Repurposing eflornithine to treat a patient with a rare ODC1 gain-of-function variant disease
Abstract
Background:
Polyamine levels are intricately controlled by biosynthetic, catabolic enzymes and antizymes. The complexity suggests that minute alterations in levels lead to profound abnormalities. We described the therapeutic course for a rare syndrome diagnosed by whole exome sequencing caused by gain-of-function variants in the C-terminus of ornithine decarboxylase (ODC), characterized by neurological deficits and alopecia.
Methods:
N-acetylputrescine levels with other metabolites were measured using ultra-performance liquid chromatography paired with mass spectrometry and Z-scores established against a reference cohort of 866 children.
Results:
From previous studies and metabolic profiles, eflornithine was identified as potentially beneficial with therapy initiated on FDA approval. Eflornithine normalized polyamine levels without disrupting other pathways. She demonstrated remarkable improvement in both neurological symptoms and cortical architecture. She gained fine motor skills with the capacity to feed herself and sit with support.
Conclusions:
This work highlights the strategy of repurposing drugs to treat a rare disease.
Funding:
No external funding was received for this work.
Introduction
Ornithine decarboxylase (ODC) is a rate-limiting enzyme in the biosynthesis of polyamines (putrescine, spermidine, spermine), which orchestrate essential physiological and pathologic processes including embryogenesis, organogenesis, and neoplastic cell growth (Bello-Fernandez et al., 1993; Pendeville et al., 2001). We recently described a new autosomal dominant genetic disorder (Bachmann-Bupp syndrome, OMIM #619075) caused by a heterozygous de novo variant in the ODC1 gene in a 3-year-old girl with phenotypic features that included alopecia universalis and global developmental delay (Figure 1; Bupp et al., 2018). The nonsense variant caused premature abrogation of 14-aa residues in the C-terminus of the protein (ODC, p·K448X, Figure 2), leading to enhanced function. Red blood cells from the patient exhibited elevated ODC activity and putrescine levels compared to healthy controls. Four additional patients with similar mutations and phenotypic features of this syndrome have since been reported (Rodan et al., 2018) and at least four more cases have been identified.
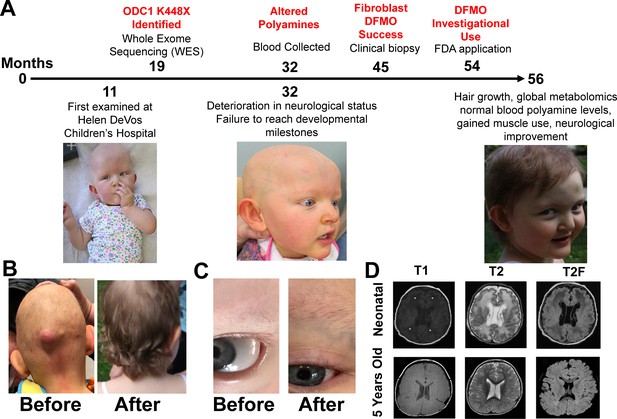
Patient phenotypes and metabolites before and after eflornithine treatment.
Panel A shows the timeline of events for the patient with milestones marked on the top and clinical observations below. Panels B-C show hair growth and muscle tone are the most noticeable phenotype changes with treatment. Follicular cysts recurred on back, neck, and posterior scalp (bottom left images). First hair growth was eyebrows 1 month into treatment (bottom right images). Panel D shows MRI before and after eflornithine treatment. Neonatal: Axial T1 (TR 483 ms, TE 9 ms, and flip angle 63 degrees), T2 (TR 3250 ms, TE 220 ms, and flip angle 90 degrees), and T2-FLAIR (TR 8002 ms, TE 122 ms, and flip angle 90 degrees) show marked abnormal signal of cerebral white matter (*) and several subependymal cysts (arrows). Five years of age: Axial T1 (TR 809 ms, TE 16 ms, and flip angle 111 degrees), T2 (TR 4850 ms, TE 107 ms, and flip angle 142 degrees), and T2-FLAIR (TR 6002 ms, TE 91 ms, and flip angle 90 degrees) show decrease in cerebral white matter volume, but normalization of signal and resolution of subependymal cysts.
