Flagella: A new kind of beat
Many organisms – ranging from single-cell protists to humans – rely on microscopic hair-like structures called cilia and flagella to perform a wide range of roles. In the human respiratory system, for example, large numbers of cilia work ceaselessly to remove dirt and pathogens from the lungs and airways (Sims et al., 2005): sometimes they move backwards and forwards in the plane, and sometimes they follow a more complicated trajectory (Figure 1A). Flagella are typically larger than cilia, and many single-cell organisms rely on the whip-like beating motion of flagella to propel them through the water in which they live.
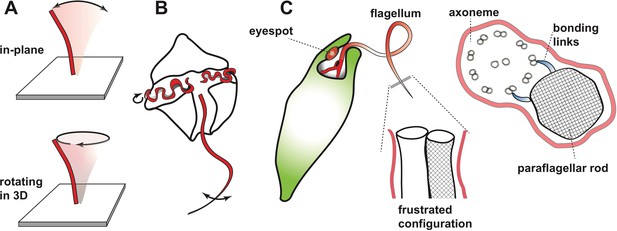
Beating motion of flagella and cilia.
(A) Cilia and flagella stir fluid by moving back and forth in their own plane (top), or in a rotary fashion (bottom). (B) The flagella of single-celled organisms exhibit diverse beat patterns, sometimes on the same cell. For example, dinoflagellates have a transverse flagellum (red) which loops around the cell’s circumference and propagates helicoidal waves, and a longitudinal flagellum which moves back and forth behind the cell. (C) The alga Euglena gracilis has one short flagellum (inside the cell body), and one long flagellum, which contains a core axoneme (white) and a paraflagellar rod (lattice pattern). When the two incompatible structures ‘glue’ together via bonding links (right side image), this creates a mechanical frustration that forces both structures to bend together. As a result, the flagellum bends out of the plane and generates a lasso-like motion which pulls the alga along a helical path.
At the centre of most cilia and flagella is a structure called an axoneme, which consists of two central microtubules inside a circle formed of nine pairs of microtubules. Motor proteins, such as dynein, are able to bind to the microtubules and produce forces that cause the axoneme – and therefore the cilium or flagellum itself – to bend. A combination of mechanical feedback and active signalling coordinates the actions of the motor proteins, and through them, the overal pattern of the beating motion. Different organisms rely on different variations of this basic mechanism, with the greatest variety being found in protists (presumably because one billion years of evolution has provided them with ample opportunity to evolve highly-specialised adaptations to many environmental niches).
Single-celled organisms can have multiple flagella that can differ (sometimes dramatically) in length and/or shape, and even beat at different frequencies (Figure 1B). For example, an alga called Euglena gracilis has two flagella, including one that whirls around like a propeller or lasso in front of the cell and pulls it through the water along a helical path (Leedale et al., 1965). Many protists use this universal strategy to scan their environment (Cortese and Wan, 2021), but the mechanism underlying the lasso-like motion of flagella in Euglena has remained a mystery. Now, in eLife, Giancarlo Cicconofri, Giovanni Noselli and Antonio DeSimone – who are based at the Centre Internacional de Mètodes Numèrics a l’Enginyeria, International School for Advanced Studies (SISSA) and Scuola Superiore Sant’Anna – report how interactions between the axoneme and an unusual structure called the paraflagellar rod may be responsible (Cicconofri et al., 2021). The paraflagellar rod is only found in certain protist groups, where it runs parallel to the axoneme and has a similar diameter (Figure 1C). Moreover, it attaches to specific locations on the axoneme via elastic bonding links, which suggests that it has a role in motility (Melkonian et al., 1982).
Cicconofri et al. developed a detailed mathematical model of the flagellum that replicated the contrasting physical properties of the paraflagellar rod and axoneme. The model showed that when the paraflagellar rod and axoneme ‘glue’ together to form a single structure, the resulting mechanical frustration can cause the axoneme to bend out of plane (Figure 1C). The researchers then go on to show that when dynein motor proteins move in a way that causes a simple planar motion in the absence of the paraflagellar rod, this instead generates a three-dimensional lasso-like motion and helical swimming when the axoneme and paraflagellar rod are glued together. By uncovering a connection between the paraflagellar rod and flagellar motility, Cicconofri et al. may have arrived at a general principle for the generation of complex spatiotemporal dynamics: keeping the underlying control principles simple and harnessing mismatched mechanical properties.
