The neuronal calcium sensor Synaptotagmin-1 and SNARE proteins cooperate to dilate fusion pores
Figures
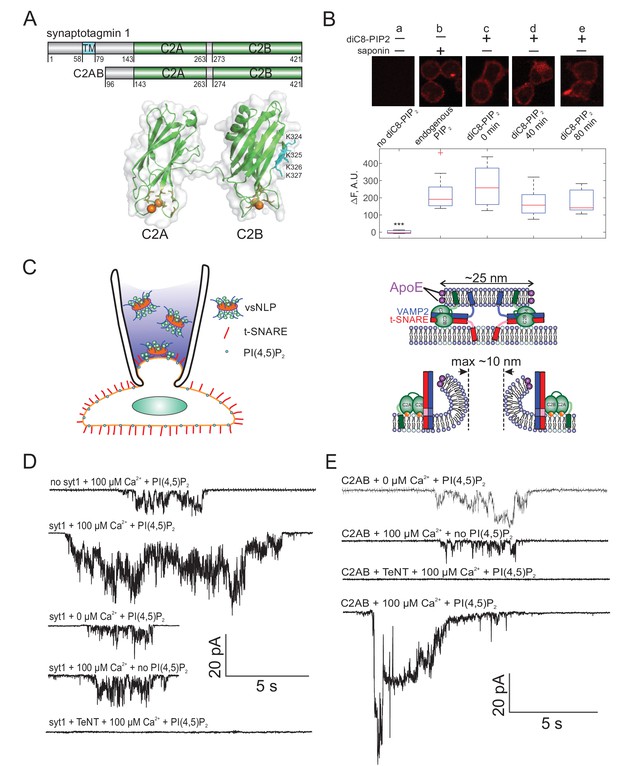
Detection of single fusion pore currents mediated by Syt1 or its C2AB domain.
(A) Domain structures of the constructs used in this study. The structure of the soluble C2AB domains was rendered using PyMol, from PDB: 5kj7 (Lyubimov et al., 2016). The orientations of the C2A and C2B domains relative to each other are not known in the presence of SNAREs and membranes. Conserved aspartate residues coordinating calcium ions are depicted in orange. Calcium ions are shown as orange spheres. A poly-lysine motif on the side of C2B (K324,K325,K326,K327 in the rat sequence) that preferentially interacts with PI(4,5)P2 (Radhakrishnan et al., 2009) is highlighted in cyan. (B) Incorporation of exogenous PI(4,5)P2 into the outer leaflet of flipped t-SNARE cells. Top: cells were incubated with diC8-PI(4,5)P2 for 20 min, rinsed, and immunolabeled for PI(4,5)P2 at the indicated time points. Only control cells that were permeabilized with saponin showed immunostaining, confirming absence of PI(4,5)P2 in the outer leaflet, and providing a reference value for inner-leaflet PI(4,5)P2 levels (a and b). Cells incubated with diC8-PI(4,5)P2 showed immunofluorescence in the absence of permeabilization, indicating successful incorporation of PI(4,5)P2 into the outer leaflet of the cell membrane (c–e). The signal was comparable to endogenous inner-leaflet PI(4,5)P2 levels, and persisted at least for 80 min (lower panel). Cells processed similarly, but not treated with saponin or diC8-PI(4,5)P2 served as negative controls (a). One-way analysis of variance (ANOVA) followed by multiple comparison test was used to compare the signals from the endogenous PI(4,5)P2 sample (b) with all others. *, **, *** indicate p<0.05, 0.01, and 0.001, respectively. (C) Schematic of the single-pore nanodisc-cell fusion assay. A glass micropipette forms a tight seal on a patch of the plasma membrane of a cell expressing ‘flipped’ t-SNARE proteins on its surface. NLPs co-reconstituted with Syt1 and VAMP2 are included in the pipette solution (left). NLP-cell fusion results in a fusion pore connecting the cytosol to the cell’s exterior (right). Under voltage clamp, direct-currents passing through the pore report pore dynamics. With ~25 nm NLPs, the scaffolding ring does not hinder pore expansion up to at least 10 nm diameter. Exogenous PI(4,5)P2 can be added to the cell’s outer leaflet as in B, and calcium in the pipette is controlled using calcium buffers. (D) Representative currents that were recorded during vsNLP-tCell fusion, for the indicated conditions. PI(4,5)P2 indicates cells were pre-treated with diC8-PI(4,5)P2. Tetanus neurotoxin (TeNT) light chain cleaves VAMP2 and blocks exocytosis. Currents were larger when all components were present (SNAREs, Syt1, exogenous PI(4,5)P2 and calcium). (E) Similar to D, but instead of full-length Syt1, 10 μM soluble Syt1 C2AB domains were used together with NLPs carrying ~4 copies of VAMP2 per face.
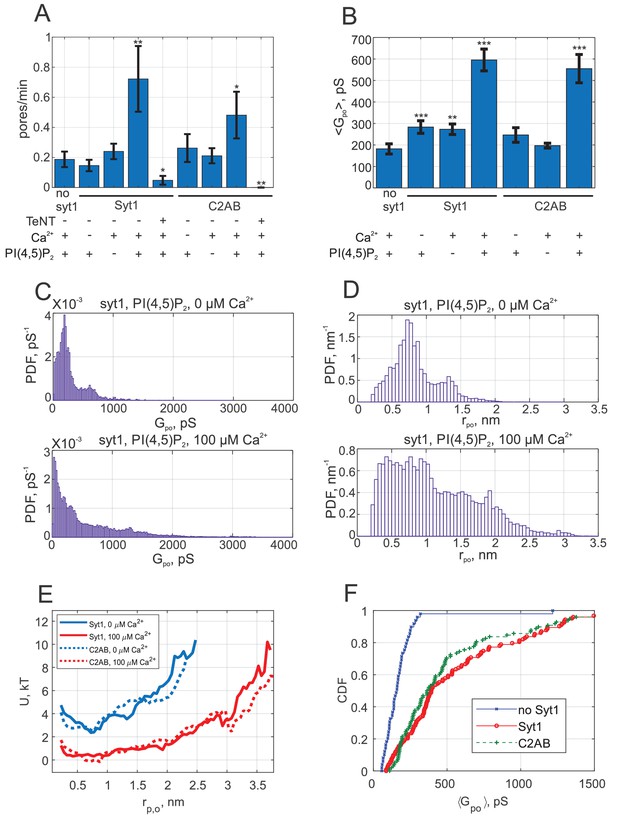
Syt1 promotes fusion and expands fusion pores in a calcium and PI(4,5)P2 dependent manner, and soluble Syt1 C2AB largely recapitulates these effects.