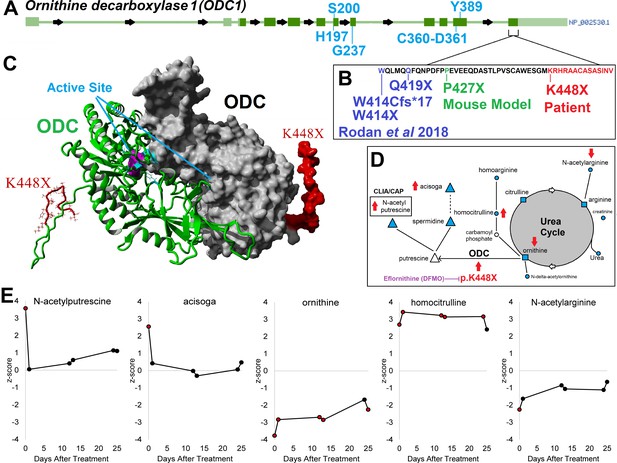
ODC1/ODC clinical variant c.1342 A > T/ p.K448X and support for eflornithine treatment.
Panel A shows the gene structure for ODC1 (ornithine decarboxylase 1) with active site amino acids labeled in blue and the last exon identified. In the last exon cluster, a mouse model variant and four different patient variants including our patient’s K448X variant are described (Panel B, red). The patient variant falls on the disordered C-terminus of ODC, where the two active sites are composed of amino acids from each of two ODC proteins forming a dimer (Panel C). The patient with K448X variant displays alterations of metabolic pathways (Panel D) including polyamines (triangle), urea (square), and others (circle). Metabolites measured are marked in cyan and those altered by K448X with red arrows based on direction of changes seen in the patient. Panel E shows changes in metabolite levels during treatment with eflornithine, with elevated levels of N-acetylputrescine and acisoga decreasing on therapy.
Remarkably, these patients all represent human phenotypes of a transgenic mouse described in 1996, overexpressing C-terminally deleted ODC in the dermal tissue, leading to higher ODC enzyme activity and increased putrescine biosynthesis (Soler et al., 1996). The phenotypic changes first described in a mouse model included hair loss that was reversible with ODC inhibitor α-difluoromethylornithine (DFMO; common name eflornithine) (Soler et al., 1996). Experiments with the patient’s cultured primary dermal fibroblasts showed eflornithine reduced ODC activity, resulting in putrescine levels comparable to controls without affecting cell morphology or inducing cell death (Schultz et al., 2019).
Based on previously published murine data with eflornithine for gain-of-function variants (Soler et al., 1996), multiple long-term safety studies for clinical use in African sleeping sickness (trypanosomiasis), colorectal cancer, and neuroblastoma (Alirol et al., 2013; Priotto et al., 2009; Saulnier Sholler et al., 2015; Meyskens et al., 2008), and the absense of toxicity in the patient’s primary cell culture inresponse to eflornithine (Schultz et al., 2019), we surmised eflornithine might be a novel therapy for patients with this syndrome.
Materials and methods
Reagent type (species) or resource | Designation | Source or reference | Identifiers | Additional information |
---|---|---|---|---|
Gene (Homo sapiens) | ODC1 | NCBI Gene | Gene ID: 4953 | https://www.ncbi.nlm.nih.gov/gene/4953 |
Chemical compound, drug | Eflornithine (DFMO) | Sanofi Aventis | Supplied for study | https://pubchem.ncbi.nlm.nih.gov/compound/Eflornithine |
Biological sample (Homo sapiens) | Blood EDTA tubes | Freshly isolated blood from patient | ||
Software, algorithm | YASARA | YASARA | http://www.yasara.org/ | Protein modelling |
Commercial assay or kit | Liquid chromatography paired massspectrometry | Metabolon, Morrisville, NC | https://www.metabolon.com/ |
Study participants and consent
Request a detailed protocolFollowing FDA approval of our single-patient Investigational New Drug (IND) Application (144022) as a compassionate use treatment protocol, the study was further reviewed and approved by the Spectrum Health Institutional Review Board (IRB). IRB approval for sample collection with informed consent was received to conduct global metabolomics that included, among others, the polyamine metabolites spermidine and N-acetylputrescine.
