A confinable home-and-rescue gene drive for population modification
Figures
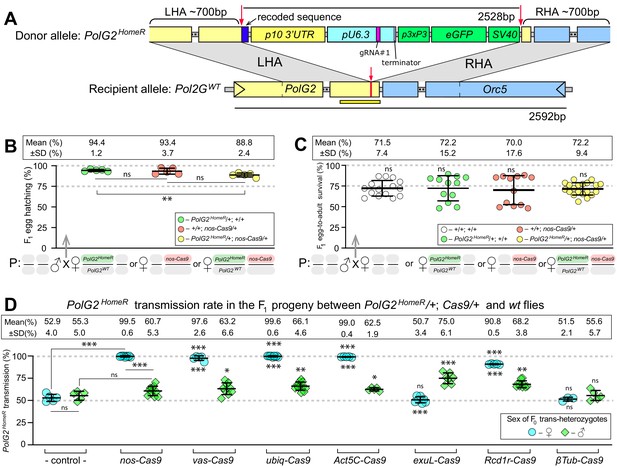
HomeR homes in the presence of Cas9 and biases its transmission in females to nearly 100%.
(A) Schematic maps of the PolG2 donor allele harboring HomeR integrated at the gRNA#1PolG2 cut site (PolG2HomeR, Figure 1—figure supplement 1A), and the recipient wildtype (wt) allele (PolG2WT) encompassing the area spanning PolG2 and Orc5 (CG7833) genes. To facilitate site-specific integration, the HomeR element is surrounded by the left and right homology arms (LHA and RHA) from the Cas9/guideRNA (Cas9/gRNA) cut site (red arrows and lines) in the wt allele. The re-coded 3’ end sequence of PolG2 is shown in dark blue. The yellow line under the PolG2WT recipient allele depicts the location of the C-terminal domain (Figure 1—figure supplement 1A). (B) Embryonic lethality of PolG2WT alleles cannot result in the nearly 100% transmission of PolG2HomeR. (C) The egg-to-adult survival rate indicates that the developmental lethality of PolG2WT alleles also cannot account for the preferential transmission of PolG2HomeR. Therefore, the homing of PolG2HomeR into PolG2WT alleles causes the super-Mendelian transmission of HomeR. (D) PolG2HomeR supports super-Mendelian transmission in conjunction with different Cas9 transgenes and/or maternal carryover of Cas9 protein (Figure 1—figure supplement 3). Trans-heterozygous females (♀) and males (♂) harboring paternal Cas9 expressed under different promoters were mated to wt flies of the opposite sex, and F1 progeny were scored for the GFP dominant marker of PolG2HomeR. The transmission rate was compared to that in PolG2HomeR/PolG2WT; +/+ flies without Cas9 (control) of the corresponding sex (statistical significance indicated above data points). In addition, the transmission rate by trans-heterozygous females was compared to that of trans-heterozygous males for each Cas9 promoter (statistical significance indicated below data points). Notably, while PolG2HomeR can bias its transmission in both sexes, the highest transmission rate is achieved in Drosophila females: 99.6 ± 0.6% in ♀ vs. 75.0 ± 6.1% in ♂. Plots show the mean ± SD over at least five biological replicates. Statistical significance was estimated using a two-sided Student’s t test with equal variance (p ≥ 0.05ns, p<0.05*, p<0.01**, and p<0.001***).
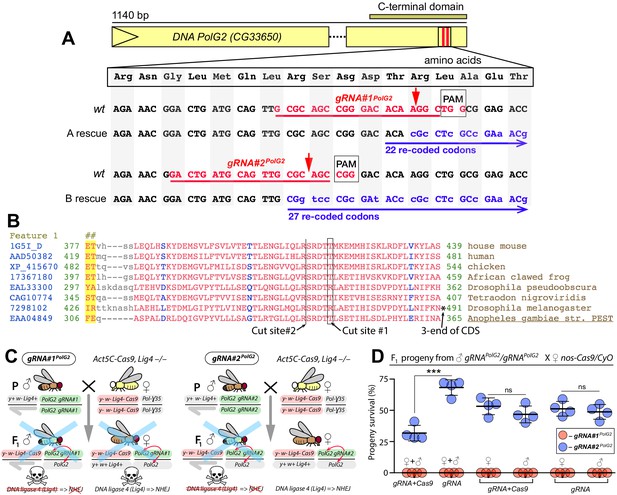
Assessing gRNAs for Cas9-mediated cleavage of PolG2.