(A) The rate at which current bursts appeared (pore nucleation rate) for the conditions indicated (error bars represent ± S.E.M.). SNARE-induced pores appeared more frequently in the presence of Syt1 or C2AB, when both calcium and PI(4,5)P2 were also present. Student's t-test (one-tailed) was used to assess significant differences between the 'no Syt1' group and the rest. *, **, *** indicate p<0.05, 0.01, and 0.001, respectively. There is no difference between the Syt1 and C2AB groups in the presence of calcium and exogenous PI(4,5)P2 (Student’s t-test: ). (B) Mean single fusion pore conductance, , for different conditions as indicated (± S.E.M.). was three-fold larger in the presence of Syt1 or C2AB, when both calcium and PI(4,5)P2 were also present. Two-sample Kolmogorov-Smirnov test was used to assess significant differences between the 'no Syt1' group and the rest. The same asterisk notation as in A was used. There is no difference between the Syt1 and C2AB groups in the presence of calcium and exogenous PI(4,5)P2 (two-sample Kolmogorov-Smirnov test: ). (C) Probability density functions (PDFs) for point-by-point open-pore conductances (see Materials and methods) for pores induced in the presence of Syt1, PI(4,5)P2 and with 0 or 100 μM calcium. Notice the higher density at larger conductance values in the presence of 100 μM calcium. (D) Probability density functions for pore radii, calculated from the conductance PDFs in C, assuming a 15-nm long cylindrical pore (Hille, 2001). (E) Apparent free energy profiles for Syt1 and soluble Syt1 C2AB domains in the absence or presence of calcium. These profiles were calculated from the pore radii PDFs as in D (see text and Materials and methods) (Wu et al., 2017b). The profiles were shifted vertically for clarity. (F) Cumulative density functions (CDFs) for mean single-pore conductances for the conditions indicated. Soluble C2AB recapitulated effects of full-length Syt1 co-reconstituted into NLPs.
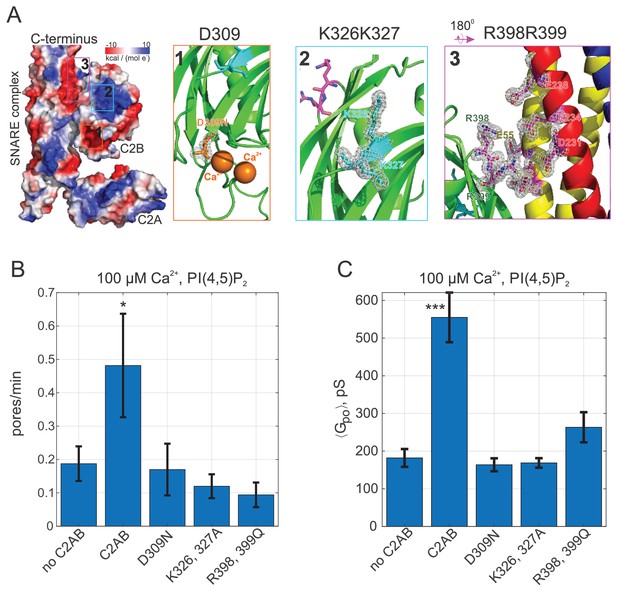
Pore expansion by Syt1 C2AB requires calcium, PI(4,5)P2, and putative SNARE binding sites to be intact.
(A) Overview of the Syt1-SNARE complex (Lyubimov et al., 2016). The electrostatic potential of PDB 5kj7 (Lyubimov et al., 2016) was rendered using Pymol. The sites mutated in this work are marked by boxes labeled 1–3 on the left and shown in the panels to the right. D309 is a key calcium-binding residue (1), K326, K327 interact with acidic lipids (2), and R398,R399 (3) interact with the t-SNAREs SNAP 25 (E51, E52, and E55) and syntaxin 1A (D231, E234, and E238). VAMP2 is shown in blue, SNAP25 in yellow, and syntaxin 1A in red. (B) Pore nucleation rates (+/- SEM) for the indicated conditions. All conditions included 100 μM free calcium and pre-incubation of tCells with exogenous PI(4,5)P2. Pores appeared two to three times less frequently with the mutated proteins compared to wild-type Syt1 C2AB. Student's t-test was used to assess significant differences between the ‘no C2AB’ group and the rest. (C) Mean single open-pore conductance values (± SEM) for the same conditions as in B. Disrupting binding to calcium (D309N), acidic lipids (K326A, K327A), or the SNARE complex (R398, R399) resulted in ~3-fold smaller mean conductance compared to wild-type C2AB, abrogating the effects of Syt1 C2AB. Two-sample Kolmogorov-Smirnov test was used to assess significant differences between the ‘no C2AB’ group and the rest. *, **, *** indicate p<0.05, 0.01, and 0.001, respectively.
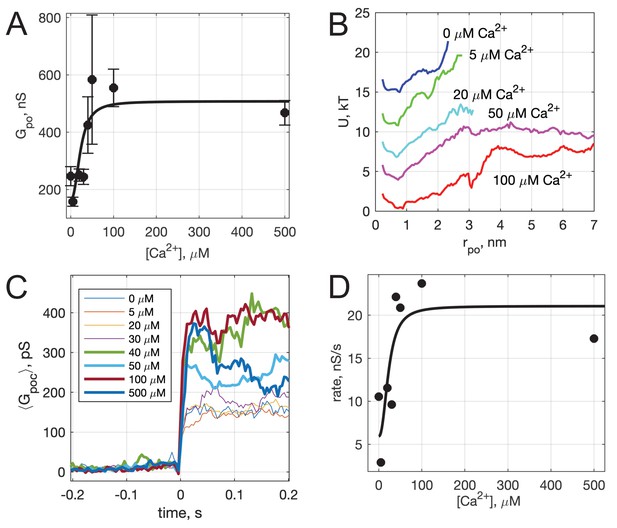
Calcium-dependence of pore properties.
(A) Mean single open-pore conductance, , as a function of free calcium concentration in the pipette solution. Plotted values are mean ± S.E.M. A fit to a Hill equation is shown as the black line, where , , and (see text). Best fit parameters (with 95% confidence bounds) were , and . (B) Apparent free energy profiles, calculated as in Figure 2E, for different calcium concentrations. (C) Kinetics of pore expansion for different [Ca2+]free as indicated. Conductance traces were aligned to the first point in a pore and averaged. (D) Expansion rates of time-aligned and averaged conductances as a function of [Ca2+]free. Expansion rates were calculated as the 10-90% rise time from the baseline to the level of conductance reached within the first 100 ms after pore opening, divided by the time it took for this rise (see Appendix 1, Supplementary Materials and methods). A fit to a Hill equation as in A is also shown, using the same and parameter values.
