Experimental Evolution: Failure to progress
It is hard to ignore the sense that life has purpose. This idea – known as teleology – is central to religious thinking. However, it is also found in many areas of human culture and scholarship that one might expect to be free from divine influence. These other areas include, somewhat surprisingly, the study of evolution. Look at the March of Progress, for example: in this infamous illustration a knuckle-dragging beast gradually evolves to become an erect intelligent human. Experts agree that this widely parodied image gives the wrong impression, but the feeling that evolution is progressive persists.
Perhaps the problem is the word itself. To evolve originally meant to unroll, implying the roll-out of a predetermined form (Bowler, 1975). Scientists used it to describe the embryonic development of an individual, back when it was thought that every human grew from a homunculus, a complete miniature person contained within sperm, just waiting to 'evolve' (Horder, 2010). By the mid-19th century 'evolution' had evolved to mean not just the developmental changes that occurred in individuals during their lifetimes, but directional changes observed in species across the geological timescales preserved within the fossil record. Early evolutionists, such as Lamarck, proposed teleologies in which living things are innately driven to progressively evolve more advanced adaptations. But Darwinian natural selection works without these vital forces or supernatural design, and it is notable that Darwin himself rarely used the word evolution in reference to his revolutionary theory.
Evolution, in the modern Darwinian sense, is essentially a random process. Mutations are random, but they are also heritable, so those that happen to improve their own transmission (that is, to increase fitness) will spread, resulting in adaptation. This is natural selection. But there is no direction to the process. Consider eyes, organs so complex that they fool some into thinking they must have been designed by a creator. Yet, having finally evolved this magnificent complexity, eyes will quite readily un-evolve again when their owners move into lightless caves, where vision is a useless and expensive liability.
But does natural selection not imply a particular form of progress, in that fitness itself must always increase? Not necessarily. Now, in eLife, Sean Buskirk, Alecia Rokes and Greg Lang report the results of experiments confirming that natural selection can sometimes result in a reduction of fitness (Buskirk et al., 2020).
The researchers, who are based at Lehigh University, allowed populations of yeast cells to evolve for 1000 generations, freezing live samples at regular intervals to create a ‘fossil record’ from which ancestors and descendants could be defrosted and compared. They found that the most evolved generations (those from the end of the experiment) would leave more offspring than intermediate generations (from the middle of the experiments) when both were mixed and allowed to compete directly: that is, their Darwinian fitness had increased. But when mixed with their original ancestors (from the start of the experiments), they were less fit; the original ancestors left more offspring. Yet, the intermediate generations were fitter than the original ancestors. So, while fitness did in fact increase at each step, it did not add up – together, somehow, two increases made a decrease.
To understand why, we need to know that the ancestor yeast cells were host to a common ‘killer’ virus (Figure 1). The virus encodes both a deadly toxin and resistance to that toxin, so yeast cells containing the virus are immune, but the yeast cells without are not. The virus cannot infect new host cells and is only transmitted through the offspring of its hosts. However, there is no benefit to making a toxin if all your competitors are resistant. So, as the virus populations evolved, the ability to make a worthless toxin was lost. And without the toxin, there was no advantage to having resistance to it so, eventually, resistance was also lost in the most evolved generations of yeast cells.
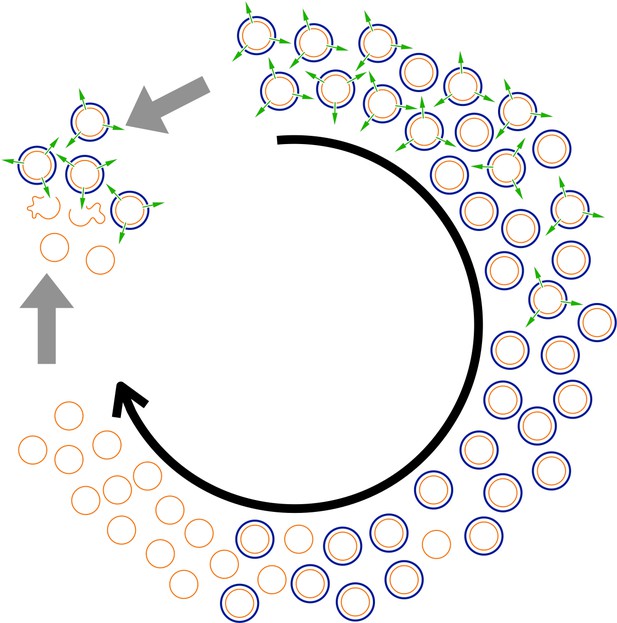
An example of evolution going round in circles.
Buskirk et al. used yeast cells (thin orange circles) that are infected with a virus producing both a killer toxin (green arrows) and resistance to the toxin (thick blue circles) at the start of the experiment (represented by the 12 o’clock position). As evolution proceeds (black arrow), cells that no longer produce the toxin but are still resistant to it, take over. Eventually, these cells are replaced by cells that have lost their resistance (since resistance now provides no benefit). But when cells from the latest generation are pitted against cells from the original generation (grey arrows), the latter emerge victorious as the toxins they produce kill the former (open orange squiggles). However, cells from the latest generation can outcompete cells from intermediate generations, and cells from intermediate generations can outcompete cells from the original generations.
