In Vivo Models: Visualizing traumatic brain injuries
Traumatic brain injuries are a leading cause of death and disability in younger people, as well as an important risk factor for neurodegenerative diseases and dementia in older adults. They can be caused by direct physical insults, whiplash or shockwaves such as those produced by explosions (Cruz-Haces et al., 2017). Yet traumatic events can also have effects beyond the immediate death and damage to neurons. In particular, they can disrupt a protein known as Tau, which normally maintains the stability of many neuronal cells. When this happens, an abnormal, hyperphosphorylated version of Tau accumulates in cells and spreads throughout the central nervous system by turning healthy Tau proteins into the harmful variant (Johnson et al., 2013; Ojo et al., 2016; Zanier et al., 2018). This accumulation is the hallmark of illnesses known as tauopathies, which include Alzheimer’s disease and a progressive brain condition found in athletes who experience regular head blows.
Scientists need accessible animal models in which they can easily observe and manipulate the proliferation of abnormal Tau proteins after a brain trauma. Rat and mouse models exist, but they are expensive and not well suited to visualizing what is happening inside the brain. Now, in eLife, Ted Allison (University of Alberta) and colleagues – including Hadeel Alyenbaawi (Alberta and Majmaah University) as first author and other researchers in Alberta and Pittsburgh – report new zebrafish larvae models for both tauopathies and traumatic brain injuries (Alyenbaawi et al., 2021).
The first model is formed of transgenic, ‘Tau-GFP reporter’ zebrafish in which the spread of the abnormal protein can be directly observed. To achieve this result, Alyenbaawi et al. genetically manipulated the animals so that their neurons would carry a reporter version of Tau that is fused with a green fluorescent protein (or GFP). As the larvae are transparent, their nervous system and the fluorescent Tau are easily visible. The fish were then injected with abnormal mice Tau proteins, causing the reporter Tau to aggregate into mobile ‘puncta’ – small dots which are a hallmark of tauopathies. More puncta were observed when extracts from brains with Tau-linked conditions were injected into the larvae, rather than the normal proteins.
Alyenbaawi et al. also devised a simple and inexpensive zebrafish model for traumatic brain injury. They put the larvae inside a closed syringe, and dropped a weight onto the plunger, creating a shockwave to mimic blast injuries in humans. Three days in a row of this regimen creates conditions reminiscent of those faced in repetitive sports injury. In the Tau-GFP reporter larvae, the shockwave treatment led to fluorescent puncta in the brain and spinal cord, consistent with traumatic brain injuries leading to Tau pathologies (Figure 1). Similarly, past reports have shown that healthy mice developed a Tau-linked condition when they received brain extracts from conspecifics that experienced traumatic brain injuries (Zanier et al., 2018).
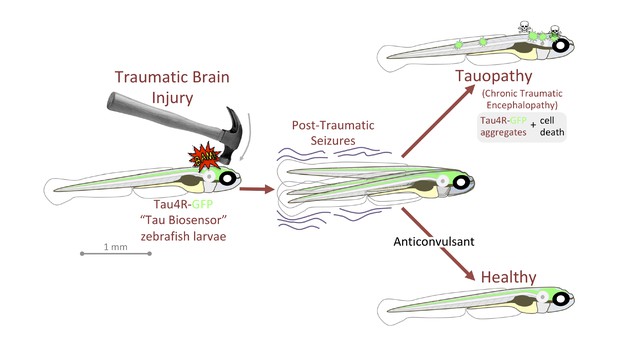
Traumatic brain injury results in seizures and Tau-linked conditions in zebrafish larvae.
Zebrafish larvae with neurons that carry Tau proteins fused with a fluorescent reporter (Tau4R-GFP) are subjected to a brain injury (left). Many then experience seizures, and without treatment they develop a Tau-linked condition in which the proteins aggregate and the neurons die (top right). Larvae that receive anticonvulsants are protected to a certain extent against seizures and the Tau-linked illness (bottom right).
Image credit: Figure based on figure 7 of Alyenbaawi et al., 2021 (CCBY 4.0).
In humans, epileptic seizures appear in over half of traumatic brain injury victims, and especially in those who have received a blast injury; these episodes may initiate or exacerbate the progression of Tau-linked conditions. In zebrafish, the traumatic brain injury larvae also developed seizure-like behaviors, with the intensity of the seizures being positively correlated to the spread of abnormal Tau. Drugs that promoted or stopped seizures respectively increased or decreased the extent of the Tau-linked condition, suggesting that anticonvulsants could help to manage brain traumas in the clinic (Figure 1).
Alyenbaawi et al. carefully identified the limitations of their models, observing for instance that the Tau-GFP reporter could spread in larvae even when the animals did not receive anomalous Tau proteins. This may result from relatively high levels of the Tau reporter in the transgenic animals, outlining the importance of controlling the expression levels of the transgene.
