Adult mouse fibroblasts retain organ-specific transcriptomic identity
Figures
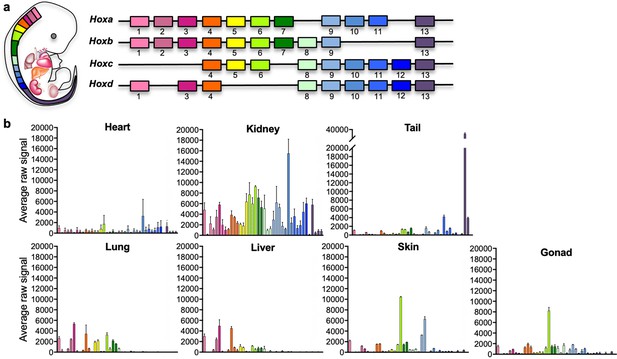
Positional code of organ fibroblasts.
(a) Murine Hox cluster code, showing proximally expressed Hox genes in pink and distal ones in purple. (b) Bar plots showing the average raw signal for all expressed Hox genes in organ-specific fibroblast samples. Data are mean ± standard error of the mean (SEM) of three biological replicates for each fibroblast type from the microarray analysis. Refer to Figure 1—figure supplements 1–2, Figure 1—source data 1, and Figure 1—source data 2.
-
Figure 1—source data 1
Microarray data: highly expressed genes common to all organ fibroblast populations, classified based on cellular process or cellular localization.
- https://cdn.elