Several nutrients from yeast (proteins, lipids, vitamins/minerals) are required to increase midgut length. Nutrients from yeast (proteins, amino acids, lipids, cholesterol, vitamins/minerals) were added against a base diet of only the amount of sucrose found in high yeast (HY) and devoid of yeast. Letters above violin plots represent grouping by statistical differences (post hoc Tukey on GLMM). Bars beneath the main plot describe caloric content provided by the different components. (F) Midgut size is opposed by sugar, but not other added calories. Diet with only lipids, isocaloric with high-sugar (HS) and HY diets, results in midguts of lengths comparable to those on HS diet. Substitution of sucrose from HS diet with isocaloric lipids (yeast:lipid 1:14) results in midguts as long as those on HY. Midguts of flies reared on a diet substituting sucrose in HY diet with lipids (yeast:lipid 1:0.7) are also similar in length to those of flies fed HY. Letters above violin plots represent grouping by statistical differences (post hoc Tukey on GLMM). Bottom part of the chart (bar graph) describes caloric content provided by the different components. (G) Opposition by sugar of yeast-induced growth is not specific to sucrose. Statistical comparisons were performed with HS vs. HY for each sugar. All flies for experiments in this figure were moved on the experimental diets at eclosion and dissected 5 days post eclosion. For the violin/dot plots shown in this figure, white dots represent single midgut measurements. Lozenges represent mean of repeats. Violin plots are color coded according to diets (HS = red, HY = light blue, cream for other diets). Numbers in parentheses at the bottom of charts indicate sample size. Additional information on the statistics can be found in Supplementary file 2.
-
Figure 2—source data 1
Numeric data for Figure 2A–D.
- https://cdn.elifesciences.org/articles/64125/elife-64125-fig2-data1-v2.csv
-
Figure 2—source data 2
Numeric data for Figure 2E.
- https://cdn.elifesciences.org/articles/64125/elife-64125-fig2-data2-v2.csv
-
Figure 2—source data 3
Numeric data for calories in Figure 2E.
- https://cdn.elifesciences.org/articles/64125/elife-64125-fig2-data3-v2.csv
-
Figure 2—source data 4
Numeric data for Figure 2F.
- https://cdn.elifesciences.org/articles/64125/elife-64125-fig2-data4-v2.csv
-
Figure 2—source data 5
Numeric data for calories in Figure 2F.
- https://cdn.elifesciences.org/articles/64125/elife-64125-fig2-data5-v2.csv
-
Figure 2—source data 6
Numeric data for Figure 2G.
- https://cdn.elifesciences.org/articles/64125/elife-64125-fig2-data6-v2.csv
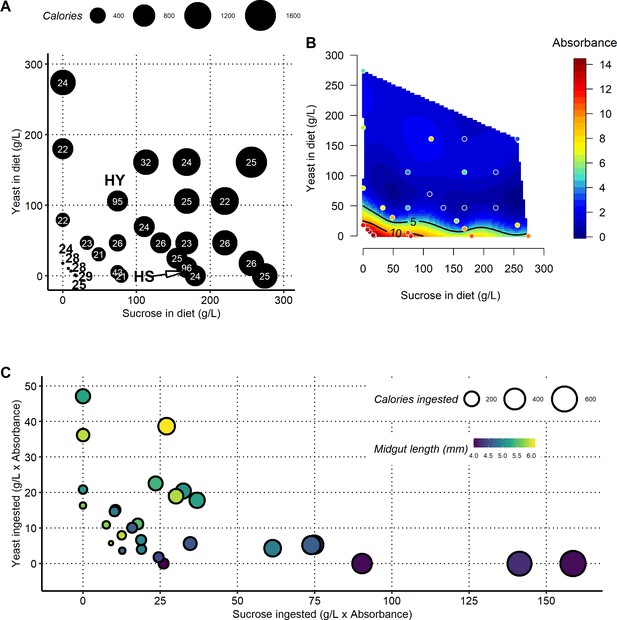
Nutritional geometry reveals the influence of yeast and sugar on midgut length.
(A) Set of diets utilized for the nutritional geometry experiment. Numbers on dots denote sample sizes for Figure 2A (pool of three independent biological replicates). We utilized 28 different diets, varying either caloric content or the yeast to sucrose ratio. The complete list of recipes can be found in Supplementary file 1. (B) FD&C1 blue transit assay (feeding assay) showing amount of food defecated, and by inference ingested, in the nutritional geometry experiment. The scale maps color to units. The graph indicates compensatory feeding at lower nutrient densities, especially low yeast. These data were used to calculate the total amount of yeast and sucrose ingested on each diet in (C). (C) Yeast and sucrose have mutually opposing effects on midgut length. Plots show midgut length as a function of sucrose or yeast ingested (g/L × Absorbance from ), or their ratio multiplied by ingestion per diet.
-
Figure 2—figure supplement 1—source data 1
Numeric data for Figure 2—figure supplement 1A.
- https://cdn.elifesciences.org/articles/64125/elife-64125-fig2-figsupp1-data1-v2.csv
-
Figure 2—figure supplement 1—source data 2
Numeric data for Figure 2—figure supplement 1B.
- https://cdn.elifesciences.org/articles/64125/elife-64125-fig2-figsupp1-data2-v2.csv
-
Figure 2—figure supplement 1—source data 3
Numeric data for Figure 2—figure supplement 1C.
- https://cdn.elifesciences.org/articles/64125/elife-64125-fig2-figsupp1-data3-v2.csv
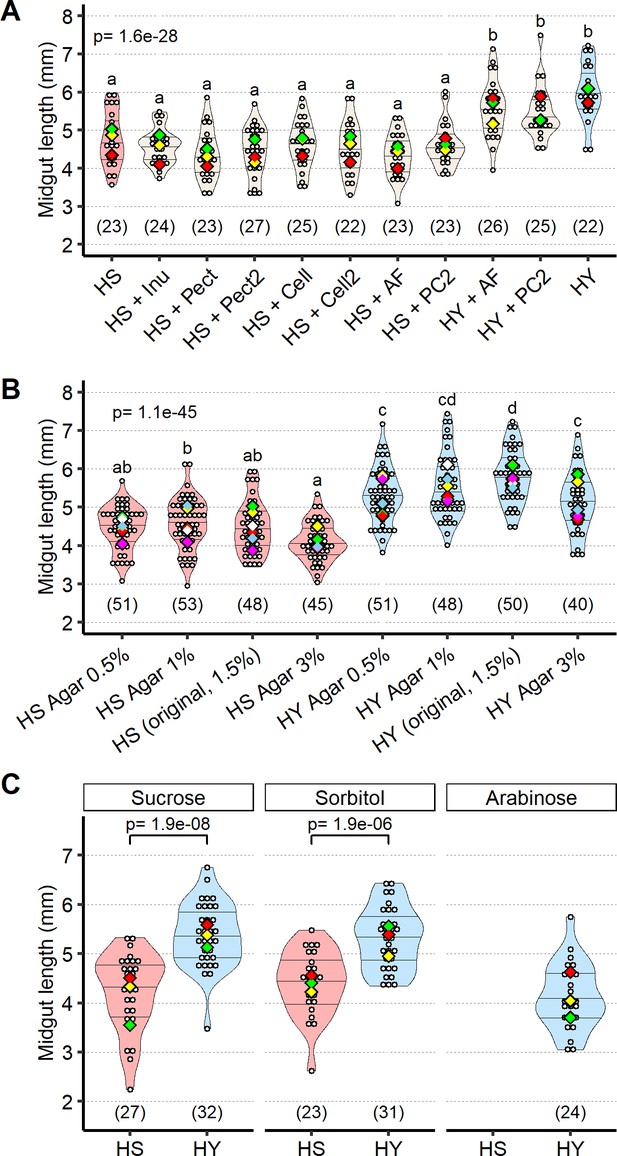
Food texture is not responsible for size differences between high-sugar (HS) and high-yeast (HY) diets.
(A, B) Food texture does not explain the differential effects of HS and HY diets on midgut length. Addition of inulin (inu), pectin (pect), cellulose (cell), and all previous fibers mixed (all fibers [AF]) or pectin + cellulose (PC) to HS does not increase midgut length. Addition of fibers to HY (HY + AF) and HY + pectin + cellulose (HY + PC2) does not affect midgut length. (B) Changes in food texture due to variation in agar concentration can affect midgut length but do not explain the effect of the dietary treatment. Changes in agar concentration do not change midgut length on HS. Either increasing or decreasing agar concentration reduces midgut length on HY. ANOVA and post hoc (Tukey) test were used for statistical analysis for (A) and (B). Letters above violin plots represent grouping by statistical differences (post hoc Tukey on GLMM). (C) Sorbitol, a nutritious but not palatable sugar, has increased size on HY compared to HS, while arabinose, a palatable but not nutritious sugar, results in death of flies before reaching dissection day on HS, and decreased size of midguts on HY diet. Statistical analysis is HS vs. HY for each sugar. All samples in this figure were moved on experimental diets at eclosion and dissected at 5 days post eclosion. For the violin/dot plots shown in this figure, white dots represent single measurements. Lozenges represent mean of repeats. Violin plots are color coded according to diets (HS = red, HY = light blue, cream for other diets). Numbers in parentheses at the bottom of charts indicate sample size. Additional information on the statistics can be found in Supplementary file 2.
-
Figure 2—figure supplement 2—source data 1
Numeric data for Figure 2—figure supplement 2A.
- https://cdn.elifesciences.org/articles/64125/elife-64125-fig2-figsupp2-data1-v2.csv
-
Figure 2—figure supplement 2—source data 2
Numeric data for Figure 2—figure supplement 2B.
- https://cdn.elifesciences.org/articles/64125/elife-64125-fig2-figsupp2-data2-v2.csv
-
Figure 2—figure supplement 2—source data 3
Numeric data for Figure 2—figure supplement 2C.
- https://cdn.elifesciences.org/articles/64125/elife-64125-fig2-figsupp2-data3-v2.csv
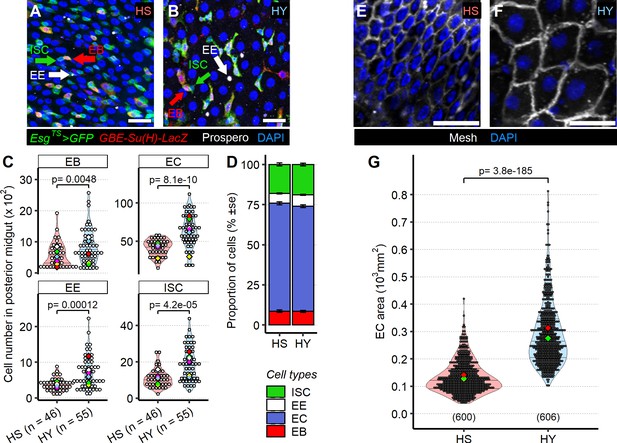
Diet composition affects both cell number and enterocyte size in the midgut.
(A–D) High-yeast (HY) diet increases numbers of all midgut cell types. Representative pictures of midguts from flies kept on high-sugar (HS) (A) or HY (B) diet. Green arrows indicate intestinal stem cells (ISCs), marked only by GFP (green), red arrows indicate enteroblasts (EBs), marked by GFP and GBE Su(H)-lacZ (red), and white arrow indicate enteroendocrine (EE) cells, marked with anti-Prospero antibody (white). All nuclei are stained with DAPI (blue). Quantification of total cell numbers in the posterior midgut (R4) for HS and HY, statistical analysis is comparing HS vs. HY for each cell type (C). HS and HY diets do not affect the relative proportion of cell types in the midgut (error is standard error of the mean D). (E–G) Diet affects enterocyte size. Representative picture of midguts stained with anti-mesh antibody on HS (E) vs. HY (F) diet. Quantification of EC size of flies on HS or HY diet confirms an increase in cell size on HY diet (G). All flies for experiments in this figure were moved on the experimental diets at eclosion and dissected 5 days post eclosion. For the violin/dot plots shown in this figure, white dots represent single midgut measurements. Lozenges represent mean of repeats. Violin plots are color coded according to diets (HS = red, HY = light blue). Numbers in parentheses at the bottom of charts indicate sample size. Additional information on the statistics can be found in Supplementary file 2. Scale bars are 25 µm for all images.
-
Figure 3—source data 1
Numeric data for Figure 3C and D and Figure 3—figure supplement 1A.
- https://cdn.elifesciences.org/articles/64125/elife-64125-fig3-data1-v2.csv
-
Figure 3—source data 2
Numeric data for Figure 3G.
- https://cdn.elifesciences.org/articles/64125/elife-64125-fig3-data2-v2.csv
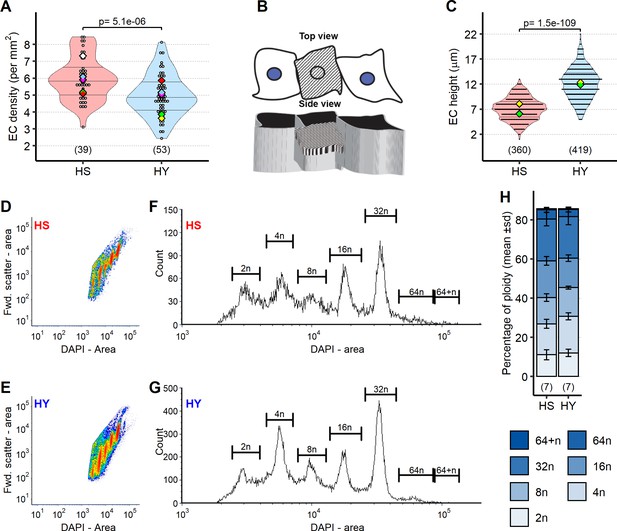
Diet composition affects enterocyte (EC) size but not the cellular composition of the midgut epithelium.
(A) ECs are more densely packed on high-sugar (HS) diet than on high-yeast (HY) diet. (B) Scheme illustrating area measurements. Top view in this scheme is as in pictures shown in Figure 3E and F. 3D side view shows side view with mesh showing measured surface. (C) Diet affects EC size. Quantification of EC height of MyoTS> GFP on HS or HY diet demonstrates an increase in cell height on HY diet. For the violin/dot plots shown in this figure, white dots represent single measurements. Lozenges represent mean of repeats. Violin plots are color coded according to diets (HS = red, HY = light blue). Numbers in parentheses at the bottom of charts indicate sample size. (D–H) Ploidy of midguts on either HS or HY diets is largely unchanged. Density plot of forward scatter area (Fwd. scatter – area) over DAPI area (DAPI – area) (D for HS, E for HY). Histogram with peak distribution and measurements (F for HS, G for HY). Stacked bar plot from seven repeats, each of 25 midguts. Error bars are standard deviation (H). Additional information on the statistics can be found in Supplementary file 2.
-
Figure 3—figure supplement 1—source data 1
Numeric data for Figure 3—figure supplement 1C.
- https://cdn.elifesciences.org/articles/64125/elife-64125-fig3-figsupp1-data1-v2.csv
-
Figure 3—figure supplement 1—source data 2
Numeric data for Figure 3—figure supplement 1H.
- https://cdn.elifesciences.org/articles/64125/elife-64125-fig3-figsupp1-data2-v2.csv
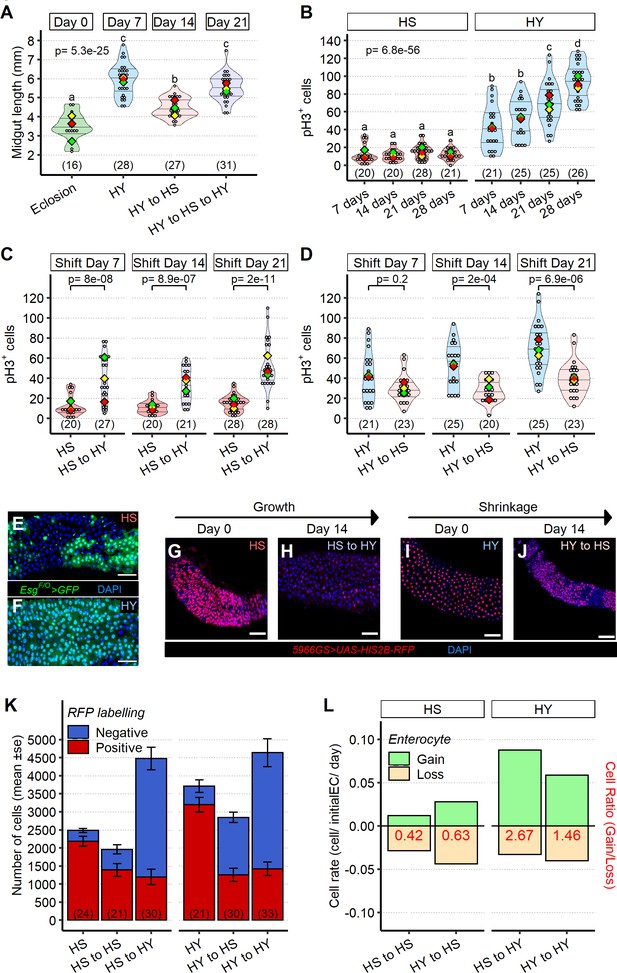
Shifts in diet composition lead to plastic midgut resizing and changes in absolute and relative cell loss and gain.
(A) Midguts can respond plastically to changes in isocaloric diets. Midgut length increases from eclosion (day 0) on high yeast (HY) for 7 days, then decreases when switched to high sugar (HS) for additional 7 days but can reincrease size upon a further 7 days HY feeding. Letters above violin plots represent grouping by statistical differences (post hoc Tukey on GLMM). (B) Mitotically active cells visualized by phospho-histone H3 (pH3) immunostaining are more numerous on HY diet than on HS diet. pH3+ cells gradually increase over time on HY, but not HS diet. Letters above violin plots represent grouping by statistical differences (post hoc Tukey on GLMM). Flies were put on the diets at eclosion, days on x-axis reflect days from eclosion. (C, D) Shifting between diets impacts pH3+ cell number in both growth (HS to HY) and shrinkage (HY to HS) experiments. Days in chart are from eclosion. Statistical comparisons are vs. pre-shift measurement. (E, F) Clonal assay with EsgF/O system put on either HS or HY from eclosion for 5 days, illustrates increased number of marked cells on HY (F) vs. HS (E) diets in region 4 of the midgut. GFP, in green, marks all cells made since the EsgF/O system was activated. (G–L) Cell loss assay enables analysis of the impact of diet composition on replacement ratio and rate. Description of experimental design is found in Materials and methods and illustrated in Figure 4—figure supplement 1H. In brief, this assay allows us to mark enterocytes (ECs) and enteroblasts (EBs) at the start of the experiment (9 days post eclosion) and to count their numbers 14 days after shifting dietary conditions (23 days post eclosion) recapitulating growth and shrinkage of the midgut, thus estimating cell gain and cell loss in these conditions. Representative pictures for the cell loss assay in growing conditions (G, H) and shrinkage conditions (I, J). In red 5966GS>His2B-RFP, marking EB and EC. Number of ECs in the posterior midgut, both marked (red, old ECs) and unmarked (blue, new ECs) by RFP, error bars are SE from three repeats (K). Data shown as rate relative to experiment start (cell/initial EC/day) (L). Number on bar in red is the ratio of EC gained/EC lost (see Materials and methods for formula). For the violin/dot plots shown in this figure, white dots represent single midgut measurements. Lozenges represent means of replicate experiments. Violin plots are color coded according to diets (green = eclosion, HS = red, HY = light blue, HY to HS = pink, HS to HY = purple). Numbers in parentheses at the bottom of charts indicate sample sizes. Additional information on statistics can be found in Supplementary file 2. Scale bars are 50 µm for all images.
-
Figure 4—source data 1
Numeric data for Figure 4A.
- https://cdn.elifesciences.org/articles/64125/elife-64125-fig4-data1-v2.csv
-
Figure 4—source data 2
Numeric data for Figure 4K.
- https://cdn.elifesciences.org/articles/64125/elife-64125-fig4-data2-v2.csv
-
Figure 4—source data 3
Numeric data for Figure 4L and Figure 4—figure supplement 2D.
- https://cdn.elifesciences.org/articles/64125/elife-64125-fig4-data3-v2.csv
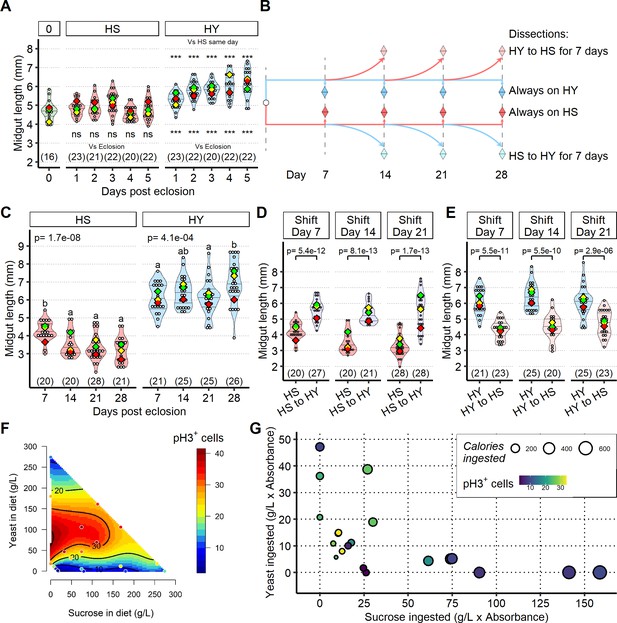
Shifts in diet composition lead to midgut resizing and are associated with changes in the absolute and relative rates of cell loss and gain.
(A) Midgut length increases progressively on high yeast (HY), but not on high sugar (HS). Statistics on top of violin plots compare HS vs. HY for each day, statistic on bottom of violin plots compare condition to eclosion, ***p<0.01, see Supplementary file 2 for further info on p-value. (B) Scheme for Figure 4B, C and D and (C), (D, E) At eclosion, flies were allocated to either HS or HY diet. 7, 14, and 21 days post eclosion, flies were either kept on the same diet or shifted on the opposite diet for 7 days (HS to HY or HY to HS). Flies were dissected every 7 days, up until day 28. (C) HS diet does not postpone post-eclosion development, but rather induces continual midgut shrinkage over 28 days of feeding. Letters above violin plots represent grouping by statistical differences (post hoc Tukey on GLMM). (D, E) Midgut size is a plastic, diet-dependent trait. Midguts of flies shifted between HS or HY can reversibly grow or shrink throughout 21 days. Statistical comparison is vs. pre-shift measurement. Day of shift is from eclosion. For the violin/dot plots shown in this figure, white dots represent single midgut measurements. Lozenges represent mean of repeats. Violin plots are color coded according to diets (green = eclosion, HS = red, HY = light blue, HY to HS = pink, HS to HY = purple). Numbers in parentheses at the bottom of charts indicate sample sizes. (F, G) Intestinal stem cell (ISC) proliferation is promoted by yeast and opposed by sugar. Cell proliferation (pH3 stain) is impeded by dietary sucrose, and increased by yeast, similar to total midgut length (Figure 2A). Plots showing counts of pH3+ cells as a function of yeast and sucrose in diet (F) and showing counts of pH3+ cell as a function of ingested yeast and sucrose (G). Additional information on the statistics can be found in Supplementary file 2.
-
Figure 4—figure supplement 1—source data 1
Numeric data for Figure 4B and Figure 4—figure supplement 1C.
- https://cdn.elifesciences.org/articles/64125/elife-64125-fig4-figsupp1-data1-v2.csv
-
Figure 4—figure supplement 1—source data 2
Numeric data for Figure 4C and Figure 4—figure supplement 1D.
- https://cdn.elifesciences.org/articles/64125/elife-64125-fig4-figsupp1-data2-v2.csv
-
Figure 4—figure supplement 1—source data 3
Numeric data for Figure 4D and Figure 4—figure supplement 1E.
- https://cdn.elifesciences.org/articles/64125/elife-64125-fig4-figsupp1-data3-v2.csv
-
Figure 4—figure supplement 1—source data 4
Numeric data for Figure 4—figure supplement 1A.
- https://cdn.elifesciences.org/articles/64125/elife-64125-fig4-figsupp1-data4-v2.csv
-
Figure 4—figure supplement 1—source data 5
Numeric data for Figure 4—figure supplement 1F and G.
- https://cdn.elifesciences.org/articles/64125/elife-64125-fig4-figsupp1-data5-v2.csv
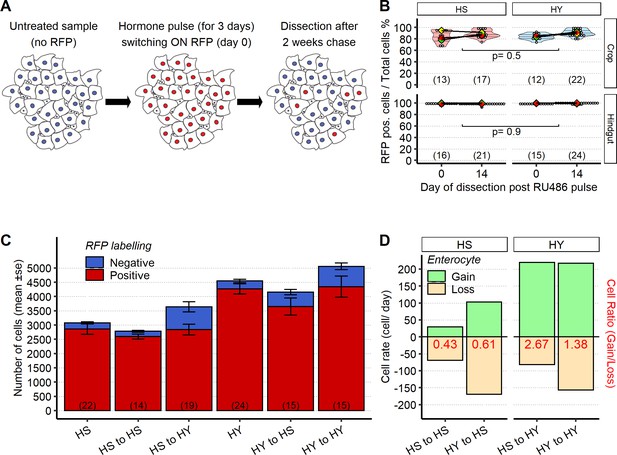
Illustration and quality control for cell loss assay.
(A) Illustration of the cell loss assay (Figure 4G,L). A pulse of RU486 for 3 days marks most enterocytes (ECs) and enteroblasts (EBs) through 5966GS>His2B-RFP. Flies were dissected at 2 and 16 days after cessation of hormone pulse. (B) His2B-RFP is highly stable on both high-sugar (HS) and high-yeast (HY) diets on tissues not undergoing turnover in a manner similar to the midgut. We assayed the stability of the His2B-RFP by driving it through an ActGS driver, in the crop and hindgut in the same timeline as the experiment presented in Figure 4K. In both organs, we found a high degree of cells marked by His2B-RFP, and on both diets, at both the initial timepoint and after 14 days from the start of the chase. Day of dissection at the bottom of the chart is relative to the start of pulse chase. The statistical comparison is comparing interaction between diet and day of dissection. For the violin/dot plot shown in this figure, white dots represent single midgut measurements. Lozenges represent mean of repeats. Black lines connecting means visualize the interaction between diets. Violin plot is color coded according to diets (HS = red, HY = light blue). (C) Cell loss assay performed at 5 days post start of chase shows limited cell loss in HY to HY condition. Number of ECs in the posterior midgut, both marked (red, old ECs) and unmarked (blue, new ECs) by RFP, error bars are SE from three repeats. (D) Diet composition modulates cell replacement rate (cell/day). Bar chart was made using the same data as in Figure 4K and L. Numbers in parentheses at the bottom of charts indicate sample sizes. Additional information on the statistics can be found in Supplementary file 2.
-
Figure 4—figure supplement 2—source data 1
Numeric data for Figure 4—figure supplement 2B.
- https://cdn.elifesciences.org/articles/64125/elife-64125-fig4-figsupp2-data1-v2.csv
-
Figure 4—figure supplement 2—source data 2
Numeric data for Figure 4—figure supplement 2C.
- https://cdn.elifesciences.org/articles/64125/elife-64125-fig4-figsupp2-data2-v2.csv
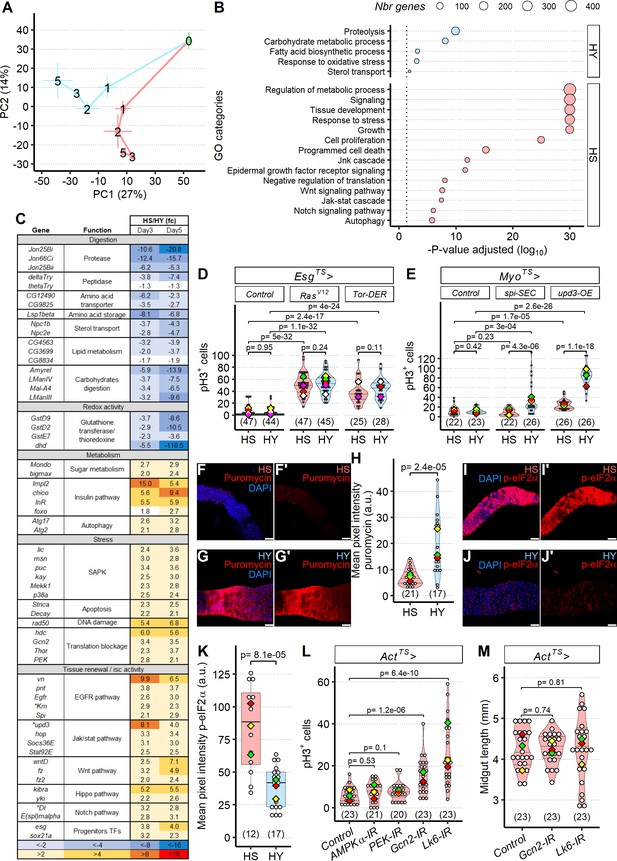
Sugar uncouples intestinal stem cell (ISC) proliferation from niche signal expression by inducing translational stress.
(A) Diet influences midgut transcriptomes after an initial programmed developmental transition. The plot shows a principal components analysis (PCA) of the whole transcriptome, with means per diet per day ± standard error (three repeats). Numbers on the plot represent the day of dissection from eclosion. Lines connect the datapoints sequentially (day 0 to day 1, day 1 to day 2, etc.), and show the divergent transcriptomic trajectory followed by midguts on the two different diets from eclosion. (B, C) Diet modulates expression of functionally distinct gene classes. Midguts of flies fed high-yeast (HY) diet show higher expression of genes with digestive functions, while high-sugar (HS) diet involves mainly genes attributed to stress response and growth. X-axis represents the statistical significance of the gene ontology (GO) categories (y-axis) after adjustment for multiple testing. Size of the dot is proportional to number of genes in the given GO category (B). Table of genes significantly differently expressed, between HS and HY, as a ratio of HS/HY, representing midgut response to HS and HY diets; additional information on the statistics is found in Materials and methods; asterisks denote genes significantly different for p-value but not for adjusted p-value (C). (D) Cell proliferation is possible on HS diet when genetically induced. Progenitor-specific (EsgTS) overexpression of a constitutively active form of Ras (UAS-RasV12) and of UAS-Tor-DER (EGFR Active), both known proliferative inducers, allows for increased proliferation on HS diet. Flies were 6 days old when dissected. (E) Enterocyte-specific overexpression (MyoTS) of UAS-upd3-OE and UAS-spi-SEC elicit increased proliferation, strongly only on HY diet, and weakly on HS with UAS-upd3-OE. Flies were 9 days old when dissected. (F–H) General translation is lower on HS than HY, shown by puromycin incorporation assay. Images show lower incorporation on HS (F, F′) than HY (G, G′) in region 4 of the midgut from 5-day-old Canton-S (Cs) flies that were shifted on HS or HY at eclosion. Quantification of mean pixel intensity of puromycin stain (H). (I–K) p-eIF2α stain is elevated on HS (I, I′) compared to HY (J, J′). Quantification of mean pixel intensity of p-eIF2α stain in region 4 of the midgut from 5-day-old Cs flies that were shifted on HS or HY at eclosion (K). (L) Re-enabling translation can restore mitosis in midguts shrinking after being shifted from HY to HS diet for 7 days. Initial shift was performed 12 days post eclosion. Blocking translational inhibition with ActTS>Gcn2 IR or ActTS>LK6 IR is sufficient to increase pH3+ cells in midguts of flies on HS diet. However, ActTS>PEK IR and ActTS>AMPKα-IR had no effect on the number of pH3+ cells. Statistical comparisons are vs. control. (M) Despite increased mitotic activity following repression of translational inhibition in ActTS>Gcn2 IR or ActTS>LK6 IR, midgut size was still reduced on flies kept on HS diet. The statistical comparison is comparing interaction between diet and fly lines. For the violin/dot plots and boxplots showed in this figure, white dots represent single midgut measurements. Lozenges represent mean of repeats. PCA, violin plots, box plots, and the PCA are color coded according to diets (green = eclosion, HS = red, HY = light blue). Numbers in parentheses at the bottom of charts indicate sample size. Additional information on the statistics can be found in Supplementary file 2. Scale bars are 50 µm for all images.
-
Figure 5—source data 1
RNA-seq count data for Figure 5A–C.
- https://cdn.elifesciences.org/articles/64125/elife-64125-fig5-data1-v2.csv
-
Figure 5—source data 2
Selected gene ontology (GO) categories for Figure 5B.
- https://cdn.elifesciences.org/articles/64125/elife-64125-fig5-data2-v2.csv
-
Figure 5—source data 3
All gene ontology (GO) results for Figure 5B.
- https://cdn.elifesciences.org/articles/64125/elife-64125-fig5-data3-v2.csv
-
Figure 5—source data 4
Differentially expressed genes for high sugar (HS) vs. high yeast (HY) (days 3 and 5), from which representative genes are shown in Figure 5C.
- https://cdn.elifesciences.org/articles/64125/elife-64125-fig5-data4-v2.csv
-
Figure 5—source data 5
Numeric data for Figure 5D.
- https://cdn.elifesciences.org/articles/64125/elife-64125-fig5-data5-v2.csv
-
Figure 5—source data 6
Numeric data for Figure 5E.
- https://cdn.elifesciences.org/articles/64125/elife-64125-fig5-data6-v2.csv
-
Figure 5—source data 7
Numeric data for Figure 5H.
- https://cdn.elifesciences.org/articles/64125/elife-64125-fig5-data7-v2.csv
-
Figure 5—source data 8
Numeric data for Figure 5K.
- https://cdn.elifesciences.org/articles/64125/elife-64125-fig5-data8-v2.csv
-
Figure 5—source data 9
Numeric data for Figure 5L.
- https://cdn.elifesciences.org/articles/64125/elife-64125-fig5-data9-v2.csv
-
Figure 5—source data 10
Numeric data for Figure 5M.
- https://cdn.elifesciences.org/articles/64125/elife-64125-fig5-data10-v2.csv
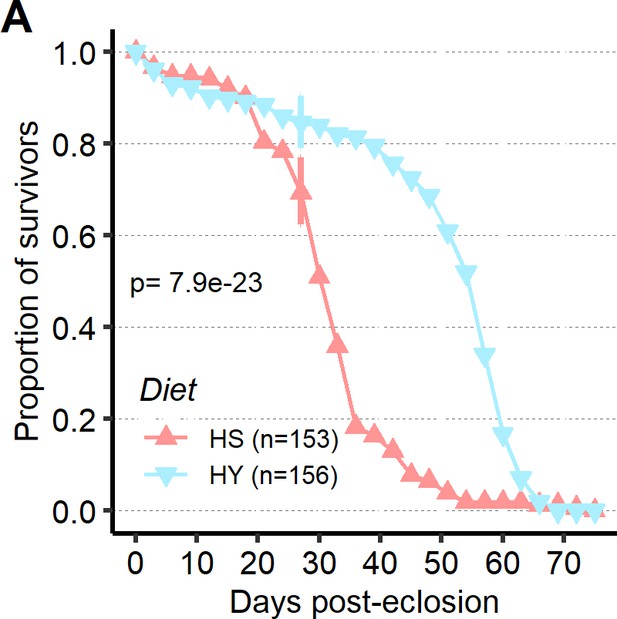
High-sugar (HS) diet is associated with shorter lifespan.
(A) Survival assay shows lower survival of flies on HS than on high-yeast (HY) diet. Additional information on the statistics can be found in Supplementary file 2. Triangles represent the day when survival was recorded.
-
Figure 5—figure supplement 1—source data 1
Numeric data for Figure 5—figure supplement 1A.
- https://cdn.elifesciences.org/articles/64125/elife-64125-fig5-figsupp1-data1-v2.csv
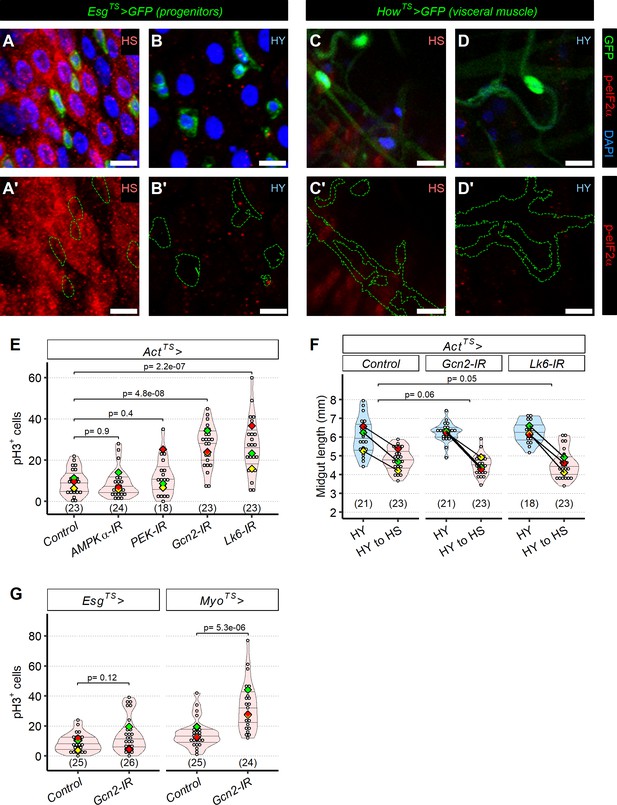
Translational stress is upregulated in the epithelium on high-sugar (HS) diet.
(A–D′) p-eIF2α stain is elevated in all cells of the epithelium on HS diet (A, A′) compared to high-yeast (HY) diet (B, B′), and less strongly in visceral muscle (C–D′). In red anti-p-eIF2α stain together with EsgTS>UAS GFP in green (progenitor cells marker) in (A, B), or alone in (A′, B′) (green dotted line is annotating the area of GFP expression). (A–B′) are maximum intensity projection of z-stack. In red anti-p-eIF2α stain together with HowTS>UAS GFP in green (Visceral muscle marker) in (C, D) or alone in (C′, D′) (green dotted line is annotating the area of GFP expression) (single z-stack). Flies were 12 days old when dissected. (E) Blocking translational inhibition with ActTS>Gcn2 IR or ActTS>LK6 IR is sufficient to increase pH3+ cells in midguts of flies shifted from HY to HS diet. However, ActTS>PEK IR and ActTS>AMPKα-IR had no effect on the number of pH3+ cells. Statistical comparisons are vs. control. Flies were 19 days old when dissected. (F) Despite increased mitotic activity following repression of translational inhibition in ActTS>Gcn2 IR or ActTS>LK6 IR, midgut size was still shrinking on flies shifted after 12 days from eclosion on HY to HS diet for additional 7 days. The statistical comparison is comparing interaction between diet and fly lines. (G) Knockdown of Gcn2 in ECs (MyoTS>Gcn2 IR), but not in progenitor cells (EsgTS>Gcn2 IR), is sufficient to increase pH3+ cells in midguts of flies shifted after 12 days from eclosion on HY to HS diet for additional 7 days. Statistical comparisons are vs. respective controls. Flies were 19 days old when dissected. Scale bars are 10 µm. For the violin/dot plots shown in this figure, white dots represent single midgut measurements. Lozenges represent mean of repeats. Black lines connecting means visualize the interaction between diets. Violin plots are color coded according to diets (HS = red, HY = light blue, HY to HS = pink). Numbers in parentheses at the bottom of charts indicate sample size. Additional information on the statistics can be found in Supplementary file 2.
-
Figure 5—figure supplement 2—source data 1
Numeric data for Figure 5—figure supplement 2E.
- https://cdn.elifesciences.org/articles/64125/elife-64125-fig5-figsupp2-data1-v2.csv
-
Figure 5—figure supplement 2—source data 2
Numeric data for Figure 5—figure supplement 2F.
- https://cdn.elifesciences.org/articles/64125/elife-64125-fig5-figsupp2-data2-v2.csv
-
Figure 5—figure supplement 2—source data 3
Numeric data for Figure 5—figure supplement 2G.
- https://cdn.elifesciences.org/articles/64125/elife-64125-fig5-figsupp2-data3-v2.csv
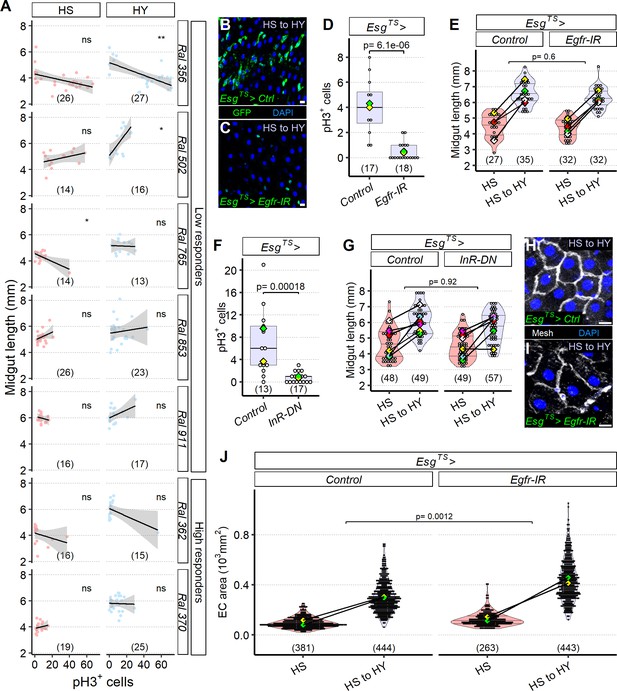
The midgut can resize independently of stem cell proliferation.
(A) Mitotic index does not correlate with midgut length. Quantification of pH3+ cells across a selected panel of high and low responder Drosophila Genetic Reference Panel (DGRP) lines shows that midgut length does not correlate with cell proliferation, flies were moved on the experimental diets at eclosion and dissected 5 days post eclosion. (B–E) Blocking EGF signaling with UAS-Egfr-IR in progenitor cells (C) results in a change in conformation of progenitor cells, which assume a more rounded shape compared to the traditional triangular shape and seem to be less in number (not quantified) compared to control (B), consistent with lower pH3+ counts than control (D). Statistical comparison for (D) is for the interaction between diet and genotype. Data for (D) is from 19 days post eclosion. However, these EsgTS>UAS-Egfr-IR midguts are still able to reach a similar length to controls (E). In (E), high sugar (HS) was dissected at 12 days post eclosion, and HS to high yeast (HY) 7 days later. (F, G) Insulin signaling with a dominant negative construct in progenitor cells results in less proliferation (F) despite EsgTS>UAS-InR-DN resulting in the same midgut length growth as the control (G). Statistical comparison for (G) is for the interaction between diet and genotype. Data for (F) is from 19 days post eclosion. In (G), HS was dissected at 12 days post eclosion, and HS to HY 7 days later. (H–J) Increase in midgut length despite proliferation blockage is accompanied with compensatory area increase of enterocyte (EC). Representative pictures of midguts stained with membrane marker mesh (white), shifted from HS to HY at 12 days post eclosion, and kept on HY for seven additional days show bigger cells on EsgTS>UAS-Egfr-IR (I) compared to control (H). Quantification of EC cell size shows compensatory effect in ECs (J). Statistical comparisons for (E, G, J) are for the interaction between diet and genotype. For the violin/dot plots and boxplots showed in this figure, white dots represent single midgut measurements. Lozenges represent mean of repeats. Violin plots and box plots are color coded according to diets (HS = red, HY = light blue, HS to HY = purple). Black lines connecting means visualize the interaction between diets. Numbers in parentheses at the bottom of charts indicate sample sizes. Additional information on the statistics can be found in Supplementary file 2. Scale bars are 10 µm for all images.
-
Figure 6—source data 1
Numeric data for Figure 6A, Figure 6—figure supplement 1A, Figure 7A, and Figure 7—figure supplement 1B.
- https://cdn.elifesciences.org/articles/64125/elife-64125-fig6-data1-v2.csv
-
Figure 6—source data 2
Numeric data for Figure 6D.
- https://cdn.elifesciences.org/articles/64125/elife-64125-fig6-data2-v2.csv
-
Figure 6—source data 3
Numeric data for Figure 6E.
- https://cdn.elifesciences.org/articles/64125/elife-64125-fig6-data3-v2.csv
-
Figure 6—source data 4
Numeric data for Figure 6F.
- https://cdn.elifesciences.org/articles/64125/elife-64125-fig6-data4-v2.csv
-
Figure 6—source data 5
Numeric data for Figure 6G.
- https://cdn.elifesciences.org/articles/64125/elife-64125-fig6-data5-v2.csv
-
Figure 6—source data 6
Numeric data for Figure 6J.
- https://cdn.elifesciences.org/articles/64125/elife-64125-fig6-data6-v2.csv
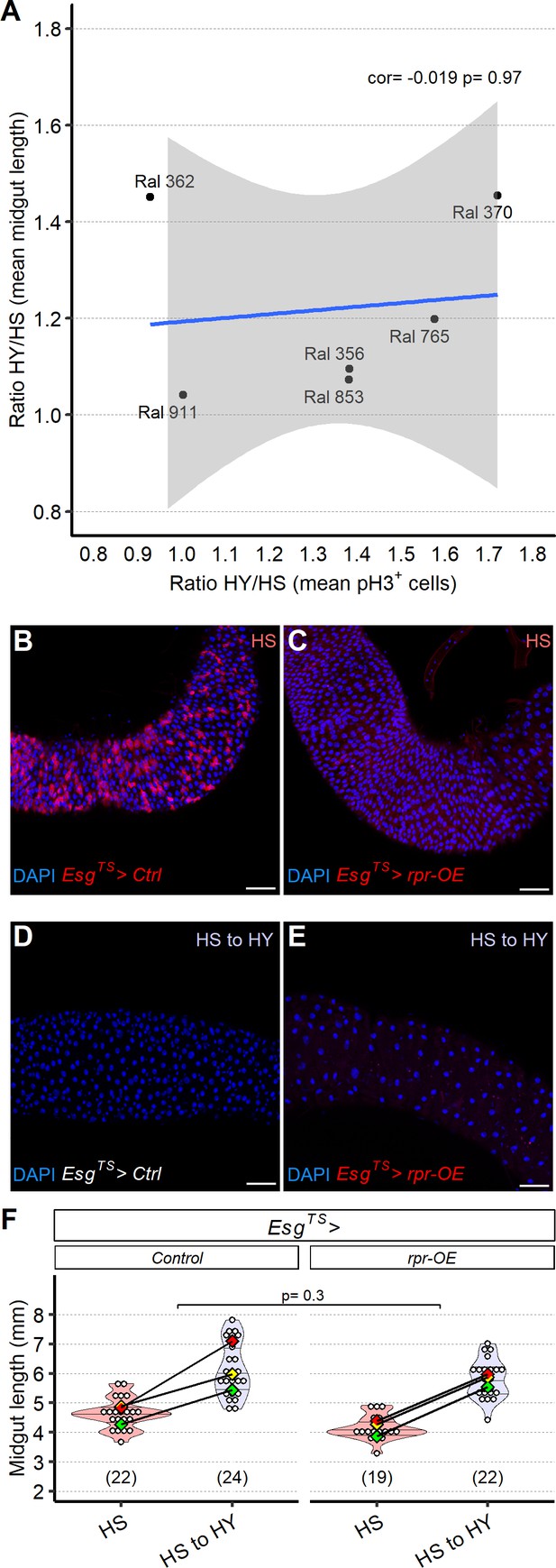
Diet-dependent changes in mitotic index do not correlate with changes in midgut size.
(A) Changes in the number of pH3+ cells between high sugar (HS) and high yeast (HY) (ratio HY/HS of mean pH3+ cells) does not correlate with changes in midgut size between HS and HY (ratio HY/HS of mean midgut length) across selected Drosophila Genetic Reference Panel (DGRP) lines. (B–F) Overexpression of reaper in progenitor cells (marked in red) via EsgTS>rpr OE results in loss of marked progenitor cells (C) compared to control (B) in region 4 of midguts, after 12 days post eclosion on HS diet (7 days at 29°C with TARGET system active). Shifting the flies on HY for additional 7 days results in a change in morphology on midguts overexpressing reaper, reminiscent of EsgTS>UAS-Egfr-IR midguts (E), while the control retains normal morphology (D, DAPI only to better show midgut morphology). However, these EsgTS>rpr OE midguts are still able to reach a similar length to controls (F). Statistical comparison for (F) is for the interaction between diet and genotype. Scale bars are 50 µm. For the violin/dot plot shown in this figure, white dots represent single midgut measurements. Lozenges represent mean of repeats. Violin plot is color coded according to diets (HS = red, HS to HY = purple). Numbers in parentheses at the bottom of chart indicate sample size. Additional information on the statistics can be found in Supplementary file 2.
-
Figure 6—figure supplement 1—source data 1
Numeric data for Figure 6—figure supplement 1F.
- https://cdn.elifesciences.org/articles/64125/elife-64125-fig6-figsupp1-data1-v2.csv
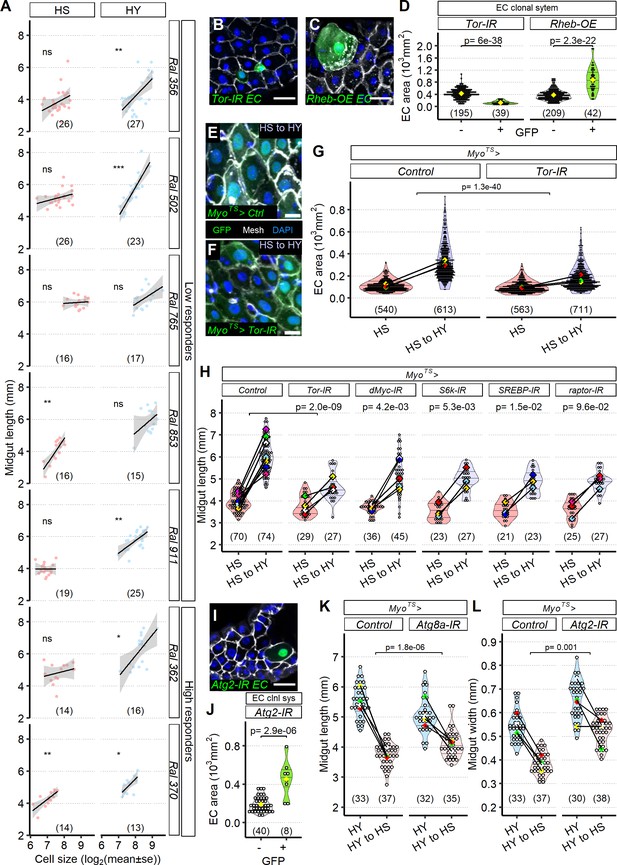
Enterocyte (EC) resizing is required for midgut plasticity.
(A) EC size mostly correlates with midgut length. Quantification of EC area across a selected panel of Drosophila Genetic Reference Panel (DGRP) lines comprising high and low responder shows that midgut length mostly correlates with EC cell area, especially in high-yeast (HY) diet. Lines on plot show smoothed splines. Flies were moved on the experimental diets at eclosion and dissected 5 days post eclosion. (B–H) The TOR pathway regulates cell size. Representative pictures of single-cell clones (hsFlp; Act >STOP>Gal4, UAS-GFP) suggest that compared to GFP- cells TOR downregulation (UAS-Tor-IR, GFP+, B) results in smaller cells, while TOR hyperactivity (UAS-Rheb-OE, C) increases cell size. Quantification of clone size in (D). Single-cell clones are marked with GFP (green). Dissection for clones was performed 14 days post eclosion. Knockdown of TOR with MyoTS, an EC-specific driver, leads to the increased number of small ECs (F) compared to control (E); EC-specific GFP is indeed visible in small cells. Quantification of EC area (G). Accordingly, blocking TOR pathway components in ECs (MyoTS) inhibits diet-induced midgut growth. Control showed in chart is representative of multiple experiments. Statistical analyses were performed only on appropriate repeat/experiment and comparing interaction between diet and fly line. For (E–H), flies were dissected for the first timepoint (HS) at 12 days post eclosion, and then shifted on HY for additional 7 days. (I, J) Representative picture utilizing single-cell clonal system suggests that blocking Atg2 (hsFlp; Act>STOP>Gal4, UAS-GFP>UAS-Atg2-IR, GFP+ cells) results in bigger ECs compared to control GFP- cells, quantified in (J). Dissection for clones was performed 14 days post eclosion. (K, L) Blocking autophagy reduces midgut resizing upon shrinkage (HY to HS for 7 days). Blocking Atg8a expression with RNAi in ECs (MyoTS>UAS-Atg8a-IR) results in less length shrinkage compared to control midguts. Blocking Atg2 expression with RNAi in ECs (MyoTS>UAS-Atg2-IR) results in less width shrinkage compared to control midguts. For (J, K), flies were dissected at the first timepoint (HY) at 12 days post eclosion, and then shifted on HS for additional 7 days. For the violin/dot plots and boxplots showed in this figure, white dots represent single midgut measurements. Lozenges represent mean of repeats. Violin plots and box plots are color coded according to diets for (G, H, K, L) (HS = red, HY = light blue, HY to HS = pink, HS to HY = purple) or to condition for (D, J) (GFP+ in green, GFP- in gray). Black lines connecting means visualize the interaction between diets. Numbers in parentheses at the bottom of charts indicate sample size. Additional information on the statistics can be found in Supplementary file 2. Scale bars are 25 µm for (B, C, I) and 10 µm for (E, F).
-
Figure 7—source data 1
Numeric data for Figure 7D.
- https://cdn.elifesciences.org/articles/64125/elife-64125-fig7-data1-v2.csv
-
Figure 7—source data 2
Numeric data for Figure 7G.
- https://cdn.elifesciences.org/articles/64125/elife-64125-fig7-data2-v2.csv
-
Figure 7—source data 3
Numeric data for Figure 7H.
- https://cdn.elifesciences.org/articles/64125/elife-64125-fig7-data3-v2.csv
-
Figure 7—source data 4
Numeric data for Figure 7J.
- https://cdn.elifesciences.org/articles/64125/elife-64125-fig7-data4-v2.csv
-
Figure 7—source data 5
Numeric data for Figure 7K.
- https://cdn.elifesciences.org/articles/64125/elife-64125-fig7-data5-v2.csv
-
Figure 7—source data 6
Numeric data for Figure 7L.
- https://cdn.elifesciences.org/articles/64125/elife-64125-fig7-data6-v2.csv
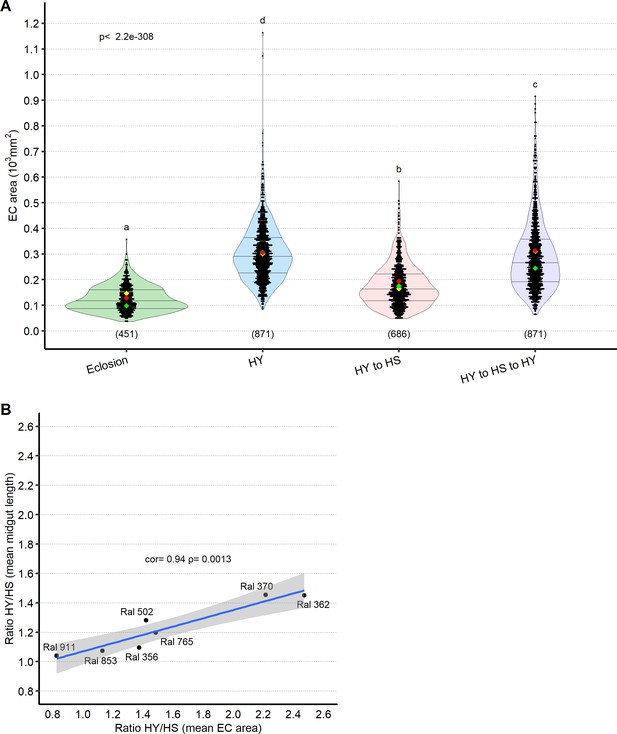
Diet-dependent changes in enterocyte (EC) size correlate with changes in midgut size.
(A) The midgut plastically resizes through changes in EC cell size. Quantification of EC area shows that, in addition to total length, the midgut is also able to plastically resize (Figure 4A) its own ECs. ECs scored are derived from midguts measured for length in Figure 4A. Flies have been kept for 7 days on the new diet after each shift at the moment of dissection. Letters above violin plots represent grouping by statistical differences (post hoc Tukey on GLMM). (B) Changes in EC area between high sugar (HS) and high yeast (HY) (ratio HY/HS of mean EC area) significantly correlate with changes in midgut size between HS and HY (ratio HY/HS of mean midgut length) across selected Drosophila Genetic Reference Panel (DGRP) lines. For the violin/dot plot shown in this figure, white dots represent single midgut measurements. Lozenges represent mean of repeats. Violin plot is color coded according to diets (green = eclosion, HS = red, HY = light blue, HY to HS = pink, HS to HY = purple). Numbers in parentheses at the bottom of charts indicate sample size. Additional information on the statistics can be found in Supplementary file 2.
-
Figure 7—figure supplement 1—source data 1
Numeric data for Figure 7—figure supplement 1A.
- https://cdn.elifesciences.org/articles/64125/elife-64125-fig7-figsupp1-data1-v2.csv
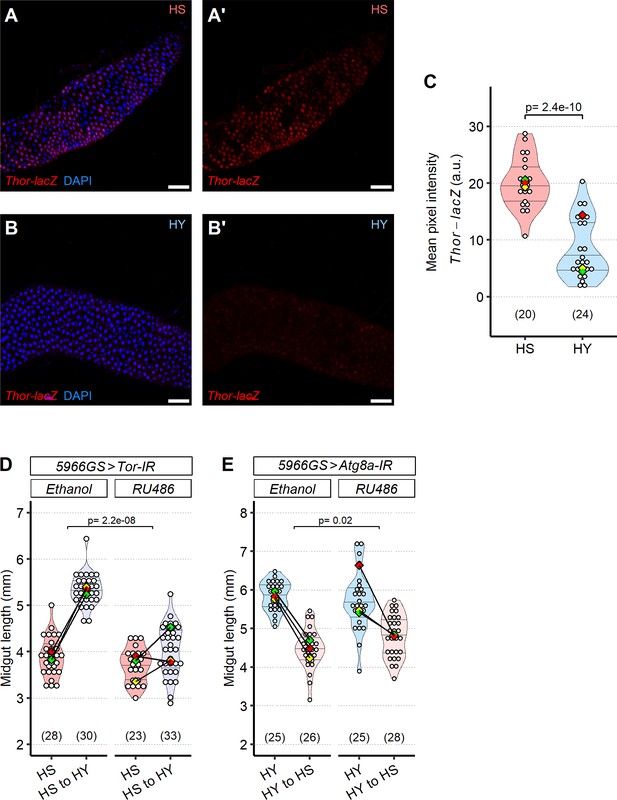
The TOR pathway regulates cell size.
(A–C) A reporter line for Foxo pathway activity, thor-lacZ, has increased intensity on high sugar (HS) (A, A′) compared to high yeast (HY) (B, B′). Quantification of mean pixel intensity of thor-lacZ stain (C). (D) Blocking TOR in enterocytes (ECs) (5966GS>UAS-Tor-IR) inhibits diet-induced midgut growth. (E) Blocking autophagy in ECs reduces midgut resizing upon shrinkage (HY to HS for 7 days) using 5966GS>UAS-Atg8a-IR. For (D, E) control, we used flies of the same genotype but not exposed to RU486. Statistical analyses were performed by comparing interaction between diet and fly line. For the violin/dot plots shown in this figure, white dots represent single midgut measurements. Lozenges represent mean of repeats. Violin plots are color coded according to diets (HS = red, HY = light blue, HY to HS = pink, HS to HY = purple). Numbers in parentheses at the bottom of charts indicate sample size. Additional information on the statistics can be found in Supplementary file 2.
-
Figure 7—figure supplement 2—source data 1
Numeric data for Figure 7—figure supplement 2C.
- https://cdn.elifesciences.org/articles/64125/elife-64125-fig7-figsupp2-data1-v2.csv
-
Figure 7—figure supplement 2—source data 2
Numeric data for Figure 7—figure supplement 2D.
- https://cdn.elifesciences.org/articles/64125/elife-64125-fig7-figsupp2-data2-v2.csv
-
Figure 7—figure supplement 2—source data 3
Numeric data for Figure 7—figure supplement 2E.
- https://cdn.elifesciences.org/articles/64125/elife-64125-fig7-figsupp2-data3-v2.csv
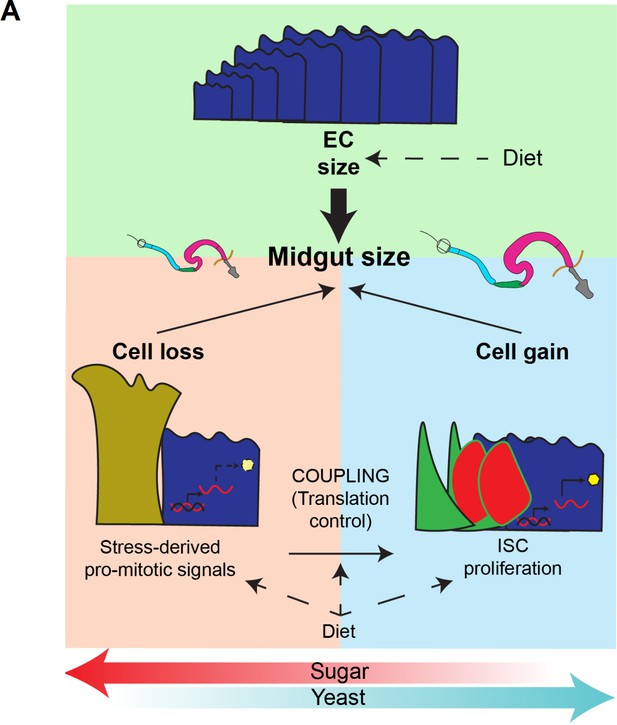
A model of diet-dependent midgut plasticity.
(A) Scheme depicting the regulation of midgut size in response to dietary changes. Enterocyte (EC) size, together with the balance between cell gain and cell loss, determines midgut size. Diet can influence these three parameters, thus influencing midgut size. Yeast promotes midgut growth, and sugar opposes it. Sugar inhibits translation and uncouples intestinal stem cell (ISC) proliferation from expression of stress-derived pro-mitotic signals, thus resulting in smaller guts.

Data shown as rate relative to experiment start (cell /initial EC/ day) for Figure 4-figure supplement 2C (5 days post chase start, 15 days from eclosion).</Author response image 1 title/legend>.
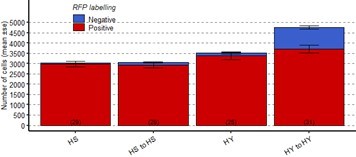
Number of ECs in the posterior midgut, both marked (Red, old ECs) and unmarked (Blue, new ECs) by RFP, error bars are SE from 3 repeats, for the experiment described above.
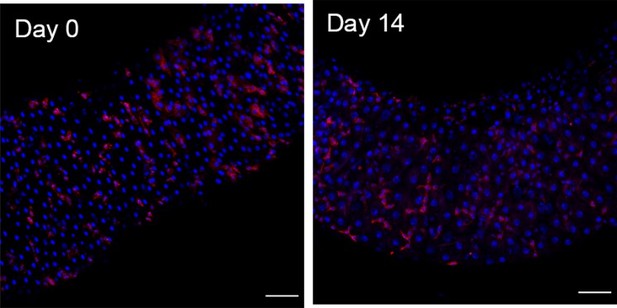
EsgTS>UAS-RFP at the start of the chase (Day0) vs at the end of it (Day14) for region 4 of the midgut.
Scale bars are 50 µm.

Percentages of RFP+ cells (RFP) over total number of cells (Dapi) at the start of a pulse chase experiment.
Videos
Nano CT scan and rendering of internal organs.
Video shows flies kept on high-sugar (HS) diet on the left, and flies kept on high-yeast (HY) diet on the right. At 5 s, a rendering of the ovaries appears in blue, showing increased size on HY diet. At 10 s, a rendering of the midgut (anterior and middle in light blue, posterior in green, and hindgut in purple) appears, showing increased size of the midgut on HY diet.