The patient first presented at Spectrum Health, Helen DeVos Children’s Hospital (Grand Rapids, MI) at 11 months of age (Figure 1A). We diagnosed the ODC C-terminal deletion at age 19 months through whole exome sequencing and characterized the metabolic dyshomeostasis by 32 months (ODC protein and polyamine abnormalities). Eflornithine oral solution was prepared by diluting the lyophilized powder with purified water to a final concentration of 100 mg/mL. At age 4 years and 8 months, we started eflornithine (Sanofi Aventis) treatment with 500 mg/m2/dose bid twice daily via a gastrostomy tube along with a low polyamine diet on November 14, 2019, for 3 months, increasing to 750 mg/m2/dose twice daily, and a final increase to 1000 mg/m2/dose twice daily after 3.5 months. Dosing was based on what had been demonstrated to be safe in pediatric patients in maintenance therapy for neuroblastoma treated with eflornithine (Saulnier Sholler et al., 2015).
Blood collection and processing
Request a detailed protocolEDTA blood tubes collected from the patient were mixed by inversion 8–10 times, centrifuged at 1000×g for 10 min at 4°C to separate plasma (minimum of 0.25 mL, free of hemolysis from red blood cells) from cellular fraction, and both fractions were immediately placed at −80°C. The plasma specimens were coded and anonymized, kept frozen, and shipped in batch to Metabolon, Morrisville, NC, for metabolomics analysis. N-acetylputrescine, the only polyamine that meets CAP/CLIA standards in this analysis, served as the primary indicator of putrescine levels. N-acetylputrescine and additional metabolites meeting CAP/CLIA standards (Figure 2E) and supplemental metabolites were measured in the EDTA plasma samples using ultra-performance liquid chromatography paired with mass spectrometry and Z-scores were calculated for each metabolite against a reference cohort of 866 pediatric patients as described previously (Squitti et al., 2019).
Blood draws for polyamine levels were obtained at initiation of therapy, 1-week post-initiation, immediately prior to each dose increase and then 7 days after each dose increase. These draws were performed in conjunction with safety screening, which included a complete blood count, liver function test, lactate dehydrogenase, complete metabolic panel, calcium, magnesium, and phosphate.
Results
The novel treatment of this ultra-rare (less than 10 known cases) genetic syndrome presented unique challenges for monitoring efficacy over time. Growth parameters and metabolite levels were monitored easily, whereas others such as cognitive and motor functioning proved challenging, making us dependent on her standardized neurological examination.
Eflornithine improves clinical findings
The patient was born with a full head of silver-blond hair similar to a previously described murine phenotype (Soler et al., 1996), which fell out in early months and she remained hairless other than a few scattered, long hairs on the scalp. One month into treatment, hair growth was noted, with eyebrows appearing first (Figure 1B–C). Two months into treatment, scalp hair began to diffusely appear in the normal pattern of hair distribution increasing to resemble normal growth for age (Figure 1). She had a history of recurring follicular cyst formation and enlargement. Multiple lesions located on the posterior scalp and back that first were small maculopapular pustules slowly increased in size to approximately 6–7 cm in diameter (Figure 1B). These lysed spontaneously, but some would enlarge until painful, requiring surgical removal. Upon initiation of eflornithine, the formation of cysts ceased immediately (Figure 1B).
