Acquisition of cellular properties during alveolar formation requires differential activity and distribution of mitochondria
Figures
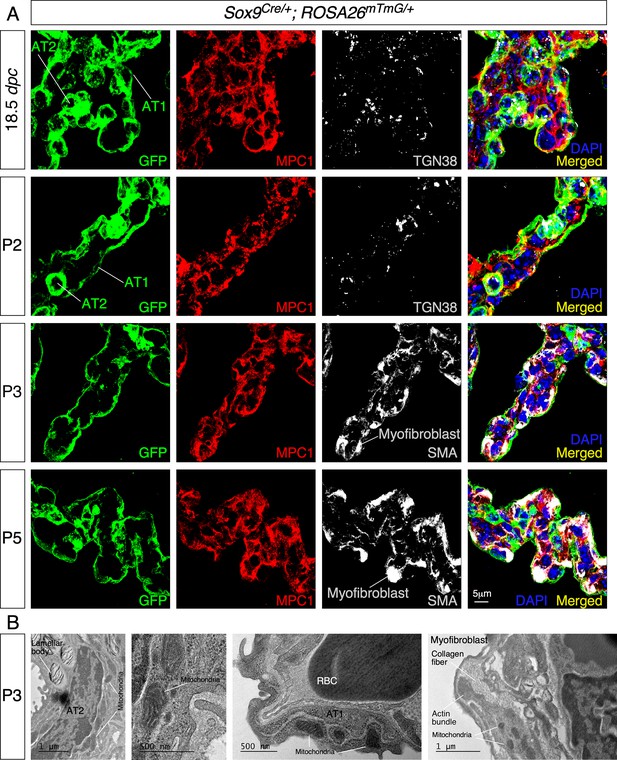
Mitochondria display subcellular concentration in alveolar epithelial cells and mesenchymal myofibroblasts of mouse lungs.
(A) Immunostaining of lung sections collected from Sox9Cre/+; ROSA26mTmG/+ mice at 18.5 days post coitus (dpc) and different postnatal (P) stages as indicated. The GFP signal identified alveolar epithelial cells (alveolar type I [AT1] and alveolar type II [AT2] cells) while myofibroblasts were characterized by smooth muscle actin (SMA) expression. Moreover, mitochondria were labeled by MPC1; the trans-Golgi network was visualized by TGN38. Enhanced MPC1 signal was distributed nonuniformly in both alveolar epithelial cells and myofibroblasts. (B) Transmission electron micrographs of lungs collected from wild-type mice at P3. Prominent features in a given lung cell type include lamellar bodies in AT2 cells, elongated cell membrane in AT1 cells, and actin bundles and collagen fibers in myofibroblasts. RBC, red blood cell.
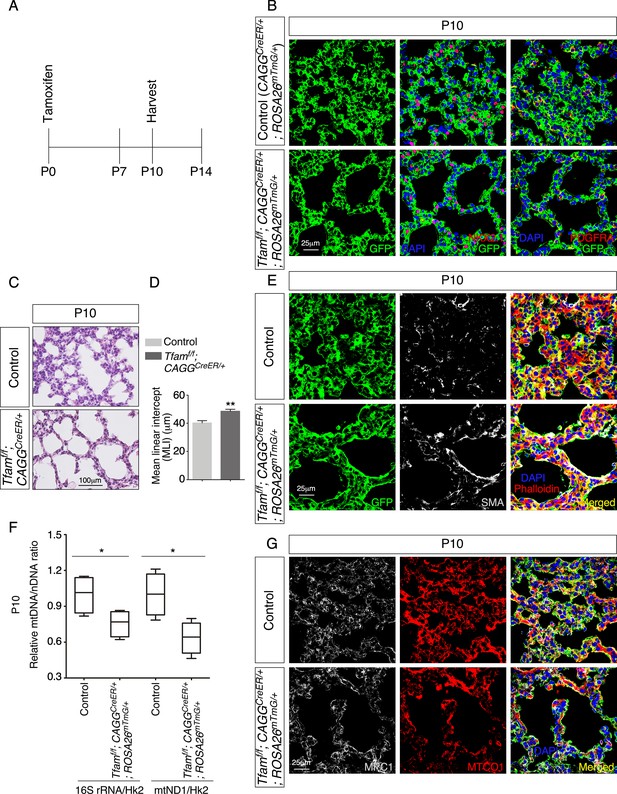
Global inactivation of Tfam in postnatal mice results in alveolar defects.
(A) Schematic diagram of the time course of postnatal (P) administration of tamoxifen and harvest of mouse lungs. (B) Immunostaining of lungs collected from CAGGCreER/+; ROSA26mTmG/+ (control) and Tfamf/f; CAGGCreER/+; ROSA26mTmG/+ mice at P10 that had received tamoxifen at P0. The GFP signal represents sites of induced CreER activity. Nuclear NKX2.1 staining marked all lung epithelial cells while PDGFRA immunoreactivity labeled mesenchymal fibroblasts/myofibroblasts. (C) Hematoxylin and eosin-stained lung sections of control and Tfamf/f; CAGGCreER/+; ROSA26mTmG/+ mice at P10. Histological analysis revealed the presence of enlarged saccules and retarded development of secondary septa in the mutant lungs. (D) Measurement of the mean linear intercept (MLI) in control and Tfamf/f; CAGGCreER/+; ROSA26mTmG/+ lungs at P10 (n = 4 for each group). The MLI was increased in Tfam-deficient lungs. (E) Immunostaining of lung sections collected from control and Tfamf/f; CAGGCreER/+; ROSA26mTmG/+ mice at P10. Smooth muscle actin (SMA) expression was characteristic of myofibroblasts and phalloidin stained the actin filaments. (F) Quantification of the relative ratio of mitochondrial DNA (mtDNA), 16S rRNA, and mitochondrially encoded NADH dehydrogenase 1 (mtND1), to nDNA (nuclear DNA), hexokinase (Hk2), in lysates derived from control and Tfamf/f; CAGGCreER/+; ROSA26mTmG/+ lungs (n = 4 for each group). (G) Immunostaining of lung sections collected from control and Tfamf/f; CAGGCreER/+; ROSA26mTmG/+ mice at P10. MPC1 antibodies marked mitochondria; MTCO1 antibodies detected cytochrome c oxidase, the expression of which was controlled by Tfam. All values are mean ± SEM. *p<0.05; **p<0.01 (unpaired Student’s t-test).
-
Figure 2—source data 1
Mean linear intercept and relative mitochondrial DNA (mtDNA)/nuclear DNA (nDNA) ratio.
- https://cdn.elifesciences.org/articles/68598/elife-68598-fig2-data1-v2.xlsx
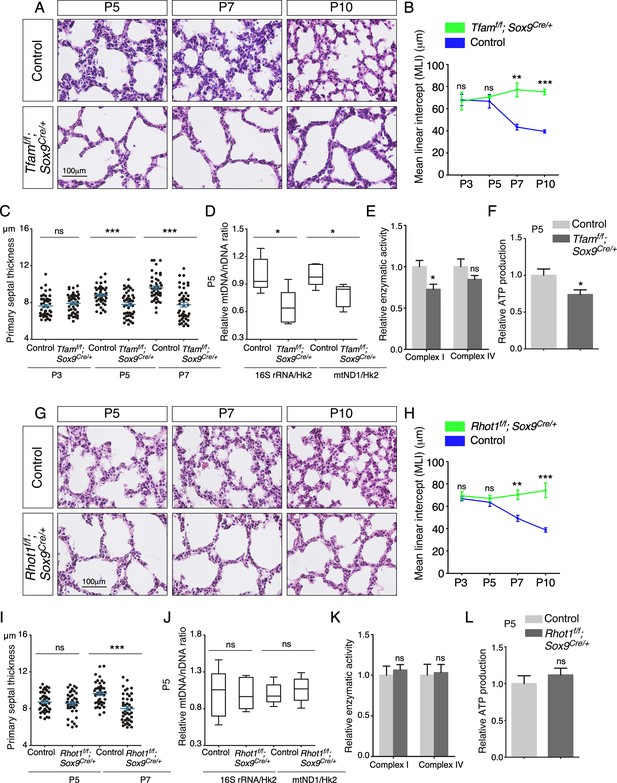
Elimination of Tfam or Rhot1 in the epithelium of mouse lungs disrupts alveolar formation.
(A) Hematoxylin and eosin-stained lung sections of control and Tfamf/f; Sox9Cre/+ mice at different postnatal (P) stages as indicated. Histological analysis revealed the presence of enlarged saccules and failure in secondary septation in the mutant lungs. (B) Measurement of the mean linear intercept (MLI) in control and Tfamf/f; Sox9Cre/+ lungs at P3–P10 (n = 3 for each group). The MLI was increased in Tfam-deficient lungs. (C) Measurement of the primary septal thickness in control and Tfamf/f; Sox9Cre/+ lungs at P3–P7 (n = 3 for each group). (D) Quantification of the relative ratio of mitochondrial DNA (mtDNA), 16S rRNA, and mitochondrially encoded NADH dehydrogenase 1 (mtND1), to nuclear DNA (nDNA), hexokinase 2 (Hk2), in lysates derived from control and Tfamf/f; Sox9Cre/+ lungs at P5 (n = 5 for each group). (E) Quantification of the relative enzymatic activity of mitochondrial complex I and complex IV in control and Tfamf/f; Sox9Cre/+ lungs at P5 (n = 5 for each group). (F) Measurement of relative ATP production in control and Tfamf/f; Sox9Cre/+ lungs at P5 (n = 5 for each group). (G) Hematoxylin and eosin-stained lung sections of control and Rhot1f/f; Sox9Cre/+ mice at different postnatal stages as indicated. Histological analysis detected enlarged saccules and lack of secondary septa in the mutant lungs. (H) Measurement of the MLI in control and Rhot1f/f; Sox9Cre/+ lungs at P3–P10 (n = 3 for each group). The MLI was increased in Rhot1-deficient lungs. (I) Measurement of the primary septal thickness in control and Rhot1f/f; Sox9Cre/+ lungs at P5–P7 (n = 3 for each group). (J) Quantification of the relative ratio of mtDNA, 16S rRNA, and mtND1, to nDNA, Hk2, in lysates derived from control and Rhot1f/f; Sox9Cre/+ lungs at P5 (n = 5 for each group). (K) Quantification of the relative enzymatic activity of mitochondrial complex I and complex IV in control and Rhot1f/f; Sox9Cre/+ lungs at P5 (n = 5 for each group). (L) Measurement of relative ATP production in control and Rhot1f/f; Sox9Cre/+ lungs at P5 (n = 5 for each group). All values are mean ± SEM. **p<0.01; ***p<0.001; ns, not significant (unpaired Student’s t-test).