Tables
Reagent type (species) or resource | Designation | Source or reference | Identifiers | Additional information |
---|---|---|---|---|
genetic reagent (D. melanogaster) | Canton-S | Bloomington Drosophila Stock Center | BDSC: 64349FLYB: FBst0064349RRID:BDSC_64349 | Flybase genotype: Canton-S |
genetic reagent (D. melanogaster) | EsgTS | Micchelli and Perrimon, 2006 | N/A | w-;Esg-Gal4; UAS-GFP, tub-Gal80TS |
genetic reagent (D. melanogaster) | EsgTS | Nagy et al., 2018 | N/A | w-;Esg-Gal4,tub-Gal80TS,UAS-mcherry-CD8 |
genetic reagent (D. melanogaster) | EsgF/O | Jiang et al., 2011 | N/A | w-;Esg-Gal4, UAS-GFP, tub-Gal80TS; Act > STOP > Gal4,UAS-flp |
genetic reagent (D. melanogaster) | MyoTS | Buchon et al., 2010 | N/A | w-; Myo1A-Gal4, UAS-GFP, tub-Gal80TS; upd3-lacZ |
genetic reagent (D. melanogaster) | ActTS | This publication | N/A | Actin5C-Gal4/Cyo; TubGal80ts,UasGFP |
genetic reagent (D. melanogaster) | 5,966GS | Guo et al., 2013 | FLYB: FBti0150384 | w-; 5,966GS/Cyo |
genetic reagent (D. melanogaster) | Single cell clonal system | Ito et al., 1997 | N/A | hsFlp; Act > STOP > Gal4,UAS-GFP |
genetic reagent (D. melanogaster) | ActGS | Bloomington Drosophila Stock Center | BDSC: 9431FLYB: FBst0009431RRID: BDSC_9431 | P{ry[+ t7.2] = hsFLP}12,y[1] w[*]; P{w[+ mC] = UAS GFP.S65T}Myo31DF[T2]; P{w[+ mC] = Act5 C(-FRT)GAL4.Switch.PR}3/TM6B, Tb[1] |
genetic reagent (D. melanogaster) | UAS-His-RFP | Mayer et al., 2005 | N/A | UAS-Histone2B-RFP |
genetic reagent (D. melanogaster) | UAS-Gcn2-IR | Bloomington Drosophila Stock Center | BDSC: 67215FLYB: FBst0067215RRID: BDSC_67215 | |
genetic reagent (D. melanogaster) | UAS-LK6-IR | Bloomington Drosophila Stock Center | BDSC: 60003FLYB: FBst0060003RRID: BDSC_60003 | |
genetic reagent (D. melanogaster) | UAS-EGFR-IR | Bloomington Drosophila Stock Center | BDSC: 60012FLYB: FBst0060012RRID: BDSC_60012 | |
genetic reagent (D. melanogaster) | UAS-Tor-IR | Bloomington Drosophila Stock Center | BDSC: 34639FLYB: FBst0034639RRID: BDSC_34639 | |
genetic reagent (D. melanogaster) | UAS-Myc-IR | Bloomington Drosophila Stock Center | BDSC: 36123FLYB: FBst0036123RRID: BDSC_36123 | |
genetic reagent (D. melanogaster) | UAS-raptor-IR | Bloomington Drosophila Stock Center | BDSC: 34814FLYB: FBst0034814RRID: BDSC_34814 | |
genetic reagent (D. melanogaster) | UAS-S6k-IR | Bloomington Drosophila Stock Center | BDSC: 41702FLYB: FBst0041702RRID: BDSC_41702 | |
genetic reagent (D. melanogaster) | UAS-SREBP-IR | Bloomington Drosophila Stock Center | BDSC: 34073FLYB: FBst0034073RRID: BDSC_34073 | |
genetic reagent (D. melanogaster) | UAS-RagA-B-IR | Bloomington Drosophila Stock Center | BDSC: 34590FLYB: FBst0034590RRID: BDSC_34590 | |
genetic reagent (D. melanogaster) | UAS-RagC-D-IR | Bloomington Drosophila Stock Center | BDSC: 32342FLYB: FBst0032342RRID: BDSC_32342 | |
genetic reagent (D. melanogaster) | UAS-Atg8a-IR | Bloomington Drosophila Stock Center | BDSC: 34340FLYB: FBst0034340RRID: BDSC_34340 | |
genetic reagent (D. melanogaster) | UAS-Atg2-IR | Bloomington Drosophila Stock Center | BDSC: 34719FLYB: FBst0034719RRID: BDSC_34719 | |
genetic reagent (D. melanogaster) | UAS-Ras85DV1 | Bloomington Drosophila Stock Center | BDSC: 64195FLYB: FBst0064195RRID: BDSC_64195 | |
genetic reagent (D. melanogaster) | UAS-Tor-DER | gift from M. Freeman | N/A | yw; Pw+; (UAS torD-DER) II RJH430, |
genetic reagent (D. melanogaster) | UAS-upd3-OE | gift of M. Crozatier Brown et al., 2001 | N/A | |
genetic reagent (D. melanogaster) | UAS-spi-SEC | Bloomington Drosophila Stock Center | BDSC: 58436FLYB: FBst0058436RRID: BDSC_58436 | |
genetic reagent (D. melanogaster) | UAS-Rheb-OE | Bloomington Drosophila Stock Center | BDSC: 9690FLYB: FBst009690RRID: BDSC_9690 | |
genetic reagent (D. melanogaster) | UAS-rpr-OE | Bloomington Drosophila Stock Center | BDSC: 5823FLYB: FBst005823RRID: BDSC_5823 | |
genetic reagent (D. melanogaster) | UAS-InR-DN | Bloomington Drosophila Stock Center | BDSC: 8252FLYB: FBst008252RRID: BDSC_8252 | The 8,252 line was backcrossed six times into an outbred population with a w-Dah background |
genetic reagent (D. melanogaster) | GBE-Su(H)-lacZ | Micchelli and Perrimon, 2006 | N/A | |
genetic reagent (D. melanogaster) | thor-lacZ | Bloomington Drosophila Stock Center | BDSC: 9558FLYB: FBst009558RRID: BDSC_9558 | |
genetic reagent (D. melanogaster) | DGRP panel | Mackay et al., 2012 | N/A | Lines used are reported in Figure 1—source data 2 |
chemical compound, drug | RU486 | Sigma-Aldrich | Cat# M8046;Puchem#: 24278572 | 100 μL of a 5 mg/mL solution of RU486 per vial |
chemical compound, drug | FD&C1 blue dye | VWR | Cat# 700010–048; MDL# MFCD00012141 | |
chemical compound, drug | Puromycin | Sigma Aldrich | Cat# P8833;MDL# MFCD00012691; PubChem# 24898984 | |
chemical compound, drug | Trizol | Life Technologies | Cat#15596018 | |
other | Yeast | MP biomedicals | Cat# 903,312 | |
other | inulin | VWR | Cat# CAAAA18425-09 | |
other | cellulose | VWR | Cat# IC19149980 | |
other | pectin | Sigma Aldrich | Cat# P9135 | |
other | lard | Walmart | Land o lakes | |
other | casein | Sigma Aldrich | Cat# C5679 | |
other | AA mix | Harlan Laboratories, Lee and Micchelli, 2013 | Cat# TD.10473 & TD.110036 | |
other | vitamin and mineral mix | Harlan Laboratories; Lee and Micchelli, 2013 | Cat# TD.10475 | |
other | cholesterol | Sigma Aldrich | Cat# C8667 | |
other | moldex | Neta Scientific | Cat# QB-A611-0572-159 | |
other | yellow cornmeal | Walmart | Aunt Jemima | |
other | glucose | Alfa Aesar | Cat# A16828 | |
other | maltose | Sigma Aldric | Cat# M5885 | |
other | fructose | Sigma Aldrich | Cat# F0127 | |
other | arabinose | VWR | Cat# 80502–266 | |
other | sorbitol | VWR | Cat# 76177–308 | |
other | nutri-fly BL cornmeal | Genesee scientific | Cat# 66–112 | |
other | BL Molasses | Genesee scientific | Cat# 66–116 | |
other | DAPI | Sigma-Aldrich | Cat# D9564 | 1:50,000 of a 10 mg/mL stock solution |
other | Citifluor AF1 | Electron Microscopy Sciences | Cat #17970–100 | |
antibody | Anti-pH3 (Rabbit polyclonal) | EMDMillipore - Sigma | Cat# 06-570RRID:AB_310177 | IF 1:1,000 |
antibody | Anti-pH3 (Mouse monoclonal) | EMDMillipore - Sigma | Cat# 05-806RRID:AB_310016 | IF 1:1,000 |
antibody | anti-β-Galactosidase (Rabbit polyclonal) | Invitrogen | Cat# A11132RRID:AB_221539 | IF 1:1,000 |
antibody | Anti-Prospero (Mouse monoclonal) | DSHB | Cat# MR1ARRID:AB_528440 | IF 1:100 |
antibody | Anti-Mesh (Rabbit polyclonal) | Gift from Mikio Furuse, Izumi et al., 2012 | 995-1RRID:AB_2568117 | IF 1:2000 |
antibody | Anti-peIF2α (Rabbit monoclonal) | Cell signaling technologies | Cat# 3398RRID:AB_2096481 | IF 1:500 |
antibody | Anti-puromycin (mouse monoclonal) | DHSB | Cat# PMY-2A4RRID:AB_2619605 | IF 1:100 |
antibody | Anti-mouse Alexa 555 (Donkey polyclonal) | Thermo Fisher | Cat# A31570RRID:AB_2536180 | IF 1:2000 |
antibody | Anti-rabbit Alexa 555 (Donkey polyclonal) | Thermo Fisher | Cat# A31572RRID:AB_162543 | IF 1:2000 |
antibody | Anti-mouse Alexa 647 (Donkey polyclonal) | Thermo Fisher | Cat# A31571RRID:AB_162542 | IF 1:2000 |
antibody | Anti-rabbit Alexa 647 (Donkey polyclonal) | Thermo Fisher | Cat# A31573RRID:AB_2536183 | IF 1:2000 |
commercial assay or kit | QuantSeq 30 mRNA-Seq Library Prep Kit FWD | Lexogen | Cat#015.2 × 96 | |
software, algorithm | Fiji | Schindelin et al., 2012 | RRID# SCR_002285 | |
software, algorithm | OsiriX DICOM viewer | Rosset et al., 2004 | RRID# SCR_013618 | |
software, algorithm | Adobe Premiere pro | Adobe | RRID# SCR_021315 | |
software, algorithm | Adobe Photoshop | Adobe | RRID# SCR_014199 | |
software, algorithm | Adobe Illustrator | Adobe | RRID# SCR_010279 | |
software, algorithm | Rstudio | RStudio Team (2020). RStudio: Integrated Development for R. RStudio, PBC, Boston, MA URL http://www.rstudio.com/. | RRID# SCR_000432 | |
software, algorithm | FCS express 6 | De Novo software | RRID:SCR_016431 | https://denovosoftware.com/ |
software, algorithm | FastQC | Babraham Bioinformatics | RRID:SCR_014583 | https://www.bioinformatics.babraham.ac.uk/index.html |
software, algorithm | Cutadapt | Martin, 2011 | RRID:SCR_011841 | |
software, algorithm | TopHat | Trapnell et al., 2009 | RRID:SCR_013035 | https://ccb.jhu.edu/software/tophat/index.shtml |
software, algorithm | HTSeq | Anders et al., 2015 | RRID:SCR_005514 | https://htseq.readthedocs.io/en/master/ |
Additional files
-
Supplementary file 1
Recipes of all diets utilized in this study.
- https://cdn.elifesciences.org/articles/64125/elife-64125-supp1-v2.docx
-
Supplementary file 2
R Markdown.
- https://cdn.elifesciences.org/articles/64125/elife-64125-supp2-v2.zip
-
Transparent reporting form
- https://cdn.elifesciences.org/articles/64125/elife-64125-transrepform1-v2.pdf