(A) Schematic map of the DNA polymerase gamma two gene (PolG2). Two gRNAs targeting its C-terminal domain were chosen and tested for guiding Cas9 cleavage. Both gRNA target sites (highlighted in red) are located near the 3’ end of the coding sequence (CDS, B), facilitating re-coding for the rescue allele (highlighted in blue), resistant to Cas9/guideRNA (Cas9/gRNA)-mediated cleavage. Red arrows depict Cas9/gRNA cut sites, which are 13 bases apart. (B) The amino acid sequence alignments of the 3’ end part of the domain (cd02426; Lu et al., 2020) of PolG2 CDS. (C) Genetic crosses to assess the Cas9-mediated cleavage of two gRNAs targeting PolG2 in the DNA ligase four null genetic background (Lig4–/–), in which the non-homologous end-joining (NHEJ) pathway is reduced. Homozygous gRNA#1PolG2 or gRNA#2PolG2 males were crossed to homozygous Act5C-Cas9, Lig4 –/– females, resulting in death of all male progeny for each gRNA. Notably, female progeny harboring gRNA#1PolG2 and Act5C-Cas9 in the Lig4+/– background also perished. (D) The gRNA#1PolG2, but not gRNA#2PolG2, induced lethality of all F1 progeny in conjunction with nos-Cas9 or maternal carryover of the Cas9 protein via lethal biallelic mosaicism (LBM; Kandul et al., 2019). The gRNA#2PolG2 also guided Cas9-mediated lethality in a fraction of trans-heterozygous male and females gRNA#2PolG2/+; nos-Cas9/+ progeny as compared to that in gRNA#2PolG2/+; +/+. The nos-Cas9/gRNA#2PolG2-dependent lethality affected both sexes equally. The plot shows the mean ± standard deviation (SD) over four biological replicates. Statistical significance was estimated using a two-sided Student’s t test with equal variance (p ≥ 0.05ns and p<0.001***). Data depicted in C and D can be found in Supplementary file 2 and Supplementary file 3, respectively.
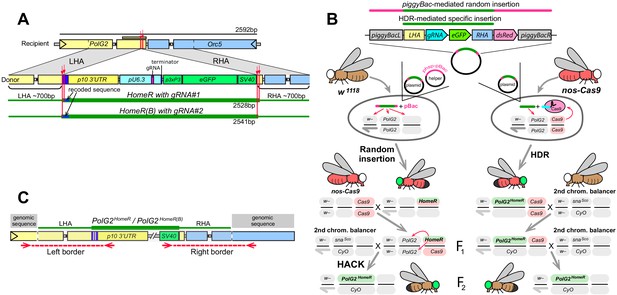
Site-specific integration of HomeR constructs at the PolG2 locus.