Prior to therapy, she had delayed development which manifested with no standing, cruising, or sitting, and limited fine motor skills. Her BMI increased during eflornithine treatment from 25th percentile to 90th percentile primarily due to increase in weight. This quantitative change was not accompanied by any change in body habitus but rather an increase in muscle bulk. She gained muscle strength demonstrated by acquisition of her ability to hold up her head without support (Figure 1B). As the video file shows after 4 months of eflornithine therapy, she was able to sit unsupported and maintain posture with the physical therapist providing resistance, use a walker, and feed herself with a spoon with some assistance. Video 1 allows for optimal visualization of this rapid improvement of our patient with this gain-of-function mutation in the ODC1 gene. The drastic external change in hair growth, and visible improvement in coordination, attention, and interaction can be clearly seen.
Treatment progression after 4 months of eflornithine therapy.
A neonatal brain MRI showed abnormal cerebral white matter and subependymal cysts (Figure 1D). Repeat MRI done at the end of the 9-month treatment trial with eflornithine demonstrated normalization of the cerebral white matter signal with decrease in volume with white matter loss and resolution of all previously noted cysts. Post-treatment magnetic resonance spectroscopy was also performed showing normalization of the N-acetylaspartate and choline signals relative to creatine Figure 1D.
Eflornithine normalizes metabolomic findings
N-acetylputrescine, the only polyamine metabolite measurement that is CAP/CLIA-certified, was quantified in addition to others using a global metabolomics approach (Figure 2) before and after initiating therapy with eflornithine. Metabolite levels from a reference cohort of 866 pediatric patients were converted into Z-scores, a calculation of standard deviations from the mean of the reference populationthat our patient’s values are compared to. In the global analysis of 915 metabolites of the patient before treatment, a total of 16 had values above the 97.5th percentile and 38 below the 2.5th percentile, with a noted difference in polyamine connected metabolites (Source data 1) without any marked disruption of any other metabolic pathways on treatment. The initial elevation of both N-acetylputrescine as well as the polyamine metabolite N-(3-acetamidopropyl) pyrrolidin-2-one (acisoga), which were above the 97.5th percentile, decreased at initiation of therapy and remained reduced at all time points (Figure 2), indicating that eflornithine treatment had the expected effect. Ornithine and N-acetylarginine were below the 2.5th percentile at start of therapy and normalized to the larger pediatric values over the course of therapy. Urea cycle components citrulline and arginine, along with other metabolites, remained at 1 to −1 standard deviation throughout the study period (Figure 2).
Discussion
The introduction of both whole genome and exome sequencing into clinical practice has led to rare diseases being diagnosed at rates never before seen (Turro et al., 2020; Tarailo-Graovac et al., 2016; Splinter et al., 2018). There are over 6000 rare diseases (incidence of greater than 1 in 2000 people) with over 300 million people worldwide affected (Nguengang Wakap et al., 2020). Though collectively common, each rare disease is unique making it challenging to develop specific therapies.
The process of developing treatment options for these rare diseases starts with a description of the genetic abnormality and developing an understanding of the molecular disruptions downstream from the affected protein. Once the biochemical perturbations are identified, then the quest to identify a drug that will return molecular pathways to normal begins. In genetic diseases, the correct mechanism to adopt could be challenging as many options exist such as activating or repressing pathways, enzyme blockade therapy, gene therapy regimens (Mendell et al., 2017), and potentially circumventing or correcting a genetic mutation such as treatments for muscular dystrophy (Iyer et al., 2019). Taking a genetic defect to human trials requires cell cultures, animal studies, and phased trials to determine safety and efficacy of such therapies. For multiple patients, often with unique genetic variants, to see benefit from this process could take the best part of a decade even as identification of successful drugs has been enhanced by the Orphan Drug Act (Augustine et al., 2013; Griggs et al., 2009).