-
Figure 3—source data 1
Mean linear intercept, primary septal thickness, relative mitochondrial DNA (mtDNA)/nuclear DNA (nDNA) ratio, relative enzymatic activity, and relative ATP production.
- https://cdn.elifesciences.org/articles/68598/elife-68598-fig3-data1-v2.xlsx
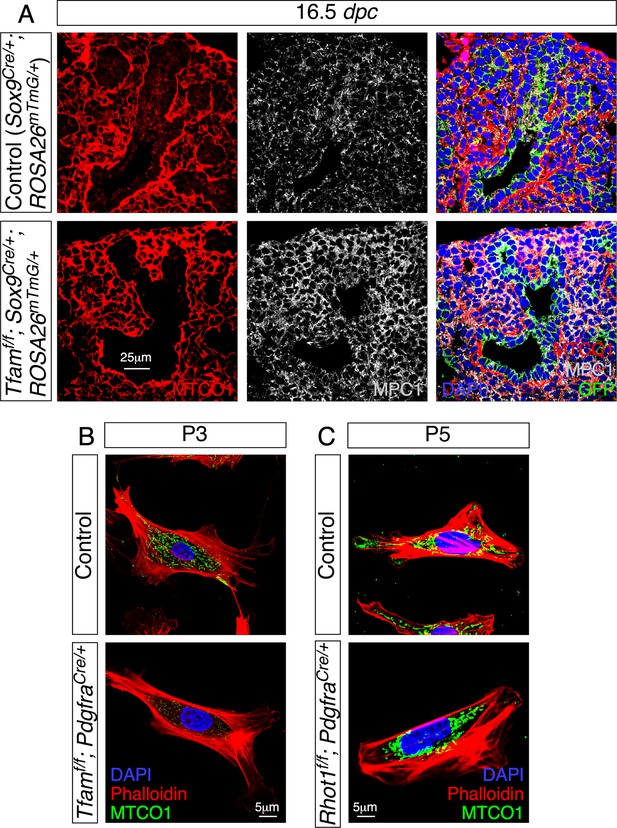
Loss of Tfam or Rhot1 disrupts mitochondrial activity and distribution, respectively.
(A) Immunostaining of lung sections collected from Sox9Cre/+; ROSA26mTmG/+ (control) and Tfamf/f; Sox9Cre/+; ROSA26mTmG/+ mice at 16.5 days post coitus (dpc). MPC1 antibodies marked mitochondria while MTCO1 antibodies detected cytochrome c oxidase, the expression of which was controlled by Tfam. (B) Immunostaining of fibroblasts derived from control or Tfamf/f; PdgfraCre/+ lungs at postnatal (P) day 3. (C) Immunostaining of fibroblasts derived from control or Rhot1f/f; PdgfraCre/+ lungs at P5.
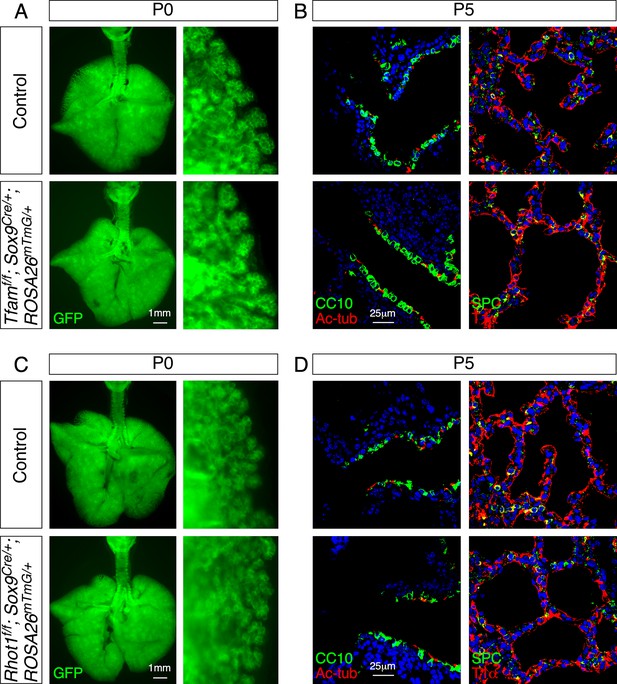
Elimination of Tfam or Rhot1 in the epithelium of mouse lungs does not perturb saccule formation or cell-type specification.
(A) Surface view of dissected lungs from Sox9Cre/+; ROSA26mTmG/+ (control) and Tfamf/f; Sox9Cre/+; ROSA26mTmG/+ mice at postnatal (P) day 0. No difference in saccule formation was noted between control and mutant lungs. (B) Immunostaining of lung sections from control and Tfamf/f; Sox9Cre/+; ROSA26mTmG/+ mice at P5. Specification of lung cell types (e.g., club cell [CC10+], ciliated cell [Ac-tub+], AT1 cell [T1α+], AT2 cell [SPC+]) was unaffected. (C) Surface view of dissected lungs from Sox9Cre/+; ROSA26mTmG/+ (control) and Rhot1f/f; Sox9Cre/+; ROSA26mTmG/+ mice at P0. No difference in saccule formation was noted between control and mutant lungs. (D) Immunostaining of lung sections from control and Rhot1f/f; Sox9Cre/+; ROSA26mTmG/+ mice at P5. Specification of lung cell types was unaffected.
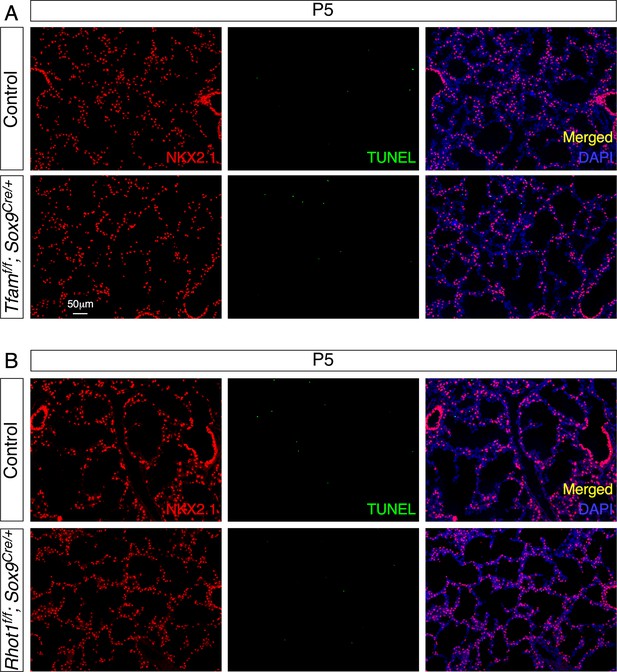
Loss of epithelial Tfam or Rhot1 does not lead to cell death in the lungs.
(A) Immunostaining of lung sections collected from control and Tfamf/f; Sox9Cre/+ mice at postnatal (P) day 5. NKX2.1 antibodies marked lung epithelial cells. The rate of cell death by TUNEL assay was unaltered in the absence of epithelial Tfam. (B) Immunostaining of lung sections collected from control and Rhot1f/f; Sox9Cre/+ mice at P5. The rate of cell death by TUNEL assay was similar between control and Rhot1-deficient lungs.
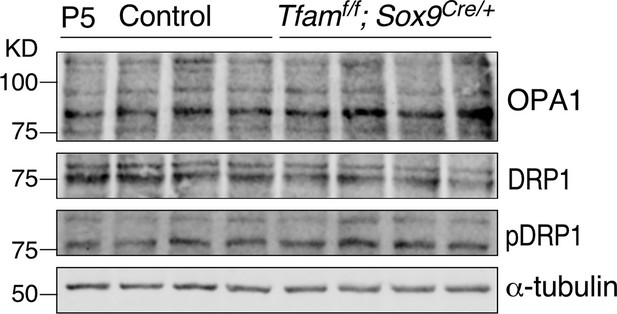
Loss of epithelial Tfam does not affect the regulators of mitochondrial fusion and fission.
Western blotting of lysates derived from control and Tfamf/f; Sox9Cre/+ mouse lungs at postnatal (P) day 5. The expression levels of OPA1 (dynamin-like 120 kDa protein), DRP1 (dynamin-related protein 1), and pDRP1 (phosphorylated DRP1) showed no apparent difference between control and Tfam-deficient lungs. OPA1 controls mitochondrial fusion while DRP1 regulates mitochondrial fission. Note that multiple isoforms of OPA1 were present. The numbers indicate the positions of protein size standards.