(A) Schematic maps of the recipient (wildtype, wt) allele encompassing the area spanning PolG2 and Orc5 (CG7833) genes, and the donor allele harboring the HomeR or HomeR(B) gene drive element site-specifically integrated at the corresponding cut site. Each of HomeR and HomeR(B) contains one gRNA (Figure 1A) and is engineered to be integrated precisely into a specific cut site mediated by the gRNA it harbors via homology-directed repair (HDR). (B) Two separate approaches were used to generate transgenic lines harboring site-specific insertions of HomeR or HomeR(B) at the Drosophila PolG2. In the first approach, two plasmids, one carrying the HomeR construct and the helper plasmid (phsp-pBac; Handler and Harrell, 1999) carrying the piggyBac transposase, were injected into w1118 embryos to generate transgenic lines harboring a random piggyBac-mediated integration tagged by double fluorescence (GFP+ and dsRed+). Then transgenic males (GFP+, dsRed+) were crossed to nos-Cas9 females (dsRed+) to generate site-specific PolG2HomeR transgenic lines, tagged by GFP alone, using Homology Assisted CRISPR Knock-in (HACK; Lin and Potter, 2016). In the second approach, the plasmid harboring the HomeR genetic construct was injected alone into nos-Cas9 embryos (dsRed+) and site-specifically integrated via HDR to generate F1 heterozygous PolG2HomeR/+; nos-Cas9/+ males with double fluorescence (GFP+ and dsRed+). In both approaches, F1 transgenic males harboring PolG2HomeR (GFP+) and nos-cas9 (dsRed+) were crossed to the second chromosome balancer line, w1118; CyO/snaSco, to balance and isolate the PolG2GDe insertion (GFP+). While both techniques (random insertion/HACK and HDR) worked to generate site-directed insertions, all subsequent analyses were performed exclusively on lines derived from the HDR approach. (C) To confirm site-specific integration of both PolG2HomeR and PolG2HomeR(B), the left and right borders between either HomeR or HomeR(B) genetic construct and Drosophila genomic sequence around the integration site were sequenced.
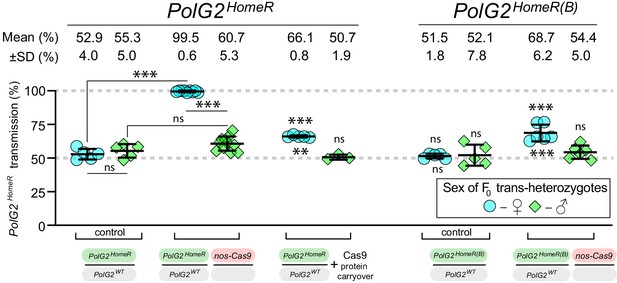
The gRNA efficiency to guide Cas9-mediated cleavage limits the transmission rate for PolG2HomeR.
PolG2HomeR and PolG2HomeR(B) share the nearly identical sequence except for 20 nucleotides of the gRNA they carry: gRNA#1PolG2 and gRNA#2PolG2, respectively (Figure 1—figure supplement 2A). Both PolG2HomeR and PolG2HomeR(B) support super-Mendelian transmission in conjunction with nos-Cas9 in females but not in males. However, PolG2HomeR induced significantly higher transmission than PolG2HomeR(B), 99.5 ± 0.6% vs. 68.7 ± 6.2%, respectively; even maternal carryover of Cas9 protein was sufficient to bias transmission of PolG2HomeR. The high transmission rate of PolG2HomeR accords with the more efficient Cas9-mediated disruption of PolG2 by gRNA#1PolG2 (Figure 1—figure supplement 1C–D). Trans-heterozygous females (♀) and males (♂) harboring paternal nos-Cas9 were mated to wildtype (wt) flies of the opposite sex, and F1 progeny were scored for the GFP dominant marker of PolG2HomeR. The transmission rate was compared to that in the corresponding heterozygous (PolG2HomeR/+ or PolG2HomeR/+) flies (statistical significance indicated above data points). In addition, the transmission rate by trans-heterozygous females was compared to that of trans-heterozygous males (statistical significance indicated below data points). Plots show the mean ± SD over at least three biological replicates. Statistical significance was estimated using a two-sided Student’s t test with equal variance (p ≥ 0.05ns, p<0.05*, p<0.01**, and p<0.001***).
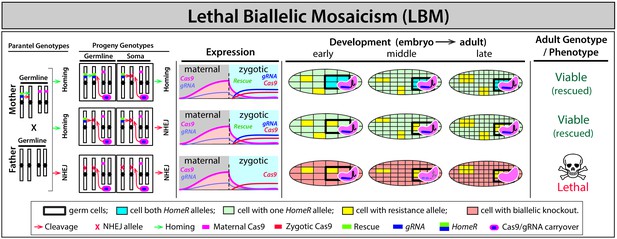
Mechanism of lethal biallelic mosaicism (LBM).