We show a more rapid strategy of matching a patient with an ultra-rare newly identified syndrome to a drug with subsequent treatment being able to safely correct many phenotypic features. Once the whole exome identified the biochemical pathway, we used data from a previously described transgenic mouse model and our published cell culture study to surmise, eflornithine therapy could be of benefit to the patient. Though experimental data suggested that eflornithine could be beneficial, there is a chasm between ex vivo and in vivo studies with difficulties in extrapolating efficacy or safety from a fibroblast study alone (Schultz et al., 2019). We were fortunate that studies existed for eflornithine in a large enough population to suggest dosage and safety (Alirol et al., 2013; Priotto et al., 2009; Saulnier Sholler et al., 2015; Meyskens et al., 2008).
Once therapy was initiated, some neurological improvement in the patient was noted with better posture, weight gain, reduction, elimination of cyst formation, and significant hair growth. Six months into therapy, she had fine motor capability that she previously lacked, such as the capacity to feed herself and sit with some support. Brain imaging also showed changes that are beyond what would be explained merely by the passage of time, suggesting improvement related to eflornithine treatment.
While COVID-19 restrictions interrupted neurological assessments over the treatment period, the improvements noted throughout the relatively short treatment period of 6 months are truly remarkable, especially given the neurological deterioration in the patient prior to eflornithine therapy. This could be especially consequential if we could initiate therapy in a neonate diagnosed early before neurological damage occurs. We are now aware of other patients identified that present with similar gain-of-function ODC variants and polyamine abnormalities such as elevated N-acetylputrescine (Rodan et al., 2018). The therapy outlined here should allow for replication of the findings with a promise for significant improvement in quality of life for these patients. For such patients we recommend continued monitoring of multiple metabolites including N-acetylputrescine and acisoga to ensure that eflornithine dosing and urea/polyamine metabolite levels stay within normal ranges. The advent of global metabolomics presents a unique opportunity not only to develop a complete understanding of the dyshomeostasis prior to therapy but also a way to appreciate the drug’s impact on interconnected metabolic cycles simultaneously and perhaps a means of identifying disruptions early and predicting adverse effects. This may lead to earlier initiation of therapy in future patients, thereby perhaps avoiding some of the neurological delay that has come to characterize the disease in our patient.
Conclusion
In this study we have laid forth a promising example of going from first publication of a new syndrome to FDA-approved single-patient investigational repurposed drug treatment in 16 months, a methodology and speed rarely seen in the clinical science of rare diseases.
Data availability
Data is provided in Source data 1.
References
-
Clinical trials in rare disease: challenges and opportunitiesJournal of Child Neurology 28:1142–1150.https://doi.org/10.1177/0883073813495959
-
Novel de novo pathogenic variant in the ODC1 gene in a girl with developmental delay, alopecia, and dysmorphic featuresAmerican Journal of Medical Genetics Part A 176:2548–2553.https://doi.org/10.1002/ajmg.a.40523
-
Clinical research for rare disease: Opportunities, challenges, and solutionsMolecular Genetics and Metabolism 96:20–26.https://doi.org/10.1016/j.ymgme.2008.10.003
-
Single-Dose Gene-Replacement Therapy for Spinal Muscular AtrophyNew England Journal of Medicine 377:1713–1722.https://doi.org/10.1056/NEJMoa1706198
-
Estimating cumulative point prevalence of rare diseases: analysis of the Orphanet databaseEuropean Journal of Human Genetics 28:165–173.https://doi.org/10.1038/s41431-019-0508-0
-
The ornithine decarboxylase gene is essential for cell survival during early murine developmentMolecular and Cellular Biology 21:6549–6558.https://doi.org/10.1128/MCB.21.19.6549-6558.2001
-
Gain-of-function variants in the ODC1 gene cause a syndromic neurodevelopmental disorder associated with macrocephaly, alopecia, dysmorphic features, and neuroimaging abnormalitiesAmerican Journal of Medical Genetics Part A 176:2554–2560.https://doi.org/10.1002/ajmg.a.60677
-
Modulation of murine hair follicle function by alterations in ornithine decarboxylase activityJournal of Investigative Dermatology 106:1108–1113.https://doi.org/10.1111/1523-1747.ep12340155
-
Effect of genetic diagnosis on patients with previously undiagnosed diseaseNew England Journal of Medicine 379:2131–2139.https://doi.org/10.1056/NEJMoa1714458
-
Exome sequencing and the management of neurometabolic disordersNew England Journal of Medicine 374:2246–2255.https://doi.org/10.1056/NEJMoa1515792
Article and author information
Author details
Funding
No external funding was received for this work.