Thus, when cells from these generations were introduced to cells from the original generations, they succumbed to the viral toxin. Buskirk et al. were able to show that natural selection acting on the host genomes, the viral genomes, or both, drove the entire process, eventually reducing the long-term competitive fitness of the yeast. So, evolutionary changes, including fitness, are not necessarily progressive.
Is this due to having two genomes – viral and nuclear – with intertwined fates? Probably not. Take the game rock-paper-scissors as an illustration. An imaginary population of reproductive rocks might evolve into mutant pieces of paper, which would have higher fitness. But once paper has taken over, it would be replaced by descendants that evolved into scissors. Are scissors fitter than their distant ancestors, the rocks? No.
Such circular interactions – where everyone can beat someone, but everyone can also be beaten by someone else – are common in nature, both between and within species (Soliveres et al., 2018; Sinervo and Lively, 1996). But Buskirk et al. show for the first time that the different players can also replace each other within a single evolutionary lineage. We sometimes feel we are making great progress – in art, architecture, fashion, or even in the unfolding of historical events – only to recognize something from the past coming round again. Evolution seems much the same.
References
-
The changing meaning of "evolution"Journal of the History of Ideas 36:95–114.https://doi.org/10.2307/2709013
-
Encyclopedia of Life SciencesHistory of developmental biology, Encyclopedia of Life Sciences, Chichester, John Wiley & Sons, Ltd, 10.1002/9780470015902.a0003080.pub2.
Article and author information
Author details
Publication history
Copyright
© 2021, Greig and Ono
This article is distributed under the terms of the Creative Commons Attribution License, which permits unrestricted use and redistribution provided that the original author and source are credited.
Metrics
-
- 2,393
- views
-
- 98
- downloads
-
- 0
- citations
Views, downloads and citations are aggregated across all versions of this paper published by eLife.
Download links
Downloads (link to download the article as PDF)
Open citations (links to open the citations from this article in various online reference manager services)
Cite this article (links to download the citations from this article in formats compatible with various reference manager tools)
Further reading
-
- Evolutionary Biology
The Xerces Blue (Glaucopsyche xerces) is considered to be the first butterfly to become extinct in historical times. It was notable for its chalky lavender wings with conspicuous white spots on the ventral wings. The last individuals were collected in their restricted habitat, in the dunes near the Presidio military base in San Francisco, in 1941. We sequenced the genomes of four 80- to 100-year-old Xerces Blue, and seven historical and one modern specimens of its closest relative, the Silvery Blue (Glaucopsyche lygdamus). We compared these to a novel annotated genome of the Green-Underside Blue (Glaucopsyche alexis). Phylogenetic relationships inferred from complete mitochondrial genomes indicate that Xerces Blue was a distinct species that diverged from the Silvery Blue lineage at least 850,000 years ago. Using nuclear genomes, both species experienced population growth during the Eemian interglacial period, but the Xerces Blue decreased to a very low effective population size subsequently, a trend opposite to that observed in the Silvery Blue. Runs of homozygosity and deleterious load in the former were significantly greater than in the later, suggesting a higher incidence of inbreeding. These signals of population decline observed in Xerces Blue could be used to identify and monitor other insects threatened by human activities, whose extinction patterns are still not well known.
-
- Evolutionary Biology
Euarthropods are an extremely diverse phylum in the modern, and have been since their origination in the early Palaeozoic. They grow through moulting the exoskeleton (ecdysis) facilitated by breaking along lines of weakness (sutures). Artiopodans, a group that includes trilobites and their non-biomineralizing relatives, dominated arthropod diversity in benthic communities during the Palaeozoic. Most trilobites – a hyperdiverse group of tens of thousands of species - moult by breaking the exoskeleton along cephalic sutures, a strategy that has contributed to their high diversity during the Palaeozoic. However, the recent description of similar sutures in early diverging non-trilobite artiopodans means that it is unclear whether these sutures evolved deep within Artiopoda, or convergently appeared multiple times within the group. Here, we describe new well-preserved material of Acanthomeridion, a putative early diverging artiopodan, including hitherto unknown details of its ventral anatomy and appendages revealed through CT scanning, highlighting additional possible homologous features between the ventral plates of this taxon and trilobite free cheeks. We used three coding strategies treating ventral plates as homologous to trilobite-free cheeks, to trilobite cephalic doublure, or independently derived. If ventral plates are considered homologous to free cheeks, Acanthomeridion is recovered sister to trilobites, however, dorsal ecdysial sutures are still recovered at many places within Artiopoda. If ventral plates are considered homologous to doublure or non-homologous, then Acanthomeridion is not recovered as sister to trilobites, and thus the ventral plates represent a distinct feature to trilobite doublure/free cheeks.