Maternal carryover of Cas9/guideRNA (Cas9/gRNA) complexes contributes to RNA-guided dominant biallelic disruption (both paternal and maternal alleles) of an essential target gene throughout development thereby ensuring recessive non-functional resistant alleles result in dominant deleterious/lethal mutations that can get negatively selected out of a population (Kandul et al., 2019). Individuals that inherit the HomeR drive are protected from LBM through expression of a dominant re-coded protected copy of the haplosufficient essential target gene (rescue) and are therefore viable/fertile, while individuals that inherit two disrupted alleles are dead (i.e. target gene is recessive lethal).
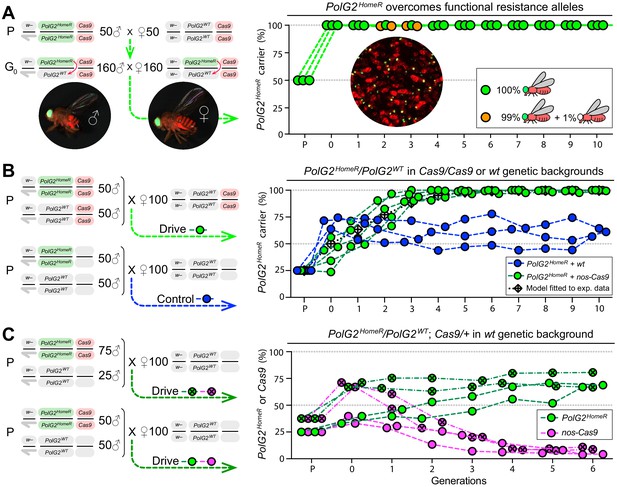
Induced resistant alleles do not impede the Cas9-dependent spread of PolG2HomeR.
(A) To explore the fate of induced functional resistant alleles (R1), three population cages were seeded with PolG2HomeR/PolG2WT heterozygous flies in nos-Cas9/nos-Cas9 genetic background and run for 10 discrete generations. PolG2HomeR and nos-Cas9 were tagged by dominant eye-specific GFP and body-specific dsRed, respectively. Images of an individual male (♂), female (♀), and a group of flies are shown. In total, nine PolG2HomeR-negative flies, as determined by the absence of eye-specific GFP, were identified at generations 2 and 3 in populations #1 and #3. After these flies participated in seeding the next generation, they were isolated and genotyped. Two functional PolG2R1 resistant alleles identified in these flies (Figure 2—figure supplement 1A) were not sampled in flies collected at generation 10 by Illumina amplicon sequencing (Figure 2—figure supplement 1B). (B) The PolG2HomeR allele spread efficiently in the homozygous Cas9 genetic background. Population drives were seeded with 50 PolG2HomeR/PolG2HomeR ♂, 50 wt ♂, and 100 wt virgin ♀ in the presence (green points) or absence (blue points) of nos-Cas9, and the carrier frequency of PolG2HomeR was scored at each discrete generation. The model for the HomeR population replacement drive (gray diamonds and a black dotted line) was fitted to the empirical data of the PolG2HomeR spread in the presence of nos-Cas9 (green points). After 10 generations, the PolG2HomeR allele spread from the introductory frequency of 25% to the carrier frequency of 99.9 ± 0.3% in the presence of nos-Cas (green points) or continued to drift at 60.8 ± 3.8% without the Cas9 transgene (blue points; p<0.0001, a two-sided Student’s t test with equal variance). (C) The invasion of PolG2HomeR is limited by the fitness of the Cas9 transgene. Double homozygous males at frequencies of 75% or 50% were released into the wt genetic background to establish four drive populations with mixtures of trans-heterozygous and wt flies at above or below 50%, respectively, in generation 0. Both PolG2HomeR and nos-Cas9 transgenes were scored at each generation by the GFP (green points) and dsRed (purple points) markers. The carrier frequency of nos-Cas9 decreased from 69.1% to 9.9% or from 36.4% to 4.0% in six generations confining the spread of PolG2HomeR.