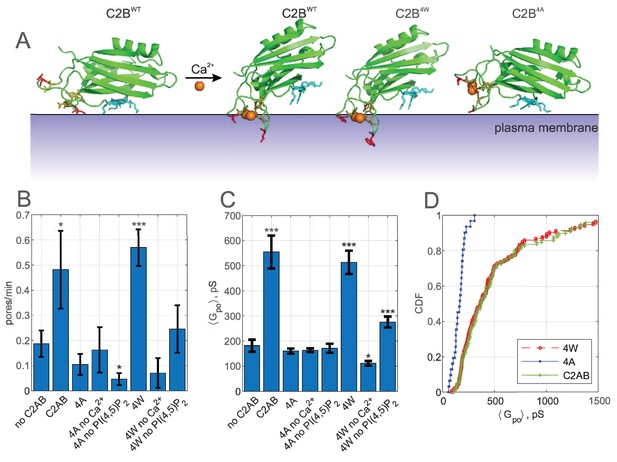
Calcium-induced membrane insertion of Syt1 C2AB hydrophobic loops are critical for both pore nucleation and expansion.
(A) Schematic depiction of Syt1 C2B domain’s calcium-dependent interactions with membranes. Calcium-free C2B interacts with acidic lipids through its poly-lysine motif (highlighted in cyan as in Figure 1). Upon binding to calcium, hydrophobic residues (V304 and I367 on C2B) insert into the membrane, causing C2B to reorient (Chapman, 2008) and inducing membrane curvature (Martens et al., 2007; Hui et al., 2009). In the presence of PI(4,5)P2, the calcium-bound C2B assumes a tilted conformation with respect to the membrane (Kuo et al., 2011). M173 and F234 on C2A top loops similarly insert into membranes in a calcium-dependent manner, with similar effect on orientation and curvature generation (Chapman, 2008) (not shown). A mutant with the membrane-inserting residues replaced with tryptophans (M173W, F234W, V304W, and I367W, ‘4W’) binds membranes more avidly, resulting in more membrane tubulation activity, whereas alanine substitution of the same residues (‘4A’) abolishes membrane penetration and curvature induction (Martens et al., 2007). (B) Pore nucleation rate (mean ± S.E.M) in the presence of wildtype, 4W and 4A mutants. Student's t-test was used to assess significant differences between the ‘no C2AB’ group and the rest. (C) Mean open-pore conductance (± S.E.M) for the conditions indicated. Two-sample Kolmogorov-Smirnov test was used to assess significant differences between the ‘no C2AB’ group and the rest. (D) Cumulative density functions for mean open-pore conductances for wild-type Syt1 C2AB, 4W and 4A mutants. In A, calcium-free C2B was rendered from PDB 5w5d (Zhou et al., 2017) and calcium-bound C2B was rendered from 5kj7 (Lyubimov et al., 2016). *, **, *** indicate p<0.05, 0.01, and 0.001, respectively.
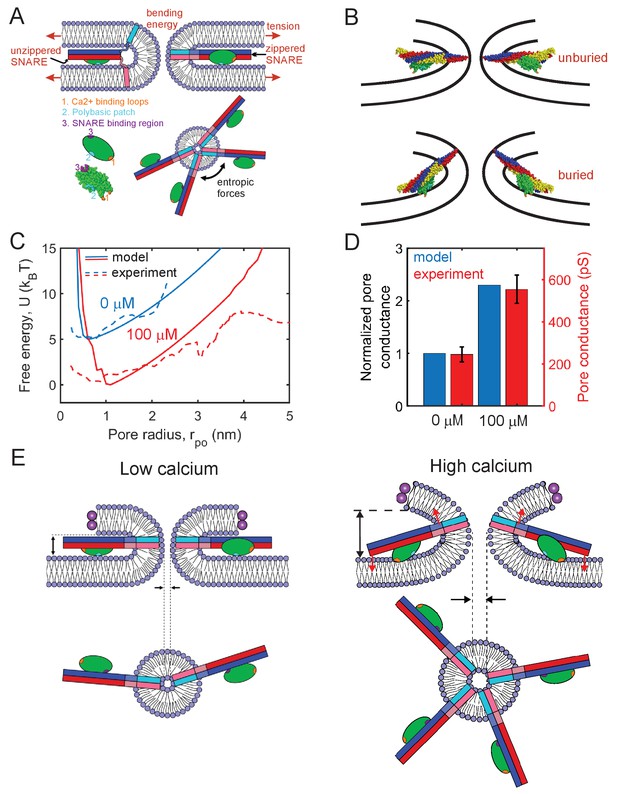
Mathematical model of the fusion pore in the presence of Syt1 and SNAREs suggests a mechanical calcium-triggered pore dilation mechanism.
(A) Schematic of model. The membrane free energy has contributions from membrane tension and bending energy. SNARE complexes may be unzippered and free to roam laterally, or zippered and confined to the pore waist. Crowding among zippered SNARE complexes generates entropic forces that tend to enlarge the pore (top view, shown lower right). The Syt1 C2B domain (green ellipsoid) has a SNARE-binding region, a polybasic patch and Ca2+-binding loops. (B) Free energy-minimizing fusion pore shapes determined by solving the membrane shape equation in the presence and absence of constraints applied by the SNARE-C2B complex (see Appendix 1). The C2B calcium-binding loops may either be unburied (top panel) or buried (lower panel) in the membrane. In the buried state the SNARE complex tilts upwards, expanding the fusion pore. The membrane shape constraint is evaluated using the SNARE-C2B complex crystal structure in a space filling representation. Both upper and lower panels depict situations in the presence of Ca2+. The model predicts the tilted configuration is strongly favored at high following equilibration, while the untilted configuration is relevant to the kinetics that establish this equilibrium, and to experiments using low . VAMP2, syntaxin, SNAP25 and the C2B domain are shown blue, red, yellow, and green, respectively. The C2B hydrophobic membrane-inserting residues (V304, I367), polybasic patch (K326, K327) and SNARE-binding region (R398, R399) are shown orange, cyan, and purple, respectively. The protein structure was generated with PyMOL (Schrodinger, LLC, 2015) using the SNARE-C2B crystal structure (PDB ID 5ccg) (Zhou et al., 2015). The TMD of the SNARE complex (PDB ID 3hd7) (Stein et al., 2009) was incorporated using UCSF chimera software (Pettersen et al., 2004). (C) Model-predicted free energy and experimental apparent free energy versus pore radius without calcium and in the presence of excess calcium. (D) Model-predicted normalized conductances shown with experimentally measured values for comparison. Experimental data taken from Figure 2B experiments including Ca2+ and PI(4,5)P2. (E) Pore dilation mechanism emerging from the model. Under conditions of low calcium concentration, the C2B domain is unburied, the SNARE complex lies parallel to the membrane and the membrane separation is set by the maximum thickness of the SNARE-C2B complex. At high calcium concentrations, the calcium binding loops penetrate the plasma membrane, rotating the C2B domain and the entire SNARE-C2B complex which exerts force (red arrows) on the upper and lower membranes of the fusion pore in a lever-like action. These forces increase the fusion pore height, which is coupled by membrane energetics to fusion pore dilation.