Apart for a recent model which used ultrasound, very few methods have been available so far to simulate traumatic brain injury in zebrafish (Cho et al., 2020). This was particularly the case for larvae, despite these young animals having a more easily observable central nervous system, a high throughput, and an ethical advantage compared to adults. The models developed by Alyenbaawi and colleagues thus constitute a welcome addition to understand the mechanisms associated with traumatic brain injury.
References
-
Pathological correlations between traumatic brain injury and chronic neurodegenerative diseasesTranslational Neurodegeneration 6:20.https://doi.org/10.1186/s40035-017-0088-2
-
Chronic repetitive mild traumatic brain injury results in reduced cerebral blood flow, axonal injury, gliosis, and increased T-Tau and tau oligomersJournal of Neuropathology & Experimental Neurology 75:636–655.https://doi.org/10.1093/jnen/nlw035
Article and author information
Author details
Publication history
Copyright
© 2021, Ekker
This article is distributed under the terms of the Creative Commons Attribution License, which permits unrestricted use and redistribution provided that the original author and source are credited.
Metrics
-
- 1,335
- views
-
- 111
- downloads
-
- 0
- citations
Views, downloads and citations are aggregated across all versions of this paper published by eLife.
Download links
Downloads (link to download the article as PDF)
Open citations (links to open the citations from this article in various online reference manager services)
Cite this article (links to download the citations from this article in formats compatible with various reference manager tools)
Further reading
-
- Neuroscience
Perceptual systems heavily rely on prior knowledge and predictions to make sense of the environment. Predictions can originate from multiple sources of information, including contextual short-term priors, based on isolated temporal situations, and context-independent long-term priors, arising from extended exposure to statistical regularities. While the effects of short-term predictions on auditory perception have been well-documented, how long-term predictions shape early auditory processing is poorly understood. To address this, we recorded magnetoencephalography data from native speakers of two languages with different word orders (Spanish: functor-initial vs Basque: functor-final) listening to simple sequences of binary sounds alternating in duration with occasional omissions. We hypothesized that, together with contextual transition probabilities, the auditory system uses the characteristic prosodic cues (duration) associated with the native language’s word order as an internal model to generate long-term predictions about incoming non-linguistic sounds. Consistent with our hypothesis, we found that the amplitude of the mismatch negativity elicited by sound omissions varied orthogonally depending on the speaker’s linguistic background and was most pronounced in the left auditory cortex. Importantly, listening to binary sounds alternating in pitch instead of duration did not yield group differences, confirming that the above results were driven by the hypothesized long-term ‘duration’ prior. These findings show that experience with a given language can shape a fundamental aspect of human perception – the neural processing of rhythmic sounds – and provides direct evidence for a long-term predictive coding system in the auditory cortex that uses auditory schemes learned over a lifetime to process incoming sound sequences.
-
- Cell Biology
- Neuroscience
Reactive astrocytes play critical roles in the occurrence of various neurological diseases such as multiple sclerosis. Activation of astrocytes is often accompanied by a glycolysis-dominant metabolic switch. However, the role and molecular mechanism of metabolic reprogramming in activation of astrocytes have not been clarified. Here, we found that PKM2, a rate-limiting enzyme of glycolysis, displayed nuclear translocation in astrocytes of EAE (experimental autoimmune encephalomyelitis) mice, an animal model of multiple sclerosis. Prevention of PKM2 nuclear import by DASA-58 significantly reduced the activation of mice primary astrocytes, which was observed by decreased proliferation, glycolysis and secretion of inflammatory cytokines. Most importantly, we identified the ubiquitination-mediated regulation of PKM2 nuclear import by ubiquitin ligase TRIM21. TRIM21 interacted with PKM2, promoted its nuclear translocation and stimulated its nuclear activity to phosphorylate STAT3, NF-κB and interact with c-myc. Further single-cell RNA sequencing and immunofluorescence staining demonstrated that TRIM21 expression was upregulated in astrocytes of EAE. TRIM21 overexpressing in mice primary astrocytes enhanced PKM2-dependent glycolysis and proliferation, which could be reversed by DASA-58. Moreover, intracerebroventricular injection of a lentiviral vector to knockdown TRIM21 in astrocytes or intraperitoneal injection of TEPP-46, which inhibit the nuclear translocation of PKM2, effectively decreased disease severity, CNS inflammation and demyelination in EAE. Collectively, our study provides novel insights into the pathological function of nuclear glycolytic enzyme PKM2 and ubiquitination-mediated regulatory mechanism that are involved in astrocyte activation. Targeting this axis may be a potential therapeutic strategy for the treatment of astrocyte-involved neurological disease.