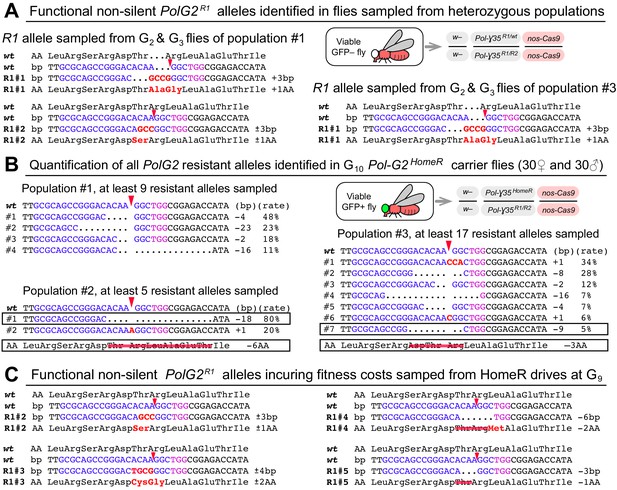
PolG2 resistant alleles induced by PolG2HomeR in experimental populations.
(A) Two functional non-silent resistant (R1) alleles were sampled in nine GFP-negative viable flies segregating from the heterozygous PolG2HomeR/PolG2WT; nos-Cas9/nos-Cas9 fly populations at generations 2 and 3. None of the nine GFP-negative flies harbored the PolG2WT allele. Instead, each fly carried at least one functional non-silent resistant allele rescuing the viability of these flies. Both PolG2R1 types changed the amino acid sequence, hence they are referred to as non-silent R1 alleles. Seven out of nine flies were heterozygous, harboring one of the identified PolG2R1 alleles together with an out-of-frame indel (loss-of-function [LOF]) allele. The remaining two GFP-negative flies were likely homozygous for the R1#1 allele, because 10 randomly sequenced clones harbored the same allele. (B) Resistant alleles, persisting for 10 generations of cleavage and homing (Figure 2A), were sampled from 60 flies chosen randomly harboring at least one PolG2HomeR allele and quantified using Illumina sequencing. For each population, nearly 50,000 amplicons of PolG2 alleles (150K total), which did not carry the 2.5 kb insert of the HomeR, were sequenced and used to estimate the minimum number of sampled resistant alleles. Note that both PolG2R1 alleles (R1#1 and R1#2) identified at earlier generations (A) were not sampled after generation 10. Two in-frame resistant alleles (−18 and −9 bp, boxed) resulted in deletions of six or three amino acids and would likely incur fitness costs without the HomeR’s rescue to their carriers. (C) Four functional non-silent PolG2R1 alleles were found to incur fitness costs on female carriers. These alleles were identified in seven GFP– females sampled at generation 9 from drives 4 and 5 (Figure 2B); when crossed to wt males, these females died in 3 days leaving no progeny and were genotyped. Every genotyped female was heterozygous for one of the four in-frame non-silent functional R1 alleles and an out-off-frame indel LOF allele. Interestingly, the sampled GFP– males were fertile, and four genotyped males harbored the same R1#3 allele. Note that one ‘short-lived’ female had the functional non-silent R1#2 allele previously identified in heterozygous population #1 (A). For all presented resistant alleles, the sequence of gRNA#1PolG2 is highlighted in blue, and its PAM sequence is in purple. Red arrows depict Cas9/guideRNA (Cas9/gRNA) cut sites. Base insertions and amino acid changes are in red. Deleted amino acids are crossed by double red lines.
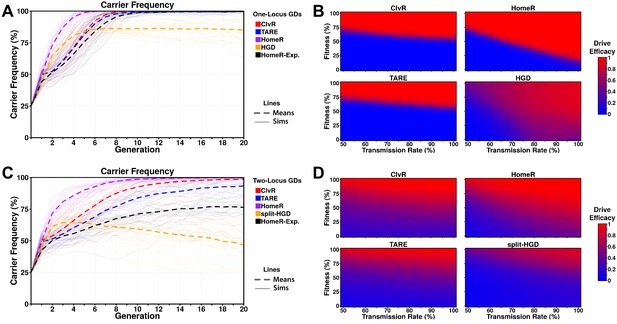
Performance of contemporary gene drive systems for population modification with a single release.