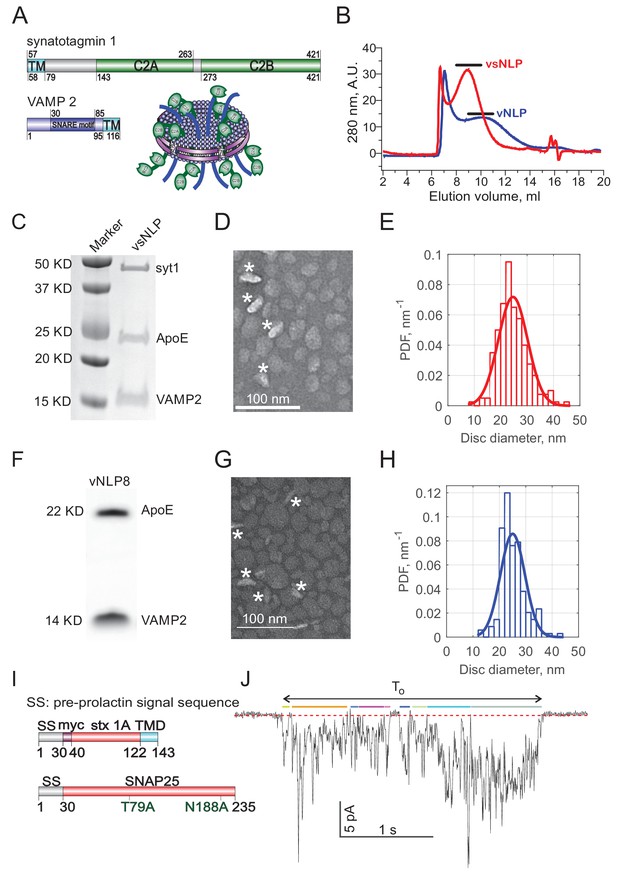
Co-reconstitution of Synaptotagmin-1 and VAMP2 into nanolipoprotein particles (vsNLPs).
(A) Schematic of an NLP reconstituted with 4 copies per face each with Syt1 and VAMP2. Syt1 C2AB domains are shown in green; VAMP2 is shown in blue and the scaffold protein ApoE protein is shown in cyan. Domain structures of Syt1 and VAMP2 are indicated. (B) Typical size-exclusion chromatography elution profiles of vNLP (NLPs loaded with VAMP2 alone) and vsNLP (NLPs loaded with VAMP2 and Syt1) samples using a Superose 6 Increase 10/300 GL column. Proteins were detected using absorbance at 280 nm. Collected eluted volumes are indicated by horizontal bars above each profile. (C) SDS-PAGE stained with Coomassie Brilliant Blue shows the purified NLPs carried Syt1 and VAMP2 proteins. (D) A representative transmission electron microscopy (TEM) image of a vsNLP sample after purification. Nanodiscs indicated by white stars have their lipid bilayer plane positioned perpendicularly to the imaging plane. (E) Distribution of vsNLP sizes from TEM images. More dilute samples (5-10x) than the example shown in D were used for size quantification, such that most NLPs were lying flat on the grid. A Gaussian fit to the distribution is shown as the red solid line (fitted mean diameter = 25±5.6 nm (± SD), n = 200 NLPs). (F–H) Characterization of nanolipoprotein particles reconstituted with VAMP2 alone. (F) SDS-PAGE of purified vNLPs stained with Coomassie Brilliant Blue indicting the NLPs incorporated ApoE and VAMP2 proteins. (G) Negative stain transmission electron microscopy image of a representative vNLP sample. Nanodiscs indicated by white stars are oriented with their disc plane perpendicular to the imaging plane. (H) Size distribution of vNLPs reconstituted with a total of 8 copies of VAMP2 per disc. A Gaussian fit is shown as the red solid line (best fit diameter = 25 ± 4.6 nm (mean ± SD), 171 discs were analyzed). (I) Domain structure of flipped t-SNARE constructs used to generate HeLa cells stably expressing flipped t-SNAREs (Giraudo et al., 2006; Giraudo et al., 2005). (J) Example of a fusion pore current burst and definition of analysis parameters. To be included in the analysis, a current burst must have amplitude >2 pA and last at least 250 ms. Open sub-periods during a burst cross a threshold (-0.25 pA, red dotted line) for at least 60 ms (indicated as the thick colored bars above the current trace). The number of open sub-periods during a burst is equal to the number of pore flickers, . The duration of the burst is the time from the first detected open-pore point until the last one and is denoted . The flicker rate is . The pore open probability is the sum of the open-pore sub-periods (the colored bars) divided by the burst lifetime,.
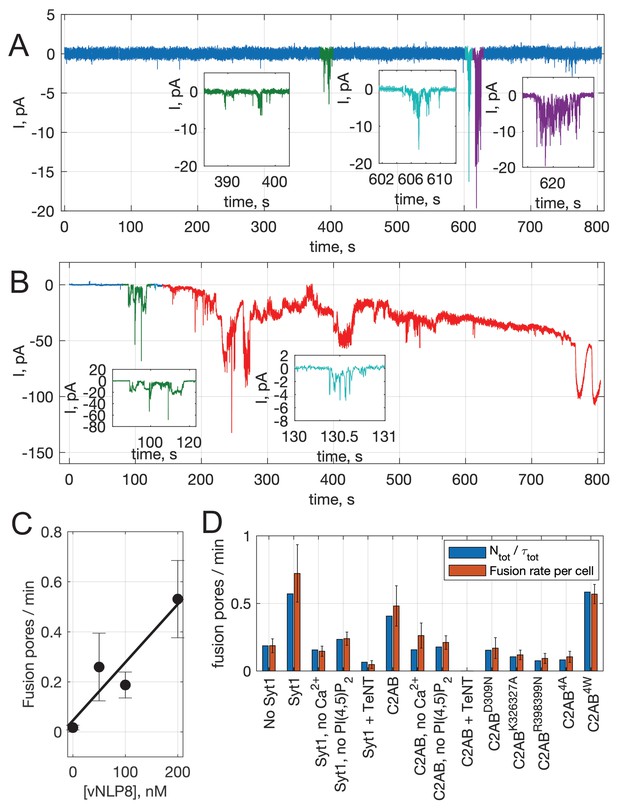
Fusion rates.