Plants also exploit this approach to achieve surprising levels of speed and agility. For instance, the hair-like awn of Erodium plant seeds are wrapped in stiff cellulose fibres arranged in a tilted helical pattern, which help disperse the seed by uncoiling and coiling the awn in response to humidity (Geer et al., 2020). A similar intimate coupling exists between the internal flagellum and cell body of some spirochaete bacteria, which gives the bacteria their helical shape and determines their movement and virulence (Dombrowski et al., 2009).
Like many other single-celled organisms, Euglena swim along helical paths to scan their local environment (Rossi et al., 2017). In the case of Euglena this is augmented with photoreception, so that the alga can navigate efficiently towards or away from light for photosynthesis. It is possible that the paraflagellar rod can actively deform and alter the beat pattern of the flagellum based on light intensity (Piccinni, 1975), perhaps in combination with a photoreceptor located at its base (Rosati et al., 1991). The mathematical model developed by Cicconofri et al. could be used to test this hypothesis.
Major open questions still remain, such as how and when the paraflagellar rod evolved, and whether it arose in a similar way in different species (Lukes et al., 2009)? For example, the paraflagellar rod is also found in closely-related organisms called kinetoplastids, which includes several parasitic flagellates that cause infectious diseases in humans. Euglena is a useful and versatile model system for answering these questions. The curious features that Euglena and other single-celled organisms evolved over billions of years could be applied to the generation of intelligent materials in soft robotics. This goes to show that no matter whether you are a mathematician or a biologist, nature continues to inspire.
References
-
Control of helical navigation by three-dimensional flagellar beatingPhysical Review Letters 126:088003.https://doi.org/10.1103/PhysRevLett.126.088003
-
The elastic basis for the shape of Borrelia burgdorferiBiophysical Journal 96:4409–4417.https://doi.org/10.1016/j.bpj.2009.02.066
-
Pneumatic coiling actuator inspired by the awns of Erodium cicutariumFrontiers in Robotics and AI 7:17.https://doi.org/10.3389/frobt.2020.00017
-
Structure and physiology of Euglena spirogyra III-VIArchiv für Mikrobiologie 50:133–155.https://doi.org/10.1007/BF00409124
-
Flagellar membrane specializations and their relationship to mastigonemes and microtubules in Euglena gracilisJournal of Cell Science 55:115–135.
-
ATPase activity in flagella from Euglena gracilis localization of the enzyme and effects of detergentsThe Journal of Protozoology 22:331–335.https://doi.org/10.1111/j.1550-7408.1975.tb05182.x
-
Ultrastructure of the apical zone of Euglena gracilis: photoreceptors and motor apparatusElectron Microscopy Reviews 4:319–342.https://doi.org/10.1016/0892-0354(91)90008-Z
Article and author information
Author details
Publication history
- Version of Record published: April 26, 2021 (version 1)
Copyright
© 2021, Wan
This article is distributed under the terms of the Creative Commons Attribution License, which permits unrestricted use and redistribution provided that the original author and source are credited.
Metrics
-
- 2,450
- views
-
- 131
- downloads
-
- 1
- citations
Views, downloads and citations are aggregated across all versions of this paper published by eLife.
Download links
Downloads (link to download the article as PDF)
Open citations (links to open the citations from this article in various online reference manager services)
Cite this article (links to download the citations from this article in formats compatible with various reference manager tools)
Further reading
-
- Developmental Biology
- Physics of Living Systems
Geometric criteria can be used to assess whether cell intercalation is active or passive during the convergent extension of tissue.
-
- Computational and Systems Biology
- Physics of Living Systems
Locomotion is a fundamental behavior of Caenorhabditis elegans (C. elegans). Previous works on kinetic simulations of animals helped researchers understand the physical mechanisms of locomotion and the muscle-controlling principles of neuronal circuits as an actuator part. It has yet to be understood how C. elegans utilizes the frictional forces caused by the tension of its muscles to perform sequenced locomotive behaviors. Here, we present a two-dimensional rigid body chain model for the locomotion of C. elegans by developing Newtonian equations of motion for each body segment of C. elegans. Having accounted for friction-coefficients of the surrounding environment, elastic constants of C. elegans, and its kymogram from experiments, our kinetic model (ElegansBot) reproduced various locomotion of C. elegans such as, but not limited to, forward-backward-(omega turn)-forward locomotion constituting escaping behavior and delta-turn navigation. Additionally, ElegansBot precisely quantified the forces acting on each body segment of C. elegans to allow investigation of the force distribution. This model will facilitate our understanding of the detailed mechanism of various locomotive behaviors at any given friction-coefficients of the surrounding environment. Furthermore, as the model ensures the performance of realistic behavior, it can be used to research actuator-controller interaction between muscles and neuronal circuits.