(A) Simulations of carrier frequency trajectories (i.e. heterozygotes and homozygotes) for one-locus versions of ClvR, TARE, HomeR, and HGD for ideal parameters (see 'Materials and methods'), and HomeR for experimental parameters (HomeR-Exp, see 'Materials and methods'). Twenty-five repetitions (lighter lines) were used to calculate the average behavior of each drive (thicker, dashed lines). Populations were initialized with 50% wildtype (+/+) adult females, 25% wildtype (+/+) adult males, and 25% drive homozygous (drive/drive) males. (B) Heatmaps depicting drive efficacy for one-locus versions of ClvR, TARE, HomeR, and HGD for a range of fitness and transmission rate parameter values. Fitness costs were incorporated as a dominant, female-specific fecundity reduction. Transmission rate was varied based on cleavage rate, using HDR rates consistent with ideal parameters, when applicable (see 'Materials and methods'). Drive efficacy is defined as the average carrier frequency at generation 20 (approximately 1 year, given a generation period of 2-3 weeks) based on 100 stochastic simulations with the same initial conditions as (A). (C) Simulations of carrier frequency trajectories for two-locus (split-drive) versions of ClvR, TARE, HomeR, and HGD for ideal parameters (see 'Materials and methods'), and HomeR for experimental parameters (HomeR-Exp, see 'Materials and methods'). Twenty-five repetitions (lighter lines) were used to calculate the average behavior of each drive (thicker, dashed lines). Populations were initialized with 50% wildtype (+/+; +/+) adult females, 25% wildtype (+/+; +/+) males, and 25% drive homozygous (Cas9/Cas9; gRNA/gRNA) males. (D) Heatmaps depicting drive efficacy for two-locus versions of ClvR, TARE, HomeR, and HGD for a range of fitness and transmission rate parameter values, implemented as in panel (B), with initial conditions given in (C).
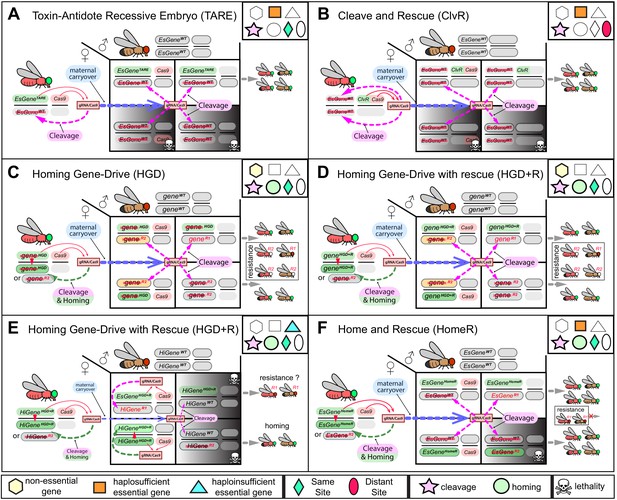
Mechanistic comparison of contemporary split-drives for population modification.