(A) An example of an entire 800 s recording that started shortly after establishing a tight seal. The colored regions correspond to currents that are counted as fusion pore currents because they fit the criteria explained in Appendix 1 Detection of pore currents and Estimation of fusion rate (also see Fig. S1J). These regions are shown with expanded axes in insets. In this example, 3 pores were counted in 800 s of recording. (B) Another example of an 800 s recording. In this case, large currents appeared starting ~140 s. The baseline did not recover before the end of the recording and the red-colored portion was excluded from analysis. Thus, two current bursts contributed to the fusion rate from this trace (starting ~90 and 130 s, colored in green and teal), from 140 s of recording. (C) Fusion rate increases with increasing NLP concentration. Fusion rates were calculated as described in Appendix 1 Estimation of fusion rate and plotted against the concentration of NLPs reconstituted with v-SNAREs (8 copies total). Pores from 12-37 cells were recorded for every condition. Error bars indicate S.E.M. The best fit straight line is shown (slope = 2.3 × 10−3 pores/(min•nM), ). (D) Comparison of per cell and overall fusion rates. As an alternative estimate of the fusion rate, we summed all detected pores, , and the analysis time over all cells (excluding portions with noisy/unstable baseline), and calculated the total number of pores divided by the total analysis time, for the indicated conditions. This estimate (blue) is compared with the per cell estimate used throughout (red).
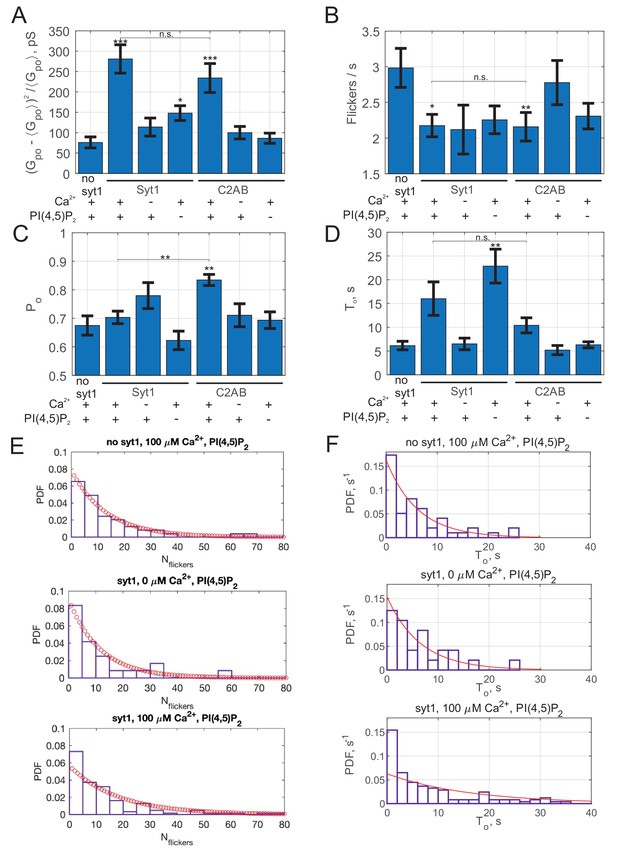
Additional properties of single fusion pores in the presence of full-length Syt1 or soluble C2AB.
Open-pore conductance fluctuations relative to mean (A), average flicker rate during a burst (B), average open-pore probability, , during a current burst (fraction of time pore is in the open state during a burst) (C), and average burst lifetime, , (D) for the indicated conditions. (E) Distributions of the number of flickers per burst, , for the indicated conditions. Fits to geometric distributions are shown in red, . Best fit parameters (with ± 95% confidence intervals) are (no Syt1, 100 μM Ca2+, averaged over 49 individual fusion pores from 10 cells, mean ), 0.083(0.051,0115) (Syt1, 0 μM Ca2+; averaged over 24 individual fusion pores from 11 cells, mean ), 0.053(0.044,0.063) (Syt1, 100 μM Ca2+; averaged over 123 individual fusion pores from 20 cells, mean ). (F) Distribution of burst lifetimes, for the indicated conditions. Best fits to single exponentials are shown as red curves, with means (and 95% confidence intervals) as follows. No Syt1, 100 μM Ca2+: 6.1 s (4.7 to 8.3 s, 49 fusion pores from 10 cells), Syt1, 0 μM Ca2+: 6.5 s (4.5 to 10.1 s, 24 fusion pores from 11 cells), Syt1, 100 μM Ca2+: 16 s (13.5 to 19.3 s, 123 fusion pores from 20 cells). In A-D, the two-sample Kolmogorov-Smirnov test was used to assess significant differences between the "no C2AB" group and the rest. *, **, *** indicate p<0.05, 0.01, and 0.001, respectively. Comparison between Syt1 and C2AB in the presence of Ca2+ and PI(4,5)P2 are also indicated (using the two-sample Kolmogorov-Smirnov test).
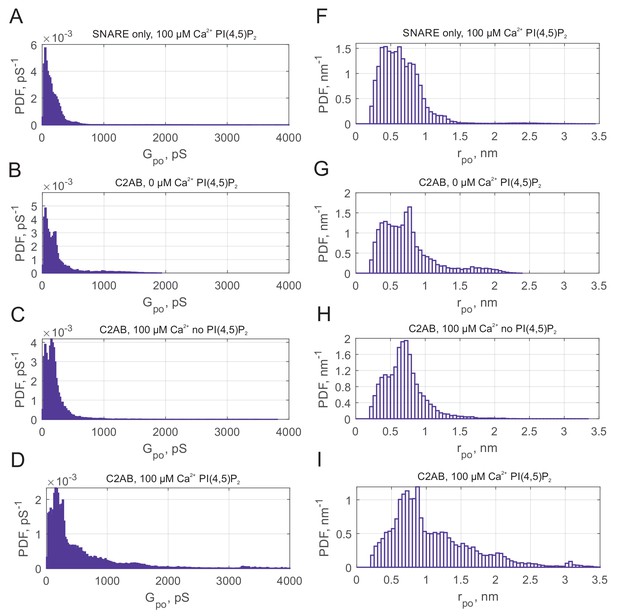
Syt1 C2AB dilates fusion pores in a calcium and PI(4,5)P2 dependent manner.