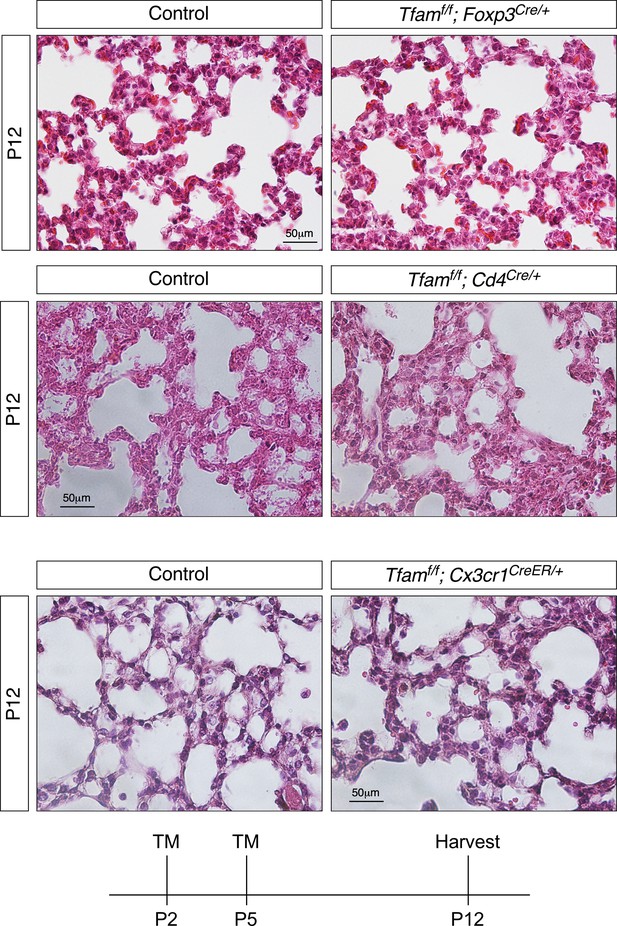
Loss of Tfam in T cells by Foxp3–Cre or Cd4-Cre and in macrophages by activated Cx3cr1-CreER does not perturb alveolar formation.
Hematoxylin and eosin-stained lung sections of control, Tfamf/f; Foxp3Cre/+ and Tfamf/f; Cd4Cre/ mice and of control and Tfamf/f; Cx3cr1CreER/+ mice at postnatal (P) day 12. Control and Tfamf/f; Cx3cr1CreER/+ mice were injected with tamoxifen (TM) at P2 and P5 and lungs were harvested at P12. Histological analysis revealed no difference between control, Tfamf/f; Foxp3Cre/+ and Tfamf/f; Cd4Cre/+ lungs and between control and Tfamf/f; Cx3cr1CreER/+ lungs.
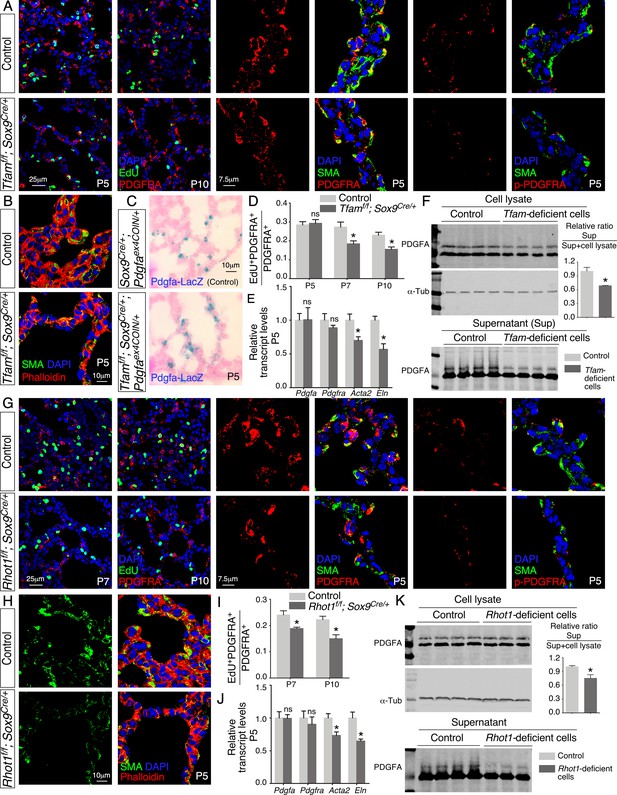
Loss of epithelial Tfam or Rhot1 compromises PDGF release.
(A) Immunostaining of lungs collected from control and Tfamf/f; Sox9Cre/+ mice at postnatal (P) day 5 or 10, some of which were injected with EdU as indicated. (B) Immunostaining of lungs collected from control and Tfamf/f; Sox9Cre/+ mice at P5. (C) LacZ staining (blue) of lung sections collected from Sox9Cre/+; Pdgfaex4COIN/+ (control) and Tfamf/f; Sox9Cre/+; Pdgfaex4COIN/+ mice. The slides were counterstained with eosin (red). No difference in the intensity of LacZ staining in the lung was noted between these two mouse lines. (D) Quantification of fibroblast/myofibroblast proliferation in control and Tfamf/f; Sox9Cre/+ lungs at P5, P7, and P10 (n = 3 for each group). The rate of fibroblast/myofibroblast proliferation was calculated as the ratio of the number of EdU+ fibroblasts/myofibroblasts (EdU+ PDGFRA+) to the number of fibroblasts/myofibroblasts (PDGFRA+). The percentage of proliferating fibroblasts/myofibroblasts was reduced in Tfamf/f; Sox9Cre/+ compared to controls at P7 and P10. (E) qPCR analysis of gene expression in control and Tfamf/f; Sox9Cre/+ lungs at P5 (n = 3 for each group). While no difference in expression levels was noted for Pdgfa and Pdgfra between control and Tfamf/f; Sox9Cre/+ lungs, the expression levels of Acta2 (smooth muscle actin [SMA]) and Eln (elastin) were significantly reduced in the absence of Tfam. (F) Western blot analysis of cell lysates and supernatants from control and Tfam-deficient cells (n = 4 for each group) lentivirally transduced with PDGFA-expressing constructs. The amount of PDGFA released into the media was reduced in Tfam-deficient cells compared to controls. α-Tubulin served as a loading control. (G) Immunostaining of lungs collected from control and Rhot1f/f; Sox9Cre/+ mice at P5, P7, or P10, some of which were injected with EdU as indicated. (H) Immunostaining of lungs collected from control and Rhot1f/f; Sox9Cre/+ mice at P5. (I) Quantification of fibroblast/myofibroblast proliferation in control and Rhot1f/f; Sox9Cre/+ lungs at P7 and P10 (n = 3 for each group). The percentage of proliferating fibroblasts/myofibroblasts was reduced in Rhot1f/f; Sox9Cre/+ compared to controls at P7 and P10. (J) qPCR analysis of gene expression in control and Rhot1f/f; Sox9Cre/+ lungs at P5 (n = 3 for each group). The expression levels of Pdgfa and Pdgfra were unaltered between control and Rhot1f/f; Sox9Cre/+ lungs; the expression levels of Acta2 and Eln were significantly reduced in the absence of Rhot1. (K) Western blot analysis of cell lysates and supernatants from control and Rhot1-deficient cells (n = 4 for each group) lentivirally transduced with PDGFA-expressing constructs. The amount of PDGFA released into the media was reduced in Rhot1-deficient cells compared to controls. α-Tubulin served as a loading control. All values are mean ± SEM. *p<0.05; ns, not significant (unpaired Student’s t-test).
-
Figure 4—source data 1
EdU quantification, relative transcript levels, and quantification of PDGFA secretion.
- https://cdn.elifesciences.org/articles/68598/elife-68598-fig4-data1-v2.xlsx
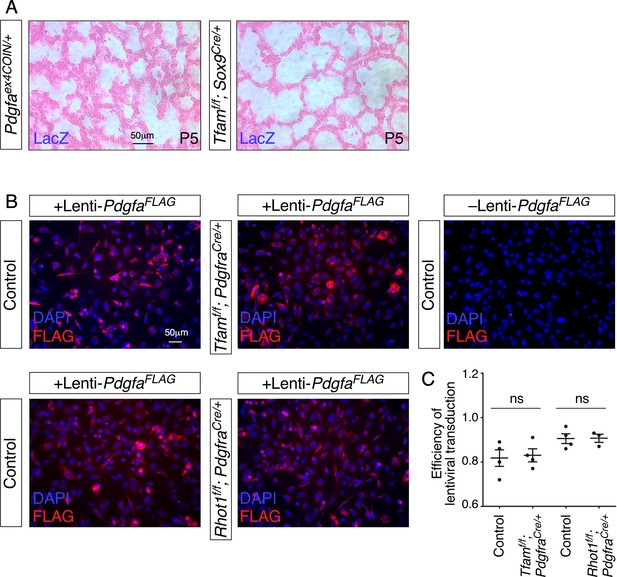
Controls for LacZ staining and lentiviral transduction.
(A) LacZ staining (blue) of lung sections collected from Pdgfaex4COIN/+ and Tfamf/f; Sox9Cre/+ mice at postnatal (P) day 5. The slides were counterstained with eosin (red). No LacZ staining in the lung was detected in these two mouse lines. (B) Immunostaining of control, Tfam-deficient (n = 4 for each group) and Rhot1-deficient cells (n = 3 for each group) lentivirally transduced with PDGFAFLAG-expressing constructs. (C) Quantification of PDGFAFLAG-expressing cells revealed no difference in transduction efficiency among these three cell lines.
-
Figure 4—figure supplement 1—source data 1
Efficiency of lentiviral transduction.
