NAD+ enhances ribitol and ribose rescue of α-dystroglycan functional glycosylation in human FKRP-mutant myotubes
Figures
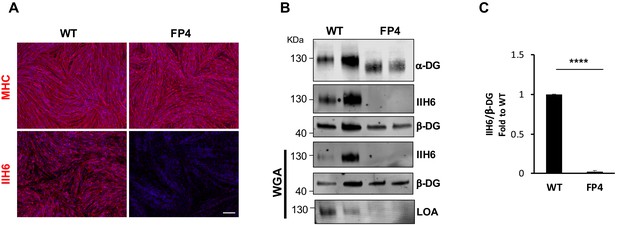
Patient-specific Walker-Warburg syndrome (WWS) induced pluripotent stem (iPS) cell-derived myotubes display reduced functional glycosylation of α-dystroglycan (α-DG).
(A) Representative immunostaining of wild type (WT) and FP4 iPS cell-derived myotubes for myosin heavy chain (MHC) and IIH6 (in red). DAPI stains nuclei (in blue). Scale bar, 200 μm. (B) Representative western blot for α-DG core and α-DG functional glycosylation (IIH6) in WT and FP4 myotubes. β-DG was used as loading control. Lower panel shows wheat germ agglutinin (WGA) pull-down for these samples and representative laminin overlay assay (LOA) of WGA elutes shows laminin detection only in WT samples. (C) Graph bars show respective quantification of IIH6 (B) normalized to β-DG and shown as the fold difference of WT. Error bars represent standard errors of five independent experiments. Significance was evaluated by the unpaired Student’s t test. ****p<0.0001.
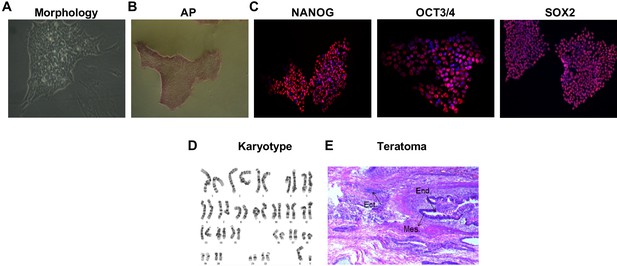
Pluripotency characterization of reprogrammed FP4 induced pluripotent stem (iPS) cell line.
(A–C) Representative images show typical (A) pluripotent colony morphology, (B) alkaline phosphatase activity, and (C) immunostaining for OCT3/4, SOX2, and NANOG (red). DAPI stains nuclei (in blue). Scale bar is 200 μm. (D) Cytogenetic analyses show normal karyotype. (E) Subcutaneous injection of FP4 iPS cells into NOD scid gamma (NSG) mice results in teratoma formation. Representative image shows hematoxylin-eosin staining of a teratoma denoting the presence of tissues derived from all three germ layers.
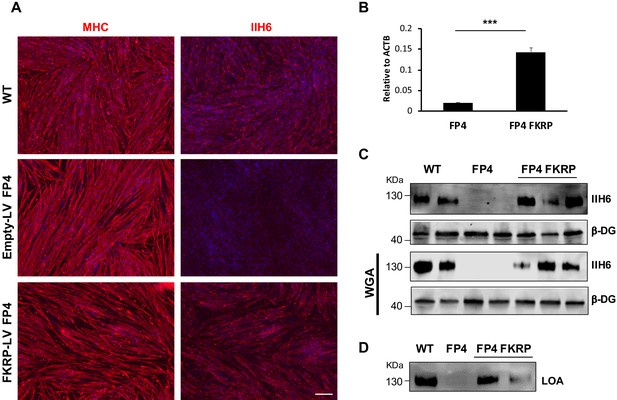
Fukutin-related protein (FKRP) overexpression rescues functional glycosylation of α-dystroglycan (α-DG) in Walker-Warburg syndrome (WWS) FP4 induced pluripotent stem (iPS) cell-derived myotubes.