(A–D) Probability density function (PDF) for point-by-point open-pore conductance values for the indicated conditions. Substantial density is present for pS only when C2AB, calcium, and PI(4,5)P2 were all present. (F–I) PDFs for open-pore radii corresponding to the conductance distributions in A-D, assuming pores are 15 nm long cylinders. Data were from 49 fusion pores/10 cells (SNARE only), 44 fusion pores/12 cells (0 µM Ca2+), 84 fusion pores/19 cells (no PI(4,5)P2) and 98 fusion pores/17 cells (100 µM Ca2+ plus PI(4,5)P2).
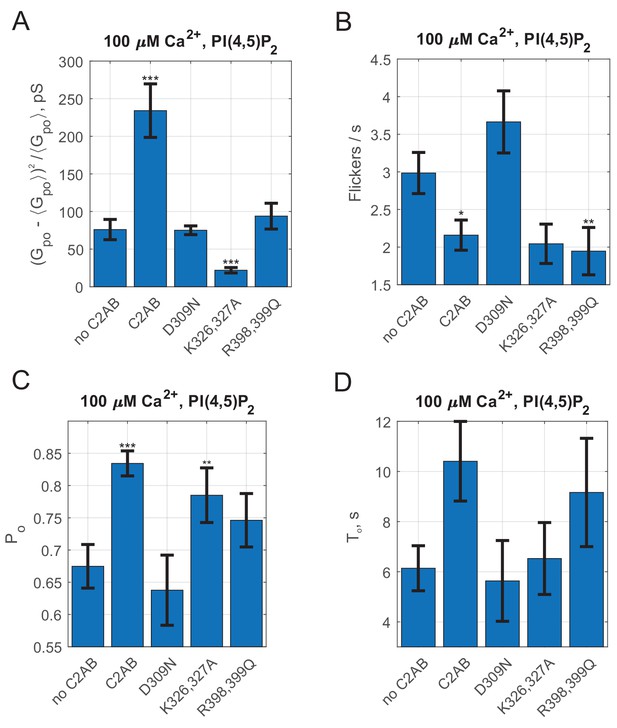
Additional fusion pore properties for Syt1 C2AB domains carrying mutations in D309, K326-327 and R398-399.
(A) Open-pore conductance fluctuations relative to mean. Compared with the SNARE-alone (no C2AB) group, fluctuations were larger for wild-type C2AB, and lower for C2ABK326A,327A. (B) Average flicker rate for the same conditions as in A. Compared with the SNARE alone group (no C2AB), C2AB and C2ABR398Q,R399Q decreased the flicker rate. (C) Average pore open probability during a burst, , for the indicated conditions. Compared with the SNARE alone group (no C2AB), C2AB and C2ABK326A,K327A had larger pore open probabilities. (D) Average burst lifetimes for the same conditions. Error bars are +/- S.E.M. Data were from no C2AB: 49 pores/10 cells, C2AB: 98 pores/17 cells, C2ABK326A,327A: 42 pores/14 cells, C2ABD309N (18 pores/7 cells), C2ABR398Q,R399Q (42 pores/18 cells). For A-D, two-sample Kolmogorov-Smirnov test was used to assess significant differences between the "no C2AB" group and the rest. *, **, *** indicate p<0.05, 0.01, and 0.001, respectively.
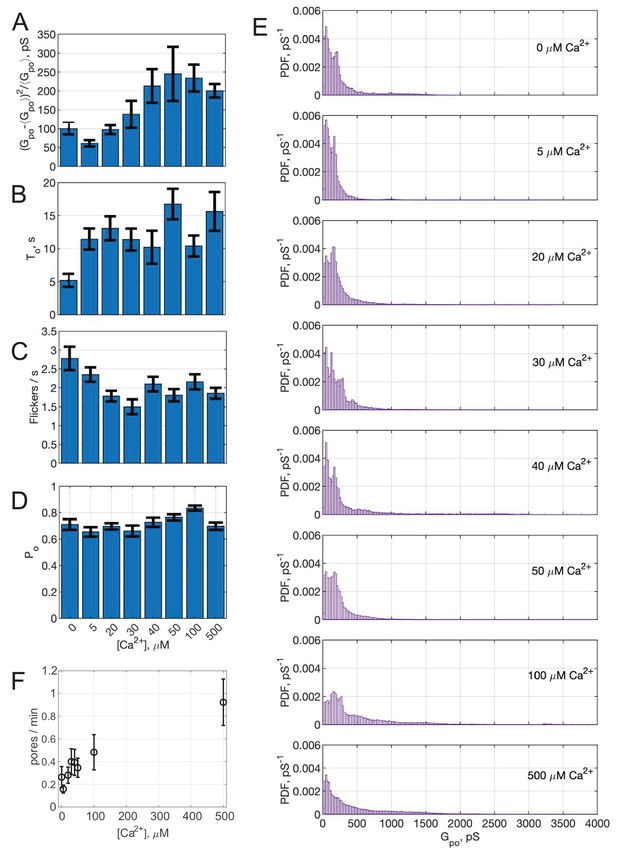
Additional fusion pore properties as a function of free calcium concentration, [Ca2+]free.
(A–D) Average single-pore conductance fluctuations relative to mean (A), average burst lifetime (B), average flicker rate (C), and the pore open probability (D) as a function of [Ca2+]free. Error bars are ± S.E.M. (E). Probability density functions (PDFs) for point-by-point open-pore conductance values at different [Ca2+]free. The probability density for pS increases as a function of calcium. (0 µM Ca2+: 44 pores/12 cells; 5 µM Ca2+: 54 pores/20 cells; 20 µM Ca2+: 114 pores/18 cells; 50 µM Ca2+: 88 pores/26 cells; 100 µM Ca2+: 98 pores/17 cells). (F) The fusion rate increases as a function of [Ca2+]free. For A-D, two-sample Kolmogorov-Smirnov test was used to assess significant differences between the "no C2AB" group and the rest. *, **, *** indicate p<0.05, 0.01, and 0.001, respectively.