Each diagram depicts the cross between females trans-heterozygous for a split gene drive (GD) and wildtype (wt) males. A two-locus toxin-antidote recessive embryo (TARE) (A) and two-locus cleave and rescue (ClvR) (B) are non-homing toxin-antidote (TA)-based drives. TARE and ClvR force their inheritance by disrupting an essential gene (EsGeneWT) in oocytes as well as in embryos using maternal carryover of Cas9/guideRNA (Cas9/gRNA), and rescuing only those embryos that inherit the drive element harboring the re-coded essential target gene, which is resistant to Cas9/gRNA-mediated cleavage. As a result, mating between trans-heterozygous females and wt males generates 50% non-viable embryos. The TARE is integrated at the target gene locus (referred to as same-site teal diamond) and uses its native promoter to drive expression of the re-coded rescue, hence it is referred to as EsGeneTARE. Panel (B) depicts the two-locus version of ClvR, in which a ClvR harbors a re-coded rescue using a non-native promoter and 3’ UTR, and both ClvR and Cas9 are inserted into two distant loci separate from the target gene (referred to as distant-site red ellipse). Since both TARE and ClvR use multiple gRNAs to target an essential gene, only very rare functional resistant alleles (R1) can survive. (C) A homing-based gene drive (HGD) with no rescue targeting a non-essential gene (gene) can bias transmission in germ cells by cleaving a non-essential gene (gene) and homing, or copying itself, at the cut site (geneGD). However, since the disruption of a non-essential gene does not cause lethality and sterility, maternal carryover of Cas9/gRNA disrupts paternal alleles in embryos, and end joining (EJ) induces both R2 and R1 (geneR2 and geneR1) resistant alleles that survive and eventually hinder the spread of HGD in a population. (D) The HGD with a rescue (HGD+R) targeting a non-essential gene can preserve the function of the target gene and possibly reduce fitness effects. However, given that the gene is non-essential, resistant alleles can accumulate and impede the spread of HGD+R. (E) A homing gene drive with HGD+R targeting a haploinsufficient gene (HiGene) requires two functional alleles for viability and fertility of its carriers. Therefore, any somatic cleavage that does not result in precise homing during development of the trans-heterozygous individuals can induce high fitness costs via lethal biallelic mosaicism (LBM). Maternal carryover and somatic expression of Cas9/gRNA are known to promote generation of resistant alleles and will increase fitness costs of HiGeneHGD+R. Ideally this type of drive system needs to function exclusively in the male germline and avoid problematic maternal deposition issues. (F) Home-and-rescue (HomeR) drives as a toxin-antidote homing drive designed to target a haplosufficient essential gene. HomeR harbors a re-coded essential gene, and its precise homing at the cut site rescues the wt function of the essential gene (EsGeneHomeR). Maternal carryover of HomeR’s Cas9/gRNA induces cleavage of paternal EsGeneWT alleles in embryos, which are rescued by only EsGeneHomeR but not EsGeneR2 maternal alleles resulting in the removal of non-rescued LOF resistant alleles (EsGeneR2) via LBM (see Figure 1—figure supplement 4). HomeR targets an essential gene to increase transmission and impair the survival of resistant alleles that disrupt the function of the target gene (R2). Red strikethrough defines an LOF (R2) allele.
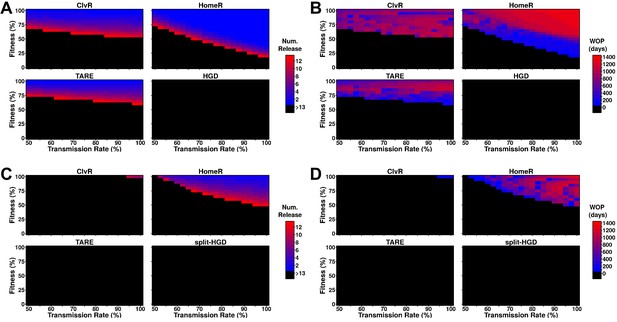
Performance of contemporary gene drive systems for population modification with multiple releases.
(A) Simulations of one-locus designs of ClvR, TARE, HomeR, and HGD in an ecologically consistent model (see 'Materials and methods'). Weekly releases of drive homozygous males (20% of the population size) were performed for up to 13 weeks (3 months, approximately one field season), and the female population was then tested for carrier frequency above 95% at any point within the subsequent 4 years. (B) Same setup as (A), but now the female populations were measured for how many days the carrier frequency remained above 95%, starting at the first release, lasting up to 4 years. This indicates the window where a disease-refractory allele could provide protection (window-of-protection [WOP]). (C) Simulations of two-locus (split-drive) versions of ClvR, TARE, HomeR, and HGD in an ecologically consistent model (see 'Materials and methods'). This time, only the first release was homozygous for Cas9 and the gene drive. Supplementary releases included only Cas9. Male releases were 20% of the total population size, and the female population was measured for drive carrier frequency, not Cas9 frequency, above 95%. (D) Using the data from (C), we applied the method from (B) to measure the WOP, where the drive carrier frequency remained above 95% in the female population. This only measures the drive frequency, not females who carry the Cas9 allele. All simulations contained 100 stochastic repetitions.