(A) Representative immunofluorescence staining of control (empty vector LV) and FKRP-LV FP4 iPS cell-derived myotubes for myosin heavy chain (MHC) and IIH6 (in red). Wild type (WT) myotubes were used as reference. DAPI stains nuclei (in blue). Scale bar, 200 μm. (B) RT-qPCR analysis shows increased expression levels of FKRP in FKRP-LV FP4 iPS cell-derived myotubes compared to control. Error bars represent standard errors of three independent experiments. (C–D) Introduction of FKRP rescues impaired functional glycosylation of α-DG in FP4 FKRP myotubes, as shown by western blot for IIH6 (C) and laminin overlay assay (LOA) denoting laminin detection (D). β-DG was used as loading control. Lower panel shows representative wheat germ agglutinin (WGA) pull-down. Significance was evaluated by the unpaired Student’s t test. ***p<0.001.
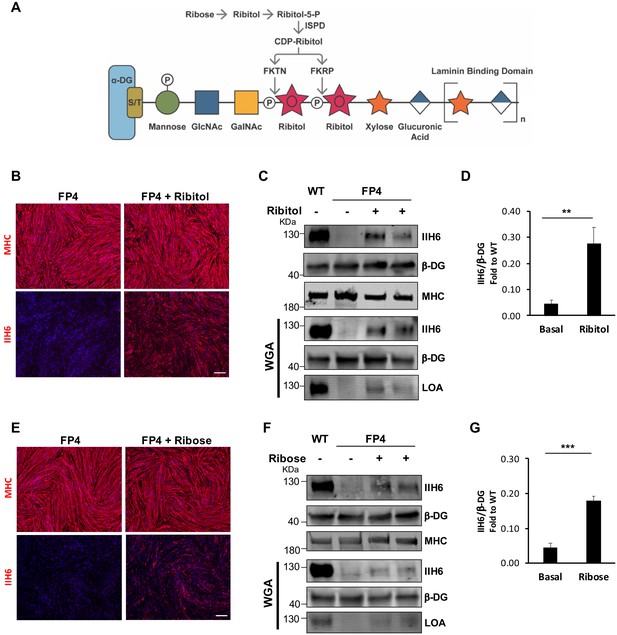
Ribitol and ribose rescue α-dystroglycan (α-DG) functional glycosylation in patient-specific Walker-Warburg syndrome (WWS) myotubes.
(A) Scheme denoting the role of ribose and ribitol in the generation of Rbo5P, which is required by fukutin (FKTN) and fukutin-related protein (FKRP) for glycosylation of α-DG. (B) Representative immunostaining for myosin heavy chain (MHC) and IIH6 (in red) in WWS FP4 induced pluripotent stem (iPS) cell-derived myotubes that had been treated or not with ribitol. DAPI stains nuclei (in blue). Scale bar, 200 μm. (C–D) Western blot shows increased IIH6 staining in WWS FP4 myotubes upon ribitol supplementation. MHC (MF-20) and β-DG were used as differentiation and loading controls, respectively. Lower panel shows wheat germ agglutinin (WGA) pull-down for these samples, and laminin overlay assay (LOA) of WGA elutes shows laminin detection in ribitol-treated FP4 myotubes. Wild type (WT) myotubes were used as positive control. (D) Bar graph shows quantification of IIH6 (from C) normalized to β-DG and shown as the fold difference of WT. Error bars represent standard errors of five independent experiments. (E) Representative immunostaining for MHC and IIH6 (in red) in FP4 iPS cell-derived myotubes that had been treated or not with ribose. DAPI stains nuclei (in blue). Scale bar, 200 μm. (F–G) Western blot for IIH6 in FP4 myotubes that had been treated with ribose. MF-20 and β-DG were used as differentiation and loading controls, respectively. Lower panel shows representative WGA, and LOA shows laminin detection in ribose-treated FP4 myotubes. WT myotubes were used as positive control. (G) Bar graph shows increased IIH6 in ribose-treated myotubes. Quantification of IIH6 (F) was normalized to β-DG and shown as the fold difference of WT. Error bars represent standard errors of five independent experiments. Significance was evaluated by the unpaired Student’s t test. *p<0.05, ***p<0.001.
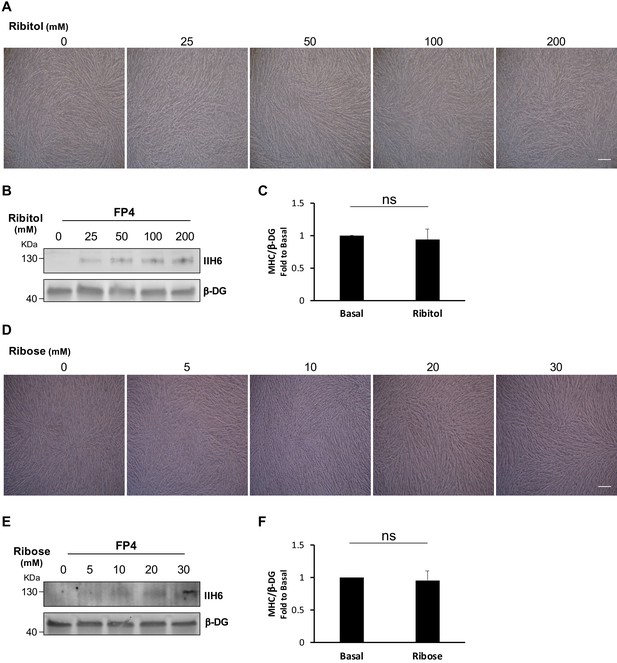
Ribitol and ribose dose-response studies in induced pluripotent stem (iPS) cell-derived myotubes.
(A–B) Phase contrast images of FP4 myogenic progenitors that upon induction of differentiation were treated with the indicated concentrations of ribitol for 5 days. (B) Western blot shows enhanced IIH6 immunoreactivity was observed as ribitol concentration increased. β-Dystroglycan (β-DG) was used as loading control. Scale bar, 200 μm. (C) Bar graph shows quantification of myosin heavy chain (MHC) from ribitol-treated myotubes normalized to β-DG and shown as the fold difference to basal. Error bars represent standard errors of seven independent experiments. (D–E) Phase contrast images of FP4 myogenic progenitors that were differentiated to myotubes and treated with increasing concentrations of ribose for 5 days. (E) Western blot shows IIH6 immunoreactivity. β-DG was used as loading control. Scale bar, 200 μm. (F) Bar graph shows quantification of MHC from ribose-treated myotubes normalized to β-DG and shown as the fold difference to basal. Error bars represent standard errors of seven independent experiments. Significance was evaluated by the unpaired Student’s t test.
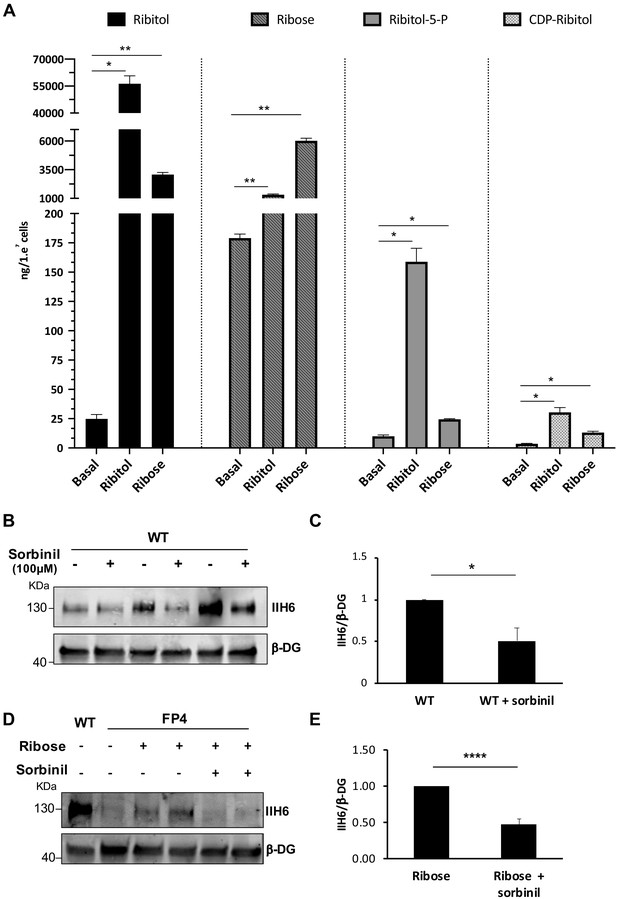
Ribitol and ribose increase the levels of ribitol-5-P and cytidine diphosphate (CDP)-ribitol in patient-specific Walker-Warburg syndrome (WWS) myotubes.
(A) Detection of ribitol, ribose, ribitol-5-P, and CDP-ribitol in WWS FP4 myotubes that had been treated or not with ribitol or ribose for 5 days (n = 3 for each cohort). Error bars represent standard errors of three independent experiments. (B–C) Sorbinil treatment for 5 days results in decreased α-dystroglycan (α-DG) functional glycosylation, as shown by western blot for IIH6 in wild type (WT) myotubes (B). β-DG was used as loading control. (C) Bar graph shows quantification of IIH6 (from B) normalized to β-DG and shown as the fold difference of WT. Error bars represent standard errors of three independent experiments. (D–E) Sorbinil treatment counteracts the positive effect of ribose on α-DG functional glycosylation. (D) Representative western blot shows reduction of IIH6 staining in FP4 myotubes that had been treated with both ribose and sorbinil. β-DG was used as loading control. (E) Bar graph shows quantification of IIH6 (from D) normalized to β-DG and shown as the fold difference of FP4 + ribose. Error bars represent standard errors of five independent experiments. Significance was evaluated by the one-way ANOVA followed by the Sidak’s multiple comparison test in (A) and the unpaired Student’s t test in (C and E). *p<0.05, **p<0.01, ***p<0.001.
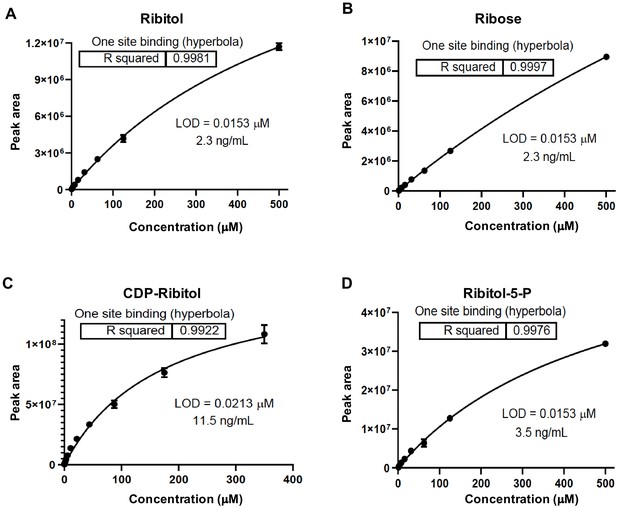
Liquid chromatography with tandem mass spectrometry (LC/MS-MS) standard curves.
(A–D) Standard curves from serial dilution of metabolites (A) ribitol, (B) ribose, (C) ribitol-5-P, and (D) cytidine diphosphate (CDP)-ribitol.
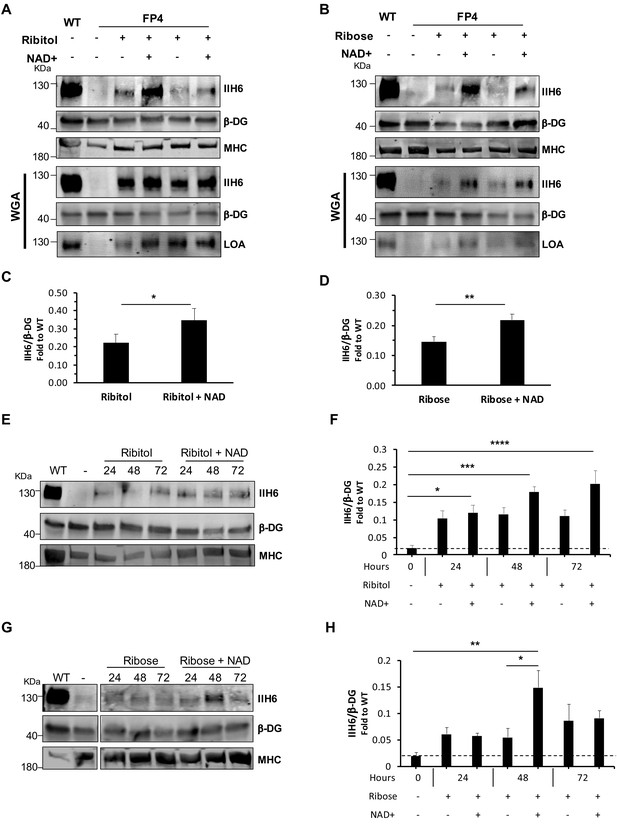
NAD+ potentiates the capacity of ribitol and ribose to rescue α-dystroglycan (α-DG) functional glycosylation.
(A–B) Western blots show increased IIH6 staining in Walker-Warburg syndrome (WWS) FP4 myotubes that had been supplemented with ribitol and NAD+ compared to ribitol alone (A) or with ribose and NAD+ compared to ribose alone (B). Myosin heavy chain (MHC) (MF-20) and β-DG were used as differentiation and loading controls, respectively. Lower panel shows wheat germ agglutinin (WGA) pull-down for these samples, and respective laminin overlay assay (LOA) of elutes shows increased laminin detection in FP4 myotubes that were treated with ribitol/NAD+ or ribose/NAD+. Wild type (WT) myotubes were used as positive control. (C–D) Bar graph shows quantification of IIH6 (A and B, respectively) normalized to β-DG and shown as the fold difference of WT. Error bars represent standard errors of 8 (for C) or 7 (for D) independent experiments. (E–H) Western blot of IIH6 in FP4 D4 differentiated myotubes treated with ribitol and ribitol/NAD+ (E) or ribose and ribose/NAD+ (F) for 24, 48, or 72 hr. MF-20 and β-DG were used as differentiation and loading controls, respectively. (G–H) Bar graph shows quantification of IIH6 (from E and F, respectively) normalized to β-DG and shown as the fold difference of WT. Error bars represent standard errors of four independent experiments. Significance was evaluated by the paired Student’s t test in (C and D) and by the one-way ANOVA followed by the Sidak’s multiple comparison test in (F and H). *p<0.05, **p<0.01, ***p<0.001, ****p<0.0001.
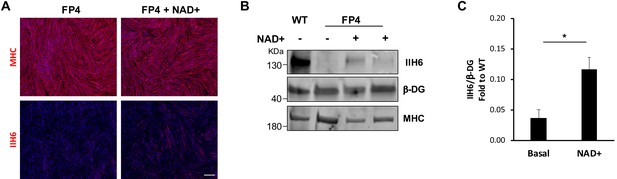
NAD+ rescue functional glycosylation of α-dystroglycan (α-DG) in FP4 induced pluripotent stem (iPS) cell-derived myotubes.
(A) Representative immunostaining of FP4 myotubes treated with NAD+ and respective control wild type (WT) myotubes for myosin heavy chain (MHC) and IIH6 (in red). DAPI stained nuclei (in blue). Scale bar, 200 μm. (B) Representative western blot for IIH6 shows increased immunoreactivity in NAD+ treated FP4 myotubes. MF-20 and β-DG were used as differentiation and loading controls, respectively. (C) Bar graph shows quantification of IIH6 (B) normalized to β-DG and shown as the fold difference of WT. Error bars represent standard errors of six independent experiments. Significance was evaluated by the unpaired Student’s t test. *p<0.05.
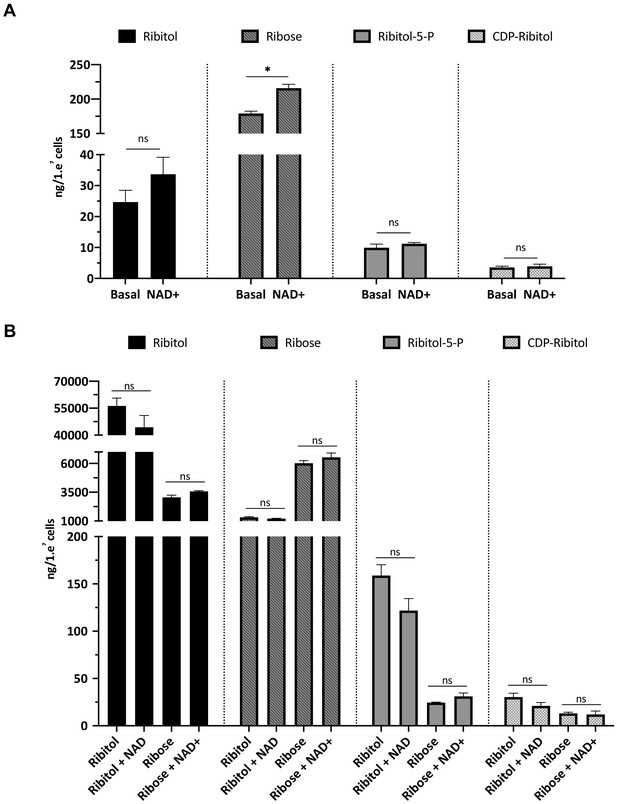
NAD+, ribitol/NAD+, and ribose/NAD+ do not increase ribitol-5-P or cytidine diphosphate (CDP)-ribitol in FP4 myotubes.
(A) Liquid chromatography with tandem mass spectrometry (LC/MS-MS) detection of ribitol, ribose, ribitol-5-P, and cytidine diphosphate (CDP)-ribitol from FP4 myotubes untreated or supplemented with NAD+ for 5 days (n = 3). Error bars represent standard errors of three independent experiments. (B) Detection of ribitol, ribose, ribitol-5-P, and CDP-ribitol from FP4 myotubes supplemented with ribitol, ribose, ribitol/ NAD+, or ribose/NAD+ for 5 days (n = 3 for each cohort). Error bars represent standard errors of three independent experiments. Significance was evaluated by the two-way ANOVA followed by the Sidak’s multiple comparison test (A) and the one-way ANOVA followed by the Sidak’s multiple comparison test in (B). *p<0.05.
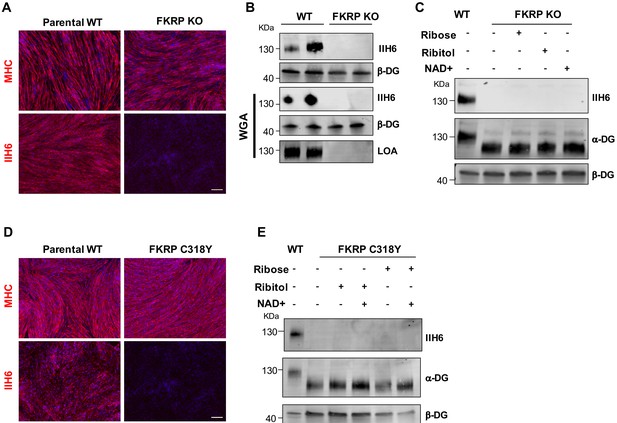
Functional fukutin-related protein (FKRP) is required for NAD+ ribitol/ribose-mediated rescue of α-dystroglycan (α-DG) functional glycosylation.
(A) Representative immunostaining of FKRP knockout (KO) and respective control wild type (WT) isogenic PS cell-derived myotubes for myosin heavy chain (MHC) and IIH6 (in red). DAPI stained nuclei (in blue). Scale bar, 200 μm. (B) Western blot for IIH6 shows absence of α-DG functional glycosylation in FKRP KO myotubes. β-DG was used as loading control. Lower panel shows representative wheat germ agglutinin (WGA) pull-down for these samples and laminin overlay assay (LOA) shows the lack of laminin detection in FKRP KO myotubes. Parental WT myotubes were used as positive control. (C) Deletion of FKRP abolishes ribitol, ribose, and NAD+ mediated rescue of α-DG functional glycosylation, as shown by western blot for IIH6. β-DG was used as loading control. (D) Representative immunostaining of parental WT (WT-2) and FKRP C318Y iPS cell-derived myotubes for MHC (upper panel) and IIH6 (lower panel). DAPI stains nuclei (in blue). Scale bar, 200 μm. (E) FKRP C318Y abolishes ribitol, ribose, and combinations with NAD+ mediated rescue of α-DG functional glycosylation, as shown by western blot for IIH6. β-DG was used as loading control.
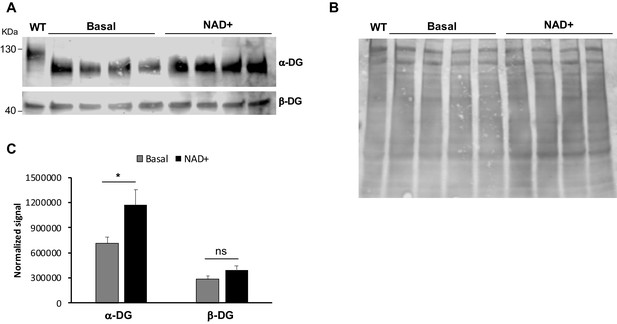
NAD+ treatment in FKRP C318Y myotubes increases α-dystroglycan (α-DG) levels.
(A–B) Western blot for core α-DG and β-DG in FKRP C318Y myotubes treated with NAD+. (B) Total protein stain was used as loading control. (C) Bar graphs shows quantification of α-DG and β-DG normalized to total protein stain. Error bars represent standard errors of eight independent experiments. Significance was evaluated by the unpaired Student’s t test. *p<0.05.
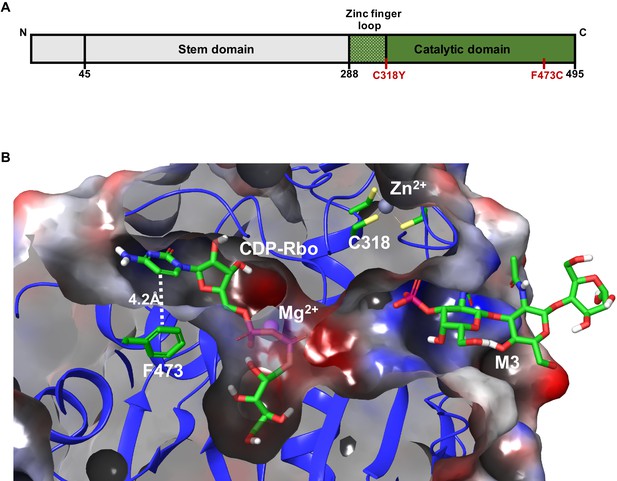
Model depicting the Walker-Warburg syndrome (WWS)-associated mutations in the fukutin-related protein (FKRP) catalytic domain.
(A) Schematic representation of FKRP protein highlighting the N-terminal stem domain and the localization of C318Y and F473C mutations in the C-terminal catalytic domain. (B) Crystal structure of the catalytic domain of FKRP highlights the cytidine diphosphate-ribitol (CDP-Rbo) and α-DG M3 glycan binding sites within its catalytic domain. The FKRP C318Y mutation is one of the four required cysteine residues located in the zinc finger loop. The F473C mutation present in the FP4 patient-specific induced pluripotent stem (iPS) cell line is located adjacent to the pyrimidine ring of CDP-Rbo essential for substrate binding. An electrostatic potential surface highlights the overall cavity size and the electrostatic complementarity between the bound substrates and FKRP, with the neutral, negatively, and positively charged surfaces colored in the white, red, and blue, respectively. The model is based on the PDB:6KAM structure.