Acknowledgements
The authors wish to thank the patient and family for their participation. We would like to dedicate this article to our patient who is a first in so many ways, and to her incredible family. We acknowledge the support we received from the research team at Spectrum Health. We are most grateful to Sanofi-Aventis for providing eflornithine for this study. We also thank Dr B Keith English, MD, Charles Schwartz, PhD, and Brittany Essenmacher for the critical review and editing of this manuscript, David Tack, PhD, for the design of some of the figures, and Olivia Verburg for her help with processing samples and logging samples.
Ethics
Human subjects: FDA approval (IND# 144022) was first acquired before therapy was initiated. The Spectrum Health IRB approved the study (IRB# 2019-161) and informed consent was acquired before blood sample collection. Consent was obtained for use of identifying patient images and videos within this manuscript. After study, authorization for publication was obtained from the parents of the child, now been placed in the medical records.
Copyright
© 2021, Rajasekaran et al.
This article is distributed under the terms of the Creative Commons Attribution License, which permits unrestricted use and redistribution provided that the original author and source are credited.
Metrics
-
- 2,077
- views
-
- 205
- downloads
-
- 17
- citations
Views, downloads and citations are aggregated across all versions of this paper published by eLife.
Download links
Downloads (link to download the article as PDF)
Open citations (links to open the citations from this article in various online reference manager services)
Cite this article (links to download the citations from this article in formats compatible with various reference manager tools)
Further reading
-
- Computational and Systems Biology
- Genetics and Genomics
Apart from ancestry, personal or environmental covariates may contribute to differences in polygenic score (PGS) performance. We analyzed the effects of covariate stratification and interaction on body mass index (BMI) PGS (PGSBMI) across four cohorts of European (N = 491,111) and African (N = 21,612) ancestry. Stratifying on binary covariates and quintiles for continuous covariates, 18/62 covariates had significant and replicable R2 differences among strata. Covariates with the largest differences included age, sex, blood lipids, physical activity, and alcohol consumption, with R2 being nearly double between best- and worst-performing quintiles for certain covariates. Twenty-eight covariates had significant PGSBMI–covariate interaction effects, modifying PGSBMI effects by nearly 20% per standard deviation change. We observed overlap between covariates that had significant R2 differences among strata and interaction effects – across all covariates, their main effects on BMI were correlated with their maximum R2 differences and interaction effects (0.56 and 0.58, respectively), suggesting high-PGSBMI individuals have highest R2 and increase in PGS effect. Using quantile regression, we show the effect of PGSBMI increases as BMI itself increases, and that these differences in effects are directly related to differences in R2 when stratifying by different covariates. Given significant and replicable evidence for context-specific PGSBMI performance and effects, we investigated ways to increase model performance taking into account nonlinear effects. Machine learning models (neural networks) increased relative model R2 (mean 23%) across datasets. Finally, creating PGSBMI directly from GxAge genome-wide association studies effects increased relative R2 by 7.8%. These results demonstrate that certain covariates, especially those most associated with BMI, significantly affect both PGSBMI performance and effects across diverse cohorts and ancestries, and we provide avenues to improve model performance that consider these effects.
-
- Genetics and Genomics
The DYRK1A enzyme is a pivotal contributor to frequent and severe episodes of otitis media in Down syndrome, positioning it as a promising target for therapeutic interventions.