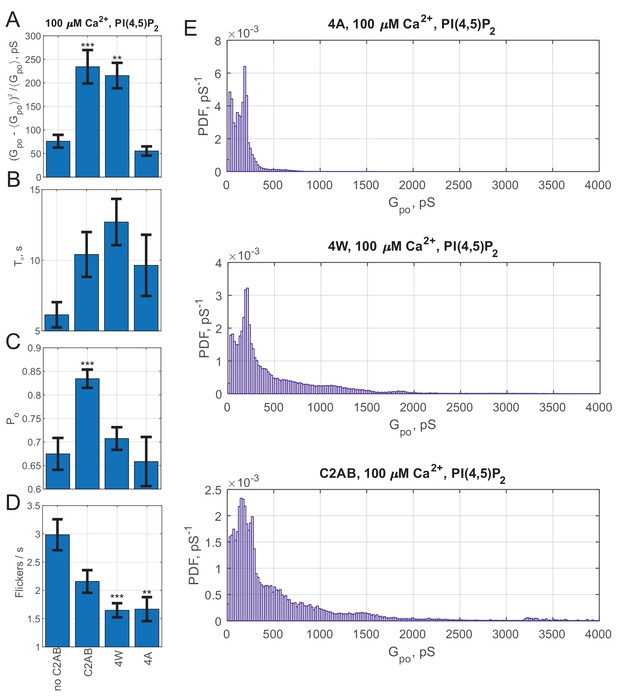
Additional fusion pore properties for Syt1 C2AB membrane penetration mutants.
(A–D) Average single-pore conductance fluctuations relative to mean (A), burst lifetime (B), pore open probability during a burst, (C), and flicker rate (D) for SNAREs alone (no C2AB), wild-type Syt1 C2AB (C2AB), the 4W mutant with enhanced membrane-penetration ability (M173W, F234W, V304W and I367W), and the 4A mutant which cannot penetrate membranes in response to calcium (M173A, F234A, V304A and I367A). Error bars are ± S.E.M. (E) Probability density functions (PDFs) for point-by-point open-pore conductance values for wild-type C2AB, and the membrane penetration mutants 4A and 4W. (4W: 115 pores/20 cells, 4A: 31 pores/9 cells). For A-D, two-sample Kolmogorov-Smirnov test was used to assess significant differences between the "no C2AB" group and the rest. *, **, *** indicate p<0.05, 0.01, and 0.001, respectively.
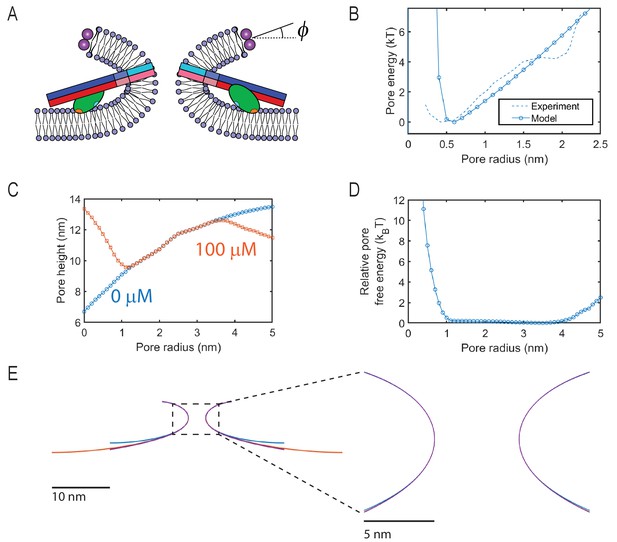
Results of the mathematical model of the fusion in the presence of SNAREs and Syt1 C2AB domains.
Data in (C) and (D) was smoothed using a moving average with width 0.5 nm. (A) Schematic illustrating a fusion pore with buried SNARE-Syt1 levers. represents the angle of twisting of the ApoE proteins. (B) Pore free energy as a function of radius predicted by the model and measured in a previous study (Wu et al., 2017b); the membrane tension was tuned to reproduce the experimental curve here. (C) Pore height, defined as the maximal separation between the NLP and the tCell membranes, as a function of pore radius with and without Ca2+. (D) Free energy difference between pores in the expanded state and those in the unexpanded state. Syt-SNARE complex-driven pore expansion costs a maximum of at low pore radii. (E) Fusion pores determined from the model using varying boundary conditions at the location where the fusion pore membrane joins the tCell (lower edge). The blue curve shows the boundary conditions used throughout the paper, while the orange curve uses (instead of 30 nm), and the purple curve imposes freely hinged boundary conditions at this location. These changes have very little effect, especially in the region close to the fusion pore (zoom in, right).
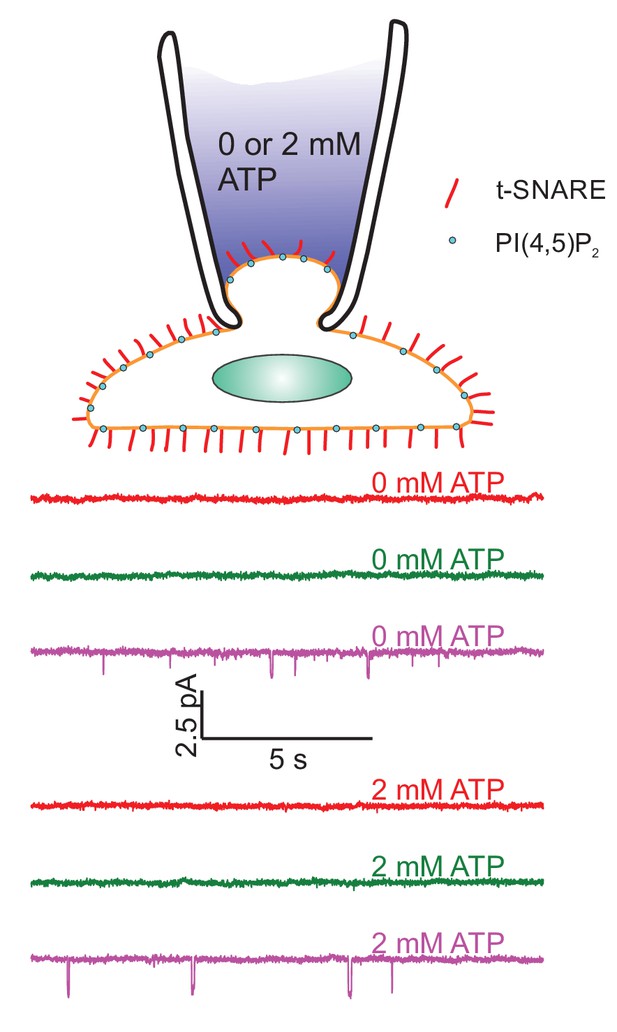
Lack of ATP-regulated channel activity in flipped t-SNARE cells.