Tables
Reagent type (species) or resource | Designation | Source or reference | Identifiers | Additional information |
---|---|---|---|---|
Strain, strain background (D. melanogaster) | gRNA#1PolG2 | 159674 | 91378 | This publication |
Strain, strain background (D. melanogaster) | gRNA#2PolG2 | 159675 | n/a | This publication |
Strain, strain background (D. melanogaster) | HomeRPolG2 | 159676 | Gene drives cannot be deposited at BDSC | This publication |
Strain, strain background (D. melanogaster) | HomeR(B)PolG2 | 159677 | Gene drives cannot be deposited at BDSC | This publication |
Strain, strain background (D. melanogaster) | nos-Cas9 | 112685 | 79004 | 30622266 |
Strain, strain background (D. melanogaster) | vas-Cas9 | 112686 | 79005 | 30622266 |
Strain, strain background (D. melanogaster) | Uniq-Cas9 | 112687 | 79006 | 30622266 |
Strain, strain background (D. melanogaster) | Act5C-Cas9 | n/a | 54590 | 25002478 |
Strain, strain background (D. melanogaster) | exuL-Cas9 | 159671 | 91375 | This publication |
Strain, strain background (D. melanogaster) | Rcd1r-Cas9 | 159673 | 91377 | This publication |
Strain, strain background (D. melanogaster) | bTub-Cas9 | 159672 | 91376 | This publication |
Additional files
-
Supplementary file 1
Target sequence of gRNA and primers used in this study.
- https://cdn.elifesciences.org/articles/65939/elife-65939-supp1-v2.docx
-
Supplementary file 2
Cleavage assay of two gRNAsPolG2 with Act5C-Cas9 in the Lig4∆ genetic background.
- https://cdn.elifesciences.org/articles/65939/elife-65939-supp2-v2.xlsx
-
Supplementary file 3
Cleavage assay of two gRNAsPolG2 with nos-Cas9 and Act5C-Cas9.
- https://cdn.elifesciences.org/articles/65939/elife-65939-supp3-v2.xlsx
-
Supplementary file 4
Transmission rate of PolG2HomeR in conjunction with different Cas9 lines.
- https://cdn.elifesciences.org/articles/65939/elife-65939-supp4-v2.xlsx
-
Supplementary file 5
Transmission rate of PolG2HomeR(B) in conjunction with nos-Cas9.
- https://cdn.elifesciences.org/articles/65939/elife-65939-supp5-v2.xlsx
-
Supplementary file 6
Hatching rate of eggs laid by PolG2HomeR /+; nos-Cas9/+ females.
- https://cdn.elifesciences.org/articles/65939/elife-65939-supp6-v2.xlsx
-
Supplementary file 7
Egg-to-adult survival of PolG2HomeR /+; nos-Cas9/+ females’ progeny.
- https://cdn.elifesciences.org/articles/65939/elife-65939-supp7-v2.xlsx
-
Supplementary file 8
Induced resistant alleles in PolG2HomeR /+; nos-Cas9/nos-Cas9 flies over10 generations.
- https://cdn.elifesciences.org/articles/65939/elife-65939-supp8-v2.xlsx
-
Supplementary file 9
Experimental drives of PolG2HomeR in the nos-Cas9/nos-Cas9 homozygous genetic background.
- https://cdn.elifesciences.org/articles/65939/elife-65939-supp9-v2.xlsx
-
Supplementary file 10
Control drives of PolG2HomeR without the Cas9 transgene.
- https://cdn.elifesciences.org/articles/65939/elife-65939-supp10-v2.xlsx
-
Supplementary file 11
Experimental drives of PolG2HomeR in conjunction with nos-Cas9 into the wt genetic background.
- https://cdn.elifesciences.org/articles/65939/elife-65939-supp11-v2.xlsx
-
Transparent reporting form
- https://cdn.elifesciences.org/articles/65939/elife-65939-transrepform-v2.docx