- https://cdn.elifesciences.org/articles/68598/elife-68598-fig4-figsupp1-data1-v2.xlsx
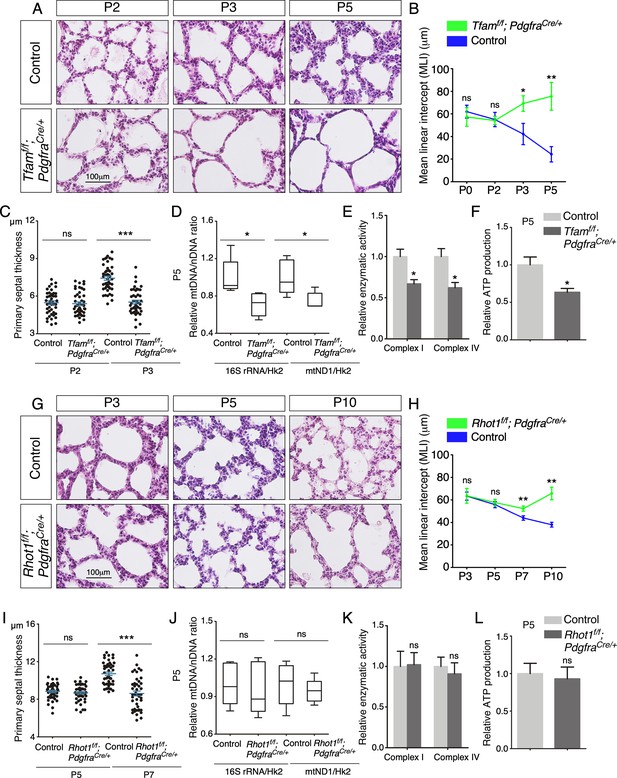
Elimination of Tfam or Rhot1 in mouse lung fibroblasts/myofibroblasts impairs alveolar formation.
(A) Hematoxylin and eosin-stained lung sections of control and Tfamf/f; PdgfraCre/+ mice at different postnatal (P) stages as indicated. Histological analysis revealed the presence of enlarged saccules and defective development of secondary septa in the mutant lungs. (B) Measurement of the mean linear intercept (MLI) in control and Tfamf/f; PdgfraCre/+ lungs at P0–P5 (n = 3 for each group). The MLI was increased in Tfam-deficient lungs. (C) Measurement of the primary septal thickness in control and Tfamf/f; PdgfraCre/+ lungs at P2–P3 (n = 3 for each group). (D) Quantification of the relative ratio of mitochondrial DNA (mtDNA), 16S rRNA, and mitochondrially encoded NADH dehydrogenase 1 (mtND1), to nuclear DNA (nDNA), hexokinase 2 (Hk2), in lysates derived from control and Tfamf/f; PdgfraCre/+ lungs at P5 (n = 5 for each group). (E) Quantification of the relative enzymatic activity of mitochondrial complex I and complex IV in control and Tfamf/f; PdgfraCre/+ lungs at P5 (n = 5 for each group). (F) Measurement of relative ATP production in control and Tfamf/f; PdgfraCre/+ lungs at P5 (n = 5 for each group). (G) Hematoxylin and eosin-stained lung sections of control and Rhot1f/f; PdgfraCre/+ mice at different postnatal stages as indicated. Histological analysis detected enlarged saccules and lack of secondary septa in the mutant lungs. (H) Measurement of the MLI in control and Rhot1f/f; PdgfraCre/+ lungs at P3–P10 (n = 3 for each group). The MLI was increased in Rhot1-deficient lungs. (I) Measurement of the primary septal thickness in control and Rhot1f/f; PdgfraCre/+ lungs at P5–P7 (n = 3 for each group). (J) Quantification of the relative ratio of mtDNA, 16S rRNA, and mtND1, to nDNA, Hk2, in lysates derived from control and Rhot1f/f; PdgfraCre/+ lungs at P5 (n = 5 for each group). (K) Quantification of the relative enzymatic activity of mitochondrial complex I and complex IV in control and Rhot1f/f; PdgfraCre/+ lungs at P5 (n = 5 for each group). (L) Measurement of relative ATP production in control and Rhot1f/f; PdgfraCre/+ lungs at P5 (n = 5 for each group). All values are mean ± SEM. *p<0.05; **p<0.01; ns, not significant (unpaired Student’s t-test).
-
Figure 5—source data 1
Mean linear intercept, primary septal thickness, relative mitochondrial DNA (mtDNA)/nuclear DNA (nDNA) ratio, relative enzymatic activity, and relative ATP production.
- https://cdn.elifesciences.org/articles/68598/elife-68598-fig5-data1-v2.xlsx
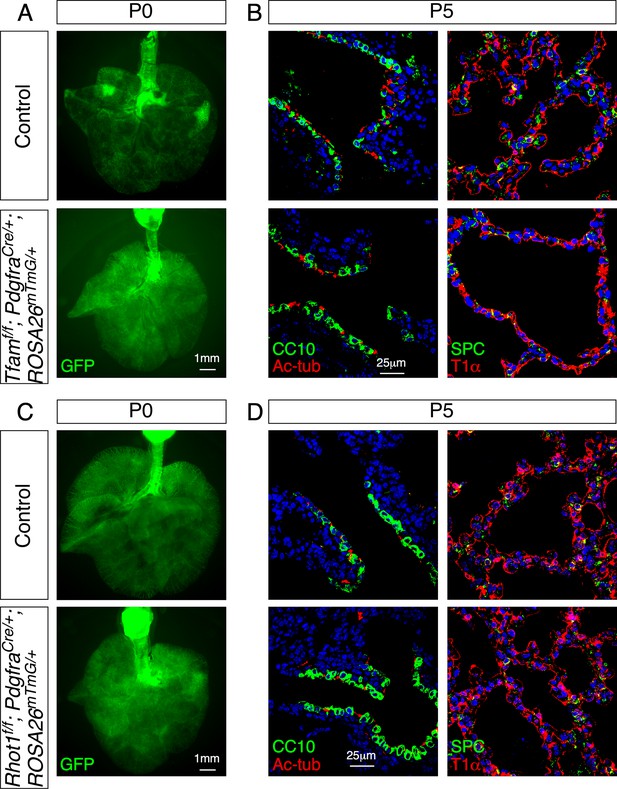
Removal of Tfam or Rhot1 in mouse lung fibroblasts/myofibroblasts does not perturb saccule formation or cell-type specification.
(A) Surface view of dissected lungs from PdgfraCre/+; ROSA26mTmG/+ (control) and Tfamf/f; PdgfraCre/+; ROSA26mTmG/+ mice at postnatal (P) day 0. No difference in saccule formation was noted between control and mutant lungs. (B) Immunostaining of lung sections from control and Tfamf/f; PdgfraCre/+; ROSA26mTmG/+ mice at P5. Specification of lung cell types was unaffected. (C) Surface view of dissected lungs from control and Rhot1f/f; PdgfraCre/+; ROSA26mTmG/+ mice at P0. No difference in saccule formation was noted between control and mutant lungs. (D) Immunostaining of lung sections from control and Rhot1f/f; PdgfraCre/+; ROSA26mTmG/+ mice at P5. Specification of lung cell types was unaffected.
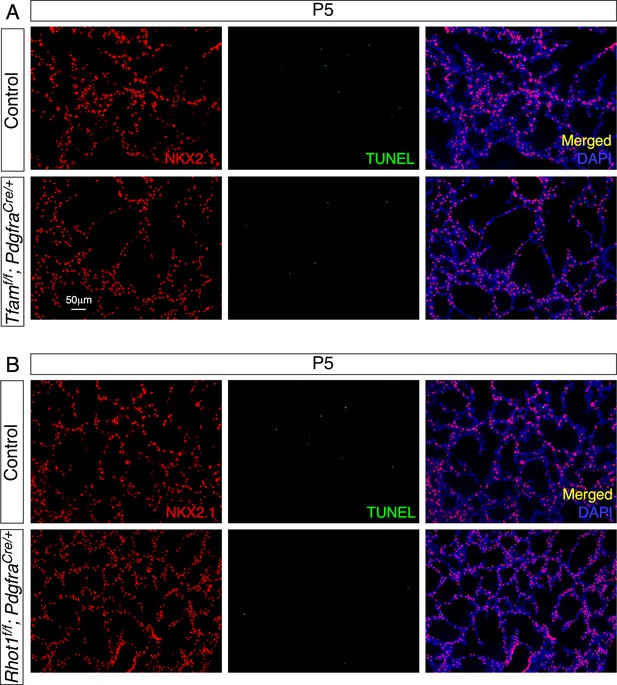
Loss of mesenchymal Tfam or Rhot1 does not lead to cell death in the lungs.
(A) Immunostaining of lung sections collected from control and Tfamf/f; PdgfraCre/+ mice at postnatal (P) day 5. NKX2.1 antibodies marked lung epithelial cells. The rate of cell death by TUNEL assay was unaltered in the absence of mesenchymal Tfam. (B) Immunostaining of lung sections collected from control and Rhot1f/f; PdgfraCre/+ mice at P5. The rate of cell death by TUNEL assay was similar between control and Rhot1-deficient lungs.
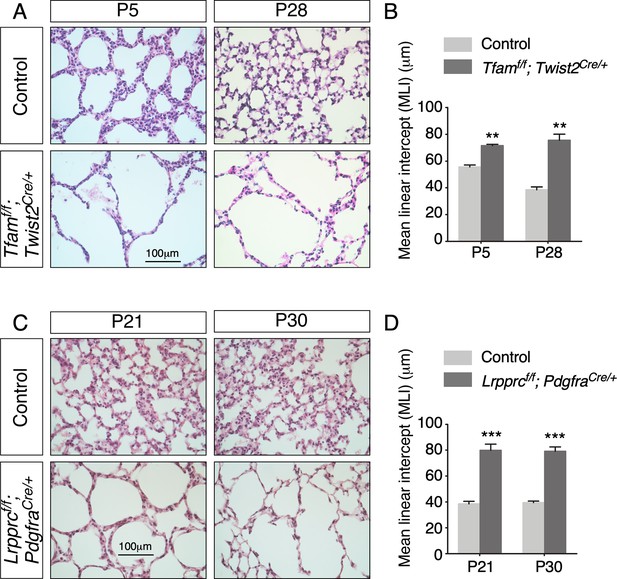
Inactivation of Tfam or Lrpprc in mouse lung fibroblasts/myofibroblasts leads to alveolar defects.
(A) Hematoxylin and eosin-stained lung sections of control and Tfamf/f; Twist2Cre/+ mice at different postnatal (P) stages as indicated. Histological analysis revealed the presence of enlarged saccules and defective development of secondary septa in the mutant lungs. (B) Measurement of the mean linear intercept (MLI) in control and Tfamf/f; Twist2Cre/+ lungs at P5 and P28 (n = 3 for each group). The MLI was increased in Tfam-deficient lungs. (C) Hematoxylin and eosin-stained lung sections of control and Lrpprcf/f; PdgfraCre/+ mice at P21 and P30. Saccules were enlarged with loss of secondary septation in the mutant lungs. (D) The MLI was increased in Lrpprc-deficient lungs at P21 and P30 (n = 3 for each group). All values are mean ± SEM. *p<0.05; **p<0.01; ***p<0.001; ns, not significant (unpaired Student’s t-test).
-
Figure 5—figure supplement 3—source data 1
Mean linear intercept.
- https://cdn.elifesciences.org/articles/68598/elife-68598-fig5-figsupp3-data1-v2.xlsx
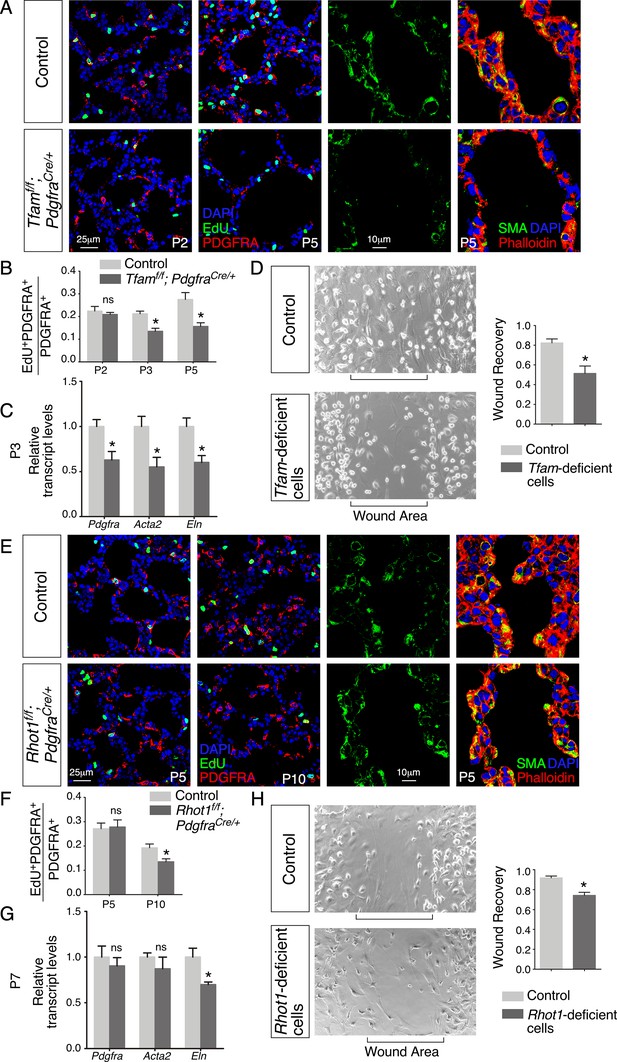
Loss of mesenchymal Tfam or Rhot1 compromises fibroblast/myofibroblast migration.
(A) Immunostaining of lungs collected from control and Tfamf/f; PdgfraCre/+ mice at postnatal (P) day 2 and 5, some of which were injected with EdU as indicated. (B) Quantification of fibroblast/myofibroblast proliferation in control and Tfamf/f; PdgfraCre/+ lungs at P2, P3, and P5 (n = 3 for each group). The rate of fibroblast/myofibroblast proliferation was calculated as the ratio of the number of EdU+ fibroblasts/myofibroblasts (EdU+ PDGFRA+) to the number of fibroblast/myofibroblasts (PDGFRA+). The percentage of proliferating fibroblasts/myofibroblasts was reduced in Tfamf/f; PdgfraCre/+ compared to controls at P3 and P5. (C) qPCR analysis of gene expression in control and Tfamf/f; PdgfraCre/+ lungs at P3 (n = 3 for each group). The expression levels of Pdgfra, Acta2, and Eln were significantly reduced in Tfamf/f; PdgfraCre/+ lungs compared to controls. (D) Wound recovery assays to assess the migratory ability of fibroblasts/myofibroblasts derived from control and Tfamf/f; PdgfraCre/+ lungs (n = 3 for each group). Within 36–48 hr, the wound area has been populated by migrating fibroblasts/myofibroblasts derived from control lungs. By contrast, fewer fibroblasts/myofibroblasts from Tfamf/f; PdgfraCre/+ lungs reached the wound area within the same time frame. Wound recovery by fibroblasts/myofibroblasts from control and Tfamf/f; PdgfraCre/+ lungs was quantified. (E) Immunostaining of lungs collected from control and Rhot1f/f; PdgfraCre/+ mice at P5 and P10, some of which were injected with EdU as indicated. (F) Quantification of fibroblasts/myofibroblasts proliferation in control and Rhot1f/f; PdgfraCre/+ lungs at P5 and P10 (n = 3 for each group). The percentage of proliferating fibroblasts/myofibroblasts was decreased in Rhot1f/f; PdgfraCre/+ compared to controls at P10. (G) qPCR analysis of gene expression in control and Rhot1f/f; PdgfraCre/+ lungs at P7 (n = 3 for each group). The expression levels of Eln were significantly reduced in Rhot1f/f; PdgfraCre/+ lungs in comparison with controls. (H) Wound recovery assays to assess the migratory ability of fibroblasts/myofibroblasts derived from control and Rhot1f/f; PdgfraCre/+ lungs (n = 3 for each group). Fewer fibroblasts/myofibroblasts from Rhot1f/f; PdgfraCre/+ lungs reached the wound area within the same time frame compared to controls. Wound recovery by fibroblasts/myofibroblasts from control and Rhot1f/f; PdgfraCre/+ lungs was quantified. All values are mean ± SEM. *p<0.05; ns, not significant (unpaired Student’s t-test).
-
Figure 6—source data 1
EdU quantification, relative transcript levels, and quantification of wound recovery.
- https://cdn.elifesciences.org/articles/68598/elife-68598-fig6-data1-v2.xlsx
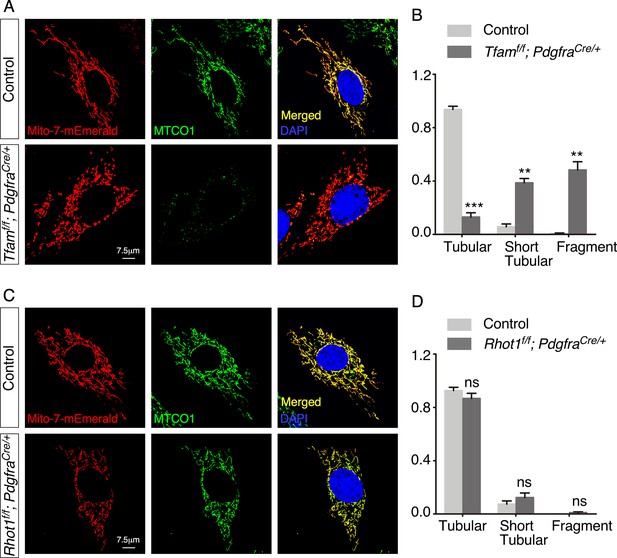
Tfam- but not Rhot1-deficient fibroblasts display short tubular and fragmented mitochondria.
(A) Immunostaining of fibroblasts derived from control and Tfamf/f; PdgfraCre/+ lungs at postnatal (P) day 5. Cells were lentivirally transduced with Mito-7-mEmerald-expressing constructs to visualize mitochondria and stained with MTCO1 antibodies to detect cytochrome c oxidase. (B) Quantification of mitochondrial network and morphology. Tubular mitochondria were the dominant form in control fibroblasts, while short tubular and fragmented mitochondria were found in Tfam-deficient fibroblasts. (C) Immunostaining of fibroblasts derived from control and Rhot1f/f; PdgfraCre/+ lungs at P5. (D) Quantification of mitochondrial network and morphology. No apparent difference in mitochondrial morphology was found between control and Rhot1-deficient fibroblasts.
-
Figure 6—figure supplement 1—source data 1
Quantification of mitochondrial morphology.
- https://cdn.elifesciences.org/articles/68598/elife-68598-fig6-figsupp1-data1-v2.xlsx
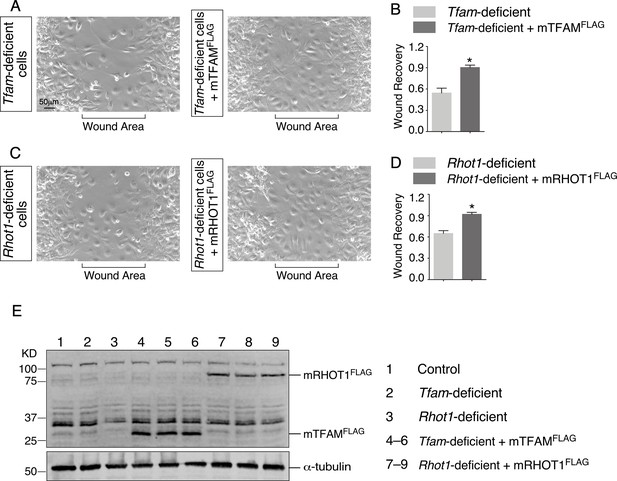
The migratory defect in Tfam- and Rhot1-deficient fibroblasts/myofibroblasts is rescued by expression of TFAM and RHOT1, respectively.
(A) Wound recovery assays to assess the migratory ability of fibroblasts/myofibroblasts derived from Tfamf/f; PdgfraCre/+ mouse lungs (n = 3 for each group). Cells were transduced with control or mouse TFAMFLAG (mTFAMFLAG)-expressing constructs. Within 36–48 hr, the wound area has been populated by migrating Tfam-deficient fibroblasts/myofibroblasts expressing mTFAMFLAG. By contrast, fewer Tfam-deficient fibroblasts/myofibroblasts reached the wound area within the same time frame. (B) Wound recovery by Tfam-deficient fibroblasts/myofibroblasts and Tfam-deficient fibroblasts/myofibroblasts expressing mTFAMFLAG was quantified. (C) Wound recovery assays to assess the migratory ability of fibroblasts/myofibroblasts derived from Rhot1f/f; PdgfraCre/+ mouse lungs (n = 3 for each group). Cells were transduced with control or mouse RHOT1FLAG (mRHOT1FLAG)-expressing constructs. Within 36–48 hr, the wound area has been populated by migrating Rhot1-deficient fibroblasts/myofibroblasts expressing mRHOT1FLAG. By contrast, fewer Rhot1-deficient fibroblasts/myofibroblasts reached the wound area within the same time frame. (D) Wound recovery by Rhot1-deficient fibroblasts and Rhot1-deficient fibroblasts/myofibroblasts expressing mRHOT1FLAG was quantified. (E) Western blotting of cell lysates from control fibroblasts/myofibroblasts, Tfam-deficient and Rhot1-deficient fibroblasts/myofibroblasts expressing mTFAMFLAG or mRHOT1FLAG, respectively, as indicated. The numbers indicate the positions of protein size standards.
-
Figure 6—figure supplement 2—source data 1
Quantification of wound recovery.
- https://cdn.elifesciences.org/articles/68598/elife-68598-fig6-figsupp2-data1-v2.xlsx
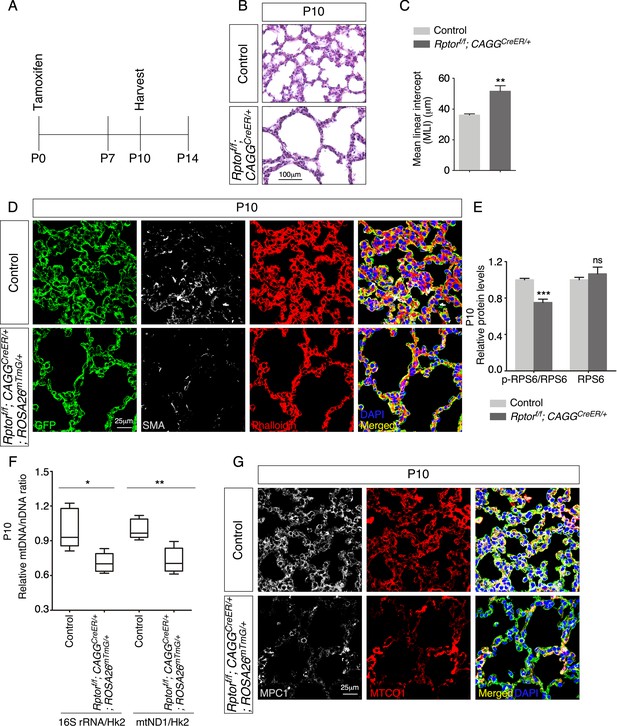
Postnatal inactivation of Rptor in mice results in alveolar defects.
(A) Schematic diagram of the time course of postnatal (P) administration of tamoxifen and harvest of mouse lungs. (B) Hematoxylin and eosin-stained lung sections of control and Rptorf/f; CAGGCreER/+ mice at P10. Histological analysis revealed the presence of enlarged saccules and retarded development of secondary septa in the mutant lungs. (C) Measurement of the mean linear intercept (MLI) in control and Rptorf/f; CAGGCreER/+ lungs at P10 (n = 5 for each group). The MLI was increased in Rptor-deficient lungs. (D) Immunostaining of lung sections collected from control and Rptorf/f; CAGGCreER/+; ROSA26mTmG/+ mice at P10. Smooth muscle actin (SMA) expression was characteristic of myofibroblasts and phalloidin stained the actin filaments. (E). Quantification of the protein levels of RPS6 and phosphorylated RPS6 (p-RPS6) in lung lysates derived from control and Rptorf/f; CAGGCreER/+; ROSA26mTmG/+ lungs (n = 5 for each group). (F) Quantification of the relative ratio of mitochondrial DNA (mtDNA), 16S rRNA, and mitochondrially encoded NADH dehydrogenase 1 (mtND1), to nuclear DNA (nDNA), hexokinase 2 (Hk2), in lysates derived from control and Rptorf/f; CAGGCreER/+; ROSA26mTmG/+ lungs (n = 5 for each group). (G) Immunostaining of lung sections collected from control and Rptorf/f; CAGGCreER/+; ROSA26mTmG/+ mice at P10. MPC1 antibodies marked mitochondria; MTCO1 antibodies detected cytochrome c oxidase, the expression of which was controlled by Tfam. All values are mean ± SEM. p<0.05; **p<0.01; ***p<0.001 (unpaired Student’s t-test).
-
Figure 7—source data 1
Mean linear intercept, relative protein levels, and relative mitochondrial DNA (mtDNA)/nuclear DNA (nDNA) ratio.
- https://cdn.elifesciences.org/articles/68598/elife-68598-fig7-data1-v2.xlsx
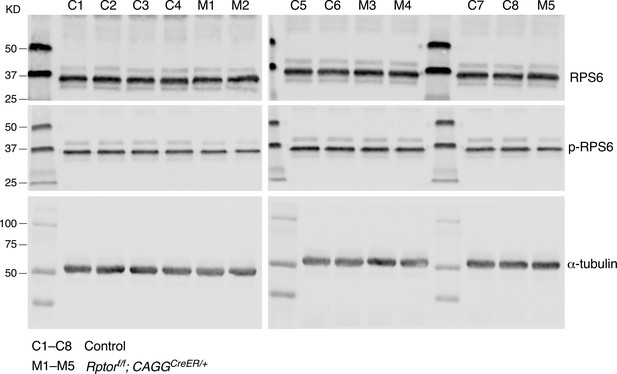
The ratio of p-RPS6 to RPS6 is reduced in the absence of Rptor.
Western blotting of lung lysates from control (C1–C8) and Rptor-deficient (M1–M5) mice at postnatal (P) day 10. Quantification of the ratio of p-RPS6 to RPS6 protein levels is shown in Figure 7E. The numbers indicate the positions of protein size standards.
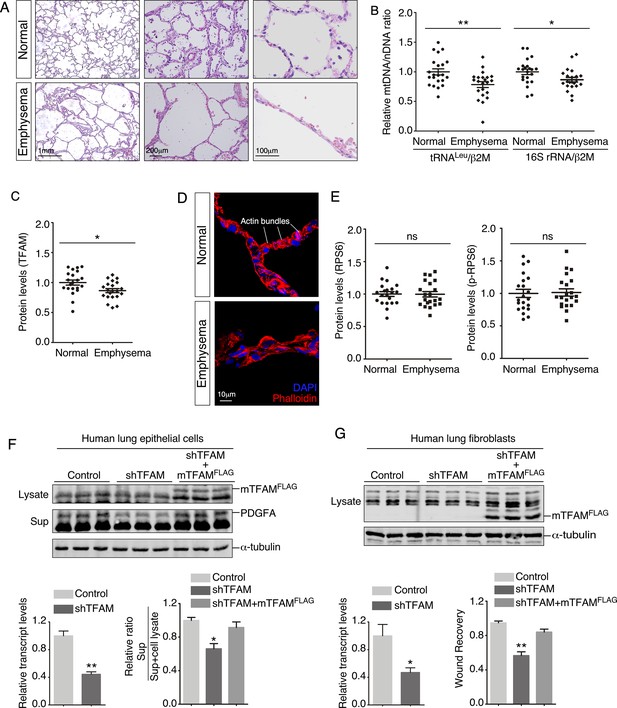
Lungs from emphysema patients exhibit a reduction in mitochondrial DNA and TFAM expression.
(A) Hematoxylin and eosin-stained lung sections of normal and emphysema patients. Disruption of alveoli in emphysema patients resulted in enlarged airspace with thin primary septa. (B) qPCR analysis of mitochondrial DNA (mtDNA) to nuclear DNA (nDNA) in lung lysates derived from normal and emphysema patients (n = 22 for each group). mtDNA-encoded tRNALeu and 16S rRNA and nDNA-encoded b2M (b2 microglobulin) were used in this study. The relative ratio of mtDNA to nDNA was calculated. (C) Quantification of TFAM protein levels in lung lysates derived from normal and emphysema patients (n = 21 for each group). (D) Immunostaining of lungs collected from control and emphysema patients. Phalloidin detected actin filaments. (E). Quantification of the protein levels of RPS6 and phosphorylated RPS6 (p-RPS6) in lung lysates derived from normal and emphysema patients (n = 21 for each group). (F) Western blot analysis of cell lysates and supernatants from human lung epithelial cells transduced with control constructs, constructs expressing shRNAs against human TFAM and constructs expressing mouse TFAMFLAG (mTFAMFLAG) as indicated. qPCR analysis revealed efficient knockdown of human TFAM transcripts. The amount of PDGFA released into the media was reduced in TFAM-knockdown cells compared to controls and was rescued by expression of mTFAMFLAG (n = 3 for each group). α-Tubulin served as a loading control. (G) Western blot analysis of cell lysates from human lung fibroblasts transduced with control constructs, constructs expressing shRNAs against human TFAM and constructs expressing mouse TFAMFLAG (mTFAMFLAG) as indicated. qPCR analysis revealed efficient knockdown of human TFAM transcripts. Wound recovery assays using control, TFAM-knockdown fibroblasts, and TFAM-knockdown fibroblasts expressing mTFAMFLAG were quantified. The migratory defect of fibroblasts was rescued by mTFAMFLAG expression (n = 3 for each group). α-Tubulin served as a loading control. All values are mean ± SEM. *p<0.05; **p<0.01; ns, not significant (unpaired Student’s t-test).
-
Figure 8—source data 1
Relative mitochondrial DNA (mtDNA)/nuclear DNA (nDNA) ratio, protein levels, relative transcript levels, quantification of PDGFA secretion, and quantification of wound recovery.
- https://cdn.elifesciences.org/articles/68598/elife-68598-fig8-data1-v2.xlsx
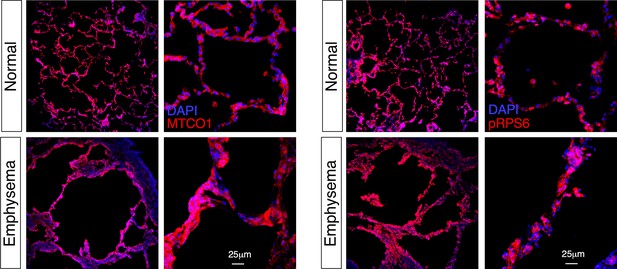
Mitochondria display inhomogeneous distribution in human lungs.
Immunostaining of human lungs from normal subjects and emphysema patients. Mitochondria labeled by MTCO1 showed inhomogeneous distribution in different cell types of normal lungs. Likewise, immunoreactivity of phosphorylated RPS6 (pRPS6), an indicator of mTORC1 activity, also displayed nonuniform distribution. Regional but not global reduction of MTCO1 was observed in lungs of emphysema patients.
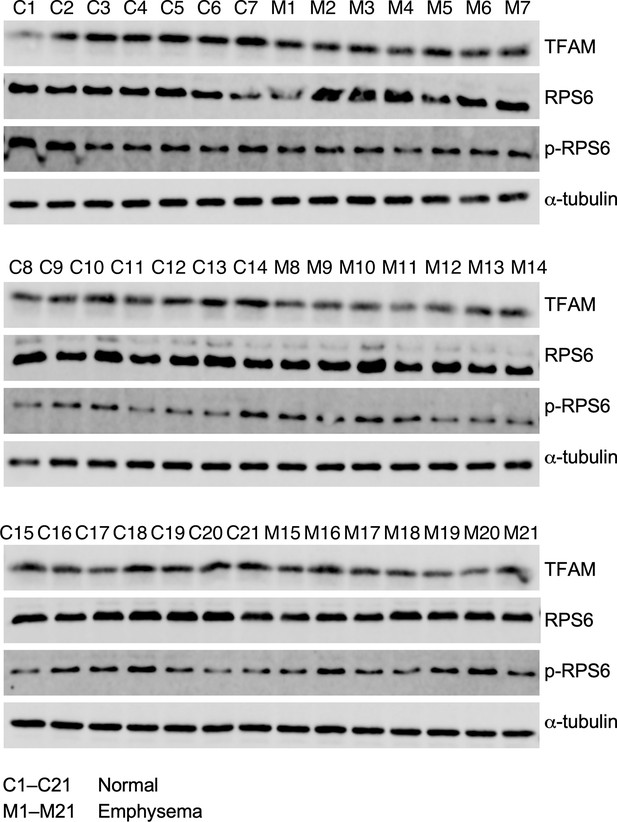
TFAM protein levels are decreased in emphysema patients.
Western blotting of lung lysates from normal (C1–C21) and emphysema (M1–M21) patients. Quantification of protein levels of TFAM, RPS6, and p-RPS6 is shown in Figure 8C and E.
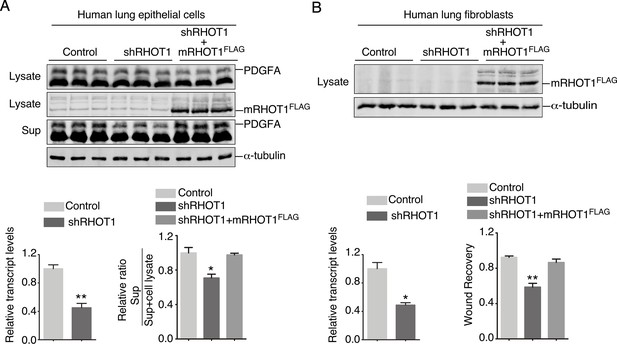
RHOT1 controls PDGF secretion in human lung epithelial cells and migration of human lung fibroblasts.
(A) Western blot analysis of cell lysates and supernatants from human lung epithelial cells transduced with control constructs, shRNA constructs against human RHOT1 and constructs expressing mouse RHOT1FLAG (mRHOT1FLAG) as indicated. qPCR analysis revealed efficient knockdown of human RHOT1 transcripts. The amount of PDGFA released into the media was reduced in RHOT1-knockdown cells compared to controls and was rescued by expression of mouse RHOT1FLAG (mRHOT1FLAG) (n = 3 for each group). α-Tubulin served as a loading control. (B) Western blot analysis of cell lysates and supernatants from human lung fibroblasts transduced with control constructs, shRNA constructs against human RHOT1, and constructs expressing mRHOT1FLAG as indicated. qPCR analysis revealed efficient knockdown of human RHOT1 transcripts. Wound recovery by control fibroblasts, RHOT1-knockdown fibroblasts, and RHOT1-knockdown fibroblasts expressing mRHOT1FLAG was quantified. The migratory defect was rescued by mRHOT1FLAG. α-Tubulin served as a loading control.
-
Figure 8—figure supplement 3—source data 1
Relative transcript levels, quantification of PDGFA secretion, and quantification of wound recovery.
- https://cdn.elifesciences.org/articles/68598/elife-68598-fig8-figsupp3-data1-v2.xlsx
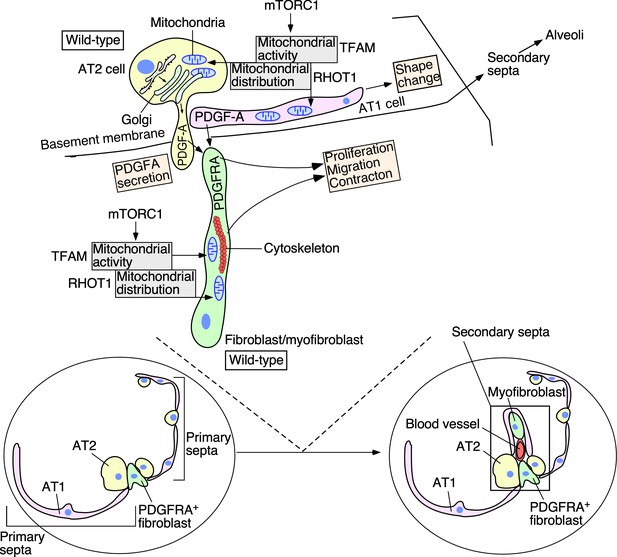
A model of regulating alveolar formation through mitochondrial activity and distribution.
The main players during alveolar formation are shown in the schematic diagram. Mitochondria display dynamic subcellular distribution in alveolar epithelial cells and mesenchymal fibroblasts/myofibroblasts. Tfam controls mitochondrial activity while Rhot1 regulates mitochondrial distribution. Mitochondrial activity and distribution in alveolar epithelial cells (type I [AT1] and type II [AT2]) contribute to secretion of the PDGF-A ligand. Reception of PDGF-A by mesenchymal fibroblasts/myofibroblasts is critical to fibroblast/myofibroblast proliferation and migration, a key step in secondary septation. Similarly, mitochondrial activity and distribution in fibroblasts/myofibroblasts are also required for fibroblast/myofibroblast contraction and migration, likely through powering the cytoskeleton. The mTORC1 pathway plays a central role in controlling mitochondrial function during alveolar formation. Specification of alveolar epithelial cells and fibroblasts/myofibroblasts was unaffected in mutant mouse lungs in which mitochondrial activity and distribution were perturbed. We propose that essential cellular processes have differential requirements of mitochondrial activity and distribution. Investigating how mitochondria control signaling pathways and cellular processes in vivo provide a new way to functionally define signaling pathways and cellular processes. We surmise that the regulatory circuitry mediated by mitochondria activity and distribution is also deployed during alveolar repair following lung injury and could contribute to the pathogenesis of chronic obstructive lung disease (COPD)/emphysema.
Tables
Reagent type (species) or resource | Designation | Source or reference | Identifiers | Additional information |
---|---|---|---|---|
Antibody | Anti-ACTA2 (mouse monoclonal) | Thermo Scientific Lab Vision | Cat# MS-113-P0; RRID:AB_64001 | IF (1:200) |
Antibody | Anti-CC10 (goat polyclonal) | Santa Cruz Biotechnology | Cat# sc-9773; RRID:AB_2183391 | IF (1:200) |
Antibody | Anti-DLP1 (mouse monoclonal) | BD Biosciences | Cat# 611113; RRID:AB_398424 | WB (1:2000) |
Antibody | Anti-phospho-DRP1 (Ser616) (rabbit polyclonal) | Cell Signaling Technology | Cat# 3455; RRID:AB_2085352 | WB (1:1000) |
Antibody | Anti-E-cadherin (rat monoclonal) | Invitrogen | Cat# 13-1900; RRID:AB_2533005 | IF (1:200) |
Antibody | Anti-GFP (chicken polyclonal) | Abcam | Cat# ab13970; RRID:AB_300798 | IF (1:200) |
Antibody | Anti-mouse HOPX (mouse monoclonal) | Santa Cruz Biotechnology | Cat# sc-398703; RRID:AB_2687966 | IF (1:100) |
Antibody | Anti-MPC1 (BRP44L) (rabbit polyclonal) | MilliporeSigma | Cat# HPA045119; RRID:AB_10960421 | IF (1:100) |
Antibody | Anti-MTCO1 [1D6] (mouse monoclonal) | Abcam | Cat# ab14705; RRID:AB_2084810 | IF (1:100) |
Antibody | Anti-NKX2.1 (rabbit monoclonal) | Epitomics | Cat# 2044-1; RRID:AB_1267367 | IF (1:100) |
Antibody | Anti-OPA1, clone 18 (mouse monoclonal) | BD Biosciences | Cat# 612606; RRID:AB_399888 | WB (1:2000) |
Antibody | Anti-PDGF receptor alpha (rabbit polyclonal) | Cell Signaling Technology | Cat# 3164; RRID:AB_2162351 | IF (1:150) |
Antibody | Anti-phospho-PDGF receptor α (Tyr754) (23B2) (rabbit monoclonal) | Cell Signaling Technology | Cat# 2992; RRID:AB_390728 | IF (1:100) |
Antibody | Anti-PECAM-1 (MEC 13.3) (rat monoclonal) | Santa Cruz Biotechnology | Cat# sc-18916; RRID:AB_627028 | IF (1:150) |
Antibody | Anti-prosurfactant protein C (proSP-C) (rabbit polyclonal) | MilliporeSigma | Cat# AB3786; RRID:AB_91588 | IF (1:200) |
Antibody | Anti-S6 ribosomal protein (54D2) (mouse monoclonal) | Cell Signaling Technology | Cat# 2317; RRID:AB_2238583 | IF (1:100); WB (1:2000) |
Antibody | Anti-phospho-S6 ribosomal protein (Ser235/236)(2F9) (rabbit monoclonal) | Cell Signaling Technology | Cat# 4856; RRID:AB_2181037 | IF (1:100); WB (1:2000) |
Antibody | Anti-T1α (hamster monoclonal) | Developmental Studies Hybridoma Bank | Cat# 8.1.1; RRID:AB_531893 | IF (1:200) |
Antibody | Anti-TFAM (rabbit polyclonal) | Proteintech | Cat# 22586-1-AP; RRID:AB_11182588 | WB (1:2000) |
Antibody | Anti-Rat TGN38 (sheep polyclonal) | Bio-Rad Laboratories | Cat# AHP499G; RRID:AB_2203272 | IF (1:100) |
Antibody | Anti-acetylated α-tubulin, clone 6-11B-1 (mouse monoclonal) | MilliporeSigma | Cat# T6793; RRID:AB_477585 | IF (1:200) |
Antibody | Anti-alpha-tubulin (mouse monoclonal) | Developmental Studies Hybridoma Bank | Cat# 12G10; RRID:AB_1157911 | WB (1:3000) |
Chemical compound, drug | ANTI-FLAG M2 Affinity Gel | Sigma-Aldrich | Cat# A2220; RRID:AB_10063035 | |
Chemical compound, drug | Biotin-XX Phalloidin | Molecular Probes | Cat# B7474 | |
Chemical compound, drug | DMEM | Mediatech | Cat# 10-013-CV | |
Chemical compound, drug | Fetal bovine serum (FBS) | Gibco | Cat# 10437-028 | |
Chemical compound, drug | Fibronectin | Corning | Cat# 354008 | |
Chemical compound, drug | Glutaraldehyde, 8% aqueous solution, EM grade | Electron Microscopy Sciences | Cat# 16000 | |
Chemical compound, drug | Insulin, transferrin, and selenium (ITS) | Gibco | Cat# 51300-044 | |
Chemical compound, drug | Paraformaldehyde, 16% solution, EM grade | Electron Microscopy Sciences | Cat# 15700 | |
Chemical compound, drug | Paraformaldehyde | Sigma-Aldrich | Cat# P6148 | |
Chemical compound, drug | Polyethylenimine (PEI) | Polysciences, Inc | Cat# 23966-2 | |
Chemical compound, drug | Penicillin/streptomycin | Gibco | Cat# 15070-063 | |
Chemical compound, drug | Protease Inhibitor Cocktail | Biotool | Cat# B14001 | |
Chemical compound, drug | Rhodamine-conjugated phalloidin | Molecular Probes | Cat# R415 | |
Chemical compound, drug | Tamoxifen | Toronto Research Chemicals | Cat# T006000 | |
Chemical compound, drug | TRIzol Reagent | Ambion | Cat# 15596018 | |
Cell line (Homo sapiens) | Human lung epithelial cells (1310 cell line) | John D. Minna (Ramirez et al., 2004) | ||
Cell line (H. sapiens) | Human lung airway fibroblasts | Stephen L. Nishimura (Araya et al., 2007) | ||
Commercial assay or kit | Click-iT EdUAlexa Fluor 488 Imaging Kit | Thermo Fisher | Cat# C10337 | |
Commercial assay or kit | Centriprep Ultracel YM-10 Centrifugal Filter Devices | MilliporeSigma | Cat# 4305 | |
Commercial assay or kit | Maxima First Strand cDNA Synthesis Kit | Thermo Scientific | Cat# K1641 | |
Commercial assay or kit | RNeasy Mini Kit | QIAGEN | Cat# 74104 | |
Commercial assay or kit | TSA Plus Cyanine 3 (Cy3) Fluorescein detection kit | PerkinElmer | Cat# NEL753001KT | |
Commercial assay or kit | Two-well culture insert | Ibidi | Cat# 80209 | |
Genetic reagent (Mus musculus) | CAGGCre-ERTM [B6.Cg-Tg(CAG-cre/Esr1*)5Amc/J] | The Jackson Laboratory | Stock# 004682; RRID:IMSR_JAX:004682 | |
Genetic reagent (M. musculus) | Cd4Cre [B6.Tg(Cd4-cre)1Cwi] | The Jackson Laboratory | Stock# 022071; RRID:MGI:3691126 | |
Genetic reagent (M. musculus) | Cx3cr1CreER [B6.129P2(C)-Cx3cr1tm4(tm2.1(cre/ERT2)Jung/J] | The Jackson Laboratory | Stock# 020940; RRID:IMSR_JAX:020940 | |
Genetic reagent (M. musculus) | Dermo1Cre [Twist2tm1.1(cre)Dor/J] | David Ornitz (Yu et al., 2003) | ||
Genetic reagent (M. musculus) | Foxp3Cre [Foxp3tm4(YFP/icre)Ayr/J] | The Jackson Laboratory | Stock# 016959; RRID:IMSR_JAX:016959 | |
Genetic reagent (M. musculus) | Lrpprcf [Lrpprctm1.1Lrsn/J] | Nils-Göran Larsson (Ruzzenente et al., 2012) | ||
Genetic reagent (M. musculus) | Miro1f [B6(Cg)-Rhot1tm2.1Jmsu/J] | The Jackson Laboratory | Stock# 031126; RRID:IMSR_JAX:031126 | |
Genetic reagent (M. musculus) | Pdgfaex4COIN [Pdgfaex4COIN] | ChristerBetsholtz (Andrae et al., 2014) | ||
Genetic reagent (M. musculus) | PdgfraCre [C57BL/6-Tg(Pdgfra-cre)1Clc/J] | The Jackson Laboratory | Stock# 013148; RRID:IMSR_JAX:013148 | |
Genetic reagent (M. musculus) | Rptorf [B6.Cg-Rptortm1.1Dmsa/J] | The Jackson Laboratory | Stock# 013188; RRID:IMSR_JAX:013188 | |
Genetic reagent (M. musculus) | ROSA26mTmG [Gt(ROSA)26Sortm4(ACTB-tdTomato,-EGFP)Luo/J] | The Jackson Laboratory | Stock# 007576; RRID:IMSR_JAX:007576 | |
Genetic reagent (M. musculus) | Sox9Cre [Sox9tm3(Cre)Crm] | Benoit de Crombrugghe (Akiyama et al., 2005) | ||
Genetic reagent (M. musculus) | Tfamf [B6.Cg-Tfamtm1.1Ncdl/J] | The Jackson Laboratory | Stock# 026123; RRID:IMSR_JAX:026123 | |
Biological sample (H. sapiens) | Human Emphysema/COPD patient tissue (deidentified) | Paul Wolters | ||
Recombinant DNA reagent | Lentiviral vector backbone for Pdgfa cloning, modified from pSECC | This paper | Refer to the ‘Lentivirus production and transduction’ | |
Recombinant DNA reagent | pSECC | Sánchez-Rivera et al., 2014 | AddgenePlasmid# 60820 | |
Recombinant DNA reagent | pMD2.G | A gift from Didier Trono | AddgenePlasmid# 12259 | |
Recombinant DNA reagent | psPAX2 | A gift from Didier Trono | AddgenePlasmid# 12260 | |
Software, algorithm | Prism | GraphPad | https://www.graphpad.com/ | |
Software, algorithm | ImageJ | National Institutes of Health (NIH) (https://imagej.nih.gov/ij/) | RRID:SCR_003070 |