Top: Schematic of the cell-attached recordings to test for ATP-regulated channel activity. Pipette solutions were the same as for single-pore measurements with 100 μM free calcium, but adjusted to contain either 0 or 2 mM ATP. Pipette potential was −40 mV. Middle: Current recordings under voltage clamp from three different patches (out of 21 total) in the absence of ATP. Bottom: Current recordings as in the middle panels, but in the presence of 2 mM freshly prepared ATP (26 cells were recorded). Occasionally, channel activity is recorded both with and without ATP.
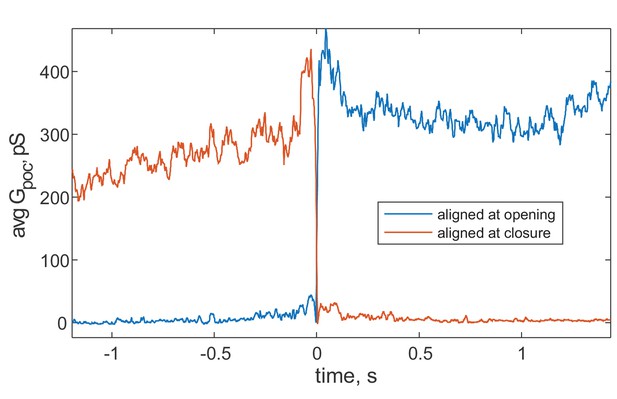
Average pore conductance as a function of time, after aligning pores to the moment of opening (blue) or closure (red).
Data for full-length Syt1, in the presence of 100 μM free Ca2+ and PI(4,5)P2. Other conditions also failed to yield large differences between pore opening or closure.
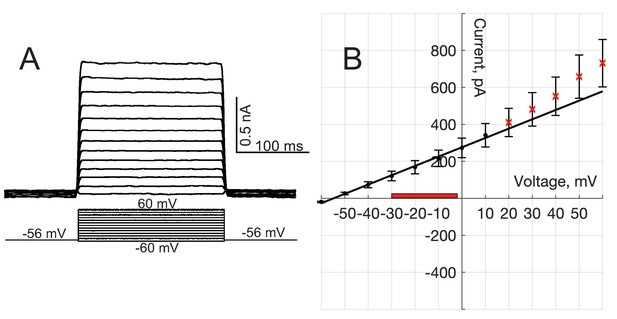
Whole-cell conductance of flipped t-SNARE HeLa cells.
(A) Whole-cell current responses to step changes in membrane potential under voltage-clamp, from a HeLa cell line expressing flipped t-SNAREs. (B) Current-voltage relationship. Currents were averaged for 27 cells. The average slope is nS (95% confidence interval), excluding the five highest voltages (red crosses). If all points are included, nS (95 % confidence interval). The range of mean open-pore currents and transmembrane voltages comprising 95% of the data values for C2AB in the presence of PI(4,5)P2 and 100 μM calcium are indicated as a red-colored box.
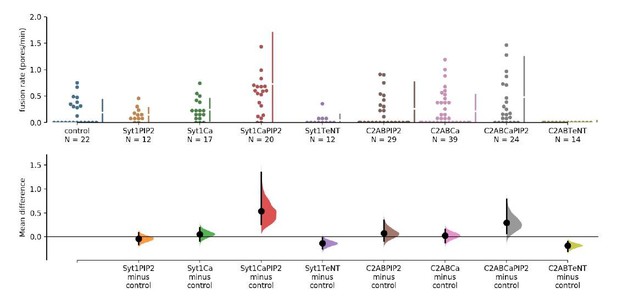
The mean difference for 8 comparisons against the shared control are shown in the above Cumming estimation plot.
The raw data is plotted on the upper axes. On the lower axes, mean differences are plotted as bootstrap sampling distributions. Each mean difference is depicted as a dot. Each 95% confidence interval is indicated by the ends of the vertical error bars. 5000 bootstrap samples were taken; the confidence interval is bias-corrected and accelerated. (see ref. 1).
Tables
Parameters used in the mathematical model with coarse-grained membranes, SNAREs and Synaptotagmin-1 C2B domains.
(A) Measured in Mitra et al., 2004. (B) Estimated previously as a best fit model parameter to experiments where NLP-tCell fusion pore was induced only by SNAREs (Wu et al., 2017b). (C) Consistent with NLP diameter measurements in this study. (D) Calculated as the weighted average of the hydration pressures of palmitoyl-2-oleoyl phosphatidylcholine (POPC) and 1,2-dioleoyl phosphatidylserine (DOPS) adopted from Rand and Parsegian, 1989, using a (85:15) molar ratio of POPC:DOPS as present in the NLP in the current study. (E) Values for the bending modulus range between (Brochard and Lennon, 1975; Khelashvili et al., 2013; Marsh, 2006; Cohen and Melikyan, 2004). We used a value of . (F) Obtained by fitting the membrane energy as a function of pore radius to measurements from a previous study using a similar method to measure the pore free energy in the absence of SNAREs, see Figure S8B (Wu et al., 2017b). (G) Calculated based on a 10° angle explored by t-SNARE TMDs around the equilibrium configuration, as measured in molecular dynamics simulations (Knecht and Grubmüller, 2003). (H) Estimated here from the persistence length of typical alpha helices and cross-sectional dimensions of the ApoE scaffold (see Appendix 1 subsection 'Mathematical Model of the ApoE scaffold'). (I) Measured in Syt1-liposome binding assays (Bai et al., 2004a; Bai et al., 2004b)
Symbol | Meaning | Value | Legend |
---|---|---|---|
Membrane thickness | (A) | ||
Zippering energy of SNARE’s linker and TMD domains | (B) | ||
NLP diameter | (C) | ||
Hydration interactions decay length | (B) | ||
Pressure pre-factor for steric hydration interaction | (D) | ||
Membrane bending modulus | (E) | ||
Membrane tension | (F) | ||
Solid angle explored by fully zippered SNAREs | (G) | ||
Twisting rigidity of ApoE scaffold | (H) | ||
Apparent affinity of Syt1 to calcium in the presence of PIP2 containing membranes | (I) | ||
Hill coefficient | 2.3 | (I) |