Tables
Reagent type (species) or resource | Designation | Source or reference | Identifiers | Additional information |
---|---|---|---|---|
Cell line (Homo sapiens, male) | FP4 | This study | Available from the Anne Bang lab | |
Genetic reagent (Homo sapiens, male) | FKRP C318Y | This study | TC1133 FKRP C318Y | Available from the Rita Perlingeiro lab |
Genetic reagent (Homo sapiens, male) | FKRP KO | This study | H9 FKRP KO | Available from the Rita Perlingeiro lab |
Cell line (Homo sapiens, male) | WT | PMID:22560081 | PLZ | Control line, available from the Rita Perlingeiro lab |
Cell line (Homo sapiens, male) | Parental WT FKRP KO | WiCell | H9 | ESC control line (WA09) |
Cell line (Homo sapiens, male) | Parental WT FKRP C318Y | PMID:26411904 | TC-1133 | Control line, available with RUCDR Infinite Biologics |
Chemical compound, drug | CHIR99021 | Tocris | Cat# 4423 | 10 µM |
Chemical compound, drug | LDN193189 | Cayman chemical | Cat# 19396 | 200 nM |
Chemical compound, drug | SB431542 | Cayman chemical | Cat# 13031 | 10 µM |
Chemical compound, drug | DAPT | Cayman chemical | Cat# 13197 | 10 µM |
Chemical compound, drug | Dexamethasone | Cayman chemical | Cat# 11015 | 10 µM |
Chemical compound, drug | Forskolin | Cayman chemical | Cat# 11018 | 10 µM |
Chemical compound, drug | Ribitol | Sigma-Aldrich | Cat# A5502 | 50 mM |
Chemical compound, drug | D-(−)-ribose | Sigma-Aldrich | Cat# R9629 | 10 mM |
Chemical compound, drug | NAD+ | Sigma-Aldrich | Cat# N0632 | 100 µM |
Chemical compound, drug | Sorbinil | Sigma-Aldrich | Cat# S7701 | 100 μM |
Chemical compound, drug | Doxycycline | Sigma-Aldrich | Cat# D9891 | 1 µg/ml |
Recombinant protein | Recombinant human FGF-basic | Peprotech | Cat# 100-18B | 5 ng/ml |
Antibody | Anti-alpha dystroglycan (mouse monoclonal) | Millipore | Cat# 05–593, RRID:AB_309828 | Dilution 1:1000 (WB) |
Antibody | Anti-alpha dystroglycan (mouse monoclonal) | DSHB | Cat# IIH6 C4, RRID:AB_2617216 | Dilution 1:50 (IF) |
Antibody | Anti-human dystroglycan (sheep polyclonal) | R and D Systems | Cat# AF6868, RRID:AB_10891298 | Dilution 1:1000 (WB) |
Antibody | Anti-MHC (mouse monoclonal) | DSHB | Cat# MF20, RRID: AB_2147781 | Dilution 1:50 (IF) 1:200 (WB) |
Antibody | Anti-laminin (rabbit polyclonal) | Sigma-Aldrich | Cat# L9393, RRID:AB_477163 | Dilution 1:1000 (WB) |
Antibody | Anti-beta dystroglycan, concentrated (mouse monoclonal) | DSHB | Cat# MANDAG2 clone 7D11, RRID:AB_2211772 | Dilution 1:1500 (WB) |
Antibody | Anti-OCT3/4 (mouse monoclonal) | SCBT | Cat# C-10, RRID: AB_628051 | Dilution 1:50 (IF) |
Antibody | Anti-SOX2 (goat polyclonal) | SCBT | Cat# Y-17, RRID: AB_2286684 | Dilution 1:50 (IF) |
Antibody | Anti-NANOG (mouse monoclonal) | SCBT | Cat# H-2, RRID: AB_10918255 | Dilution 1:50 (IF) |
Antibody | Anti-SSEA4 (mouse monoclonal) | SCBT | Cat# sc-21704, RRID: AB_628289 | Dilution 1:50 (IF) |
Antibody | Alexa fluor 555 goat anti-mouse IgG and IgM (goat polyclonal) | Thermo Fisher Scientific | Cat# A-21424, RRID: AB_141780 Cat# A-21042, RRID:AB_2535711 | Dilution 1:500 (IF) |
Antibody | DyLight 680 anti-rabbit IgG (goat polyclonal) | Thermo Fisher Scientific | Cat# 35568, RRID:AB_614946 | Dilution 1:10000 (WB) |
Antibody | DyLight 680 anti-mouse IgM (goat polyclonal) | Thermo Fisher Scientific | Cat# SA5-10154, RRID:AB_2556734 | Dilution 1:10000 (WB) |
Antibody | DyLight 680 anti-sheep IgG (rabbit polyclonal) | Thermo Fisher Scientific | Cat# SA5-10058, RRID:AB_2556638 | Dilution 1:10000 (WB) |
Antibody | DyLight 800 anti-mouse IgG (goat polyclonal) | Thermo Fisher Scientific | Cat# SA5-10176, RRID:AB_2556756 | Dilution 1:10000 (WB) |
Other | DAPI stain | SCBT | sc-3598 | (1.5 µg/ml) |
Other | Laminin from Engelbreth-Holm-Swarm murine sarcoma basement membrane | Millipore Sigma | Cat# L2020 | 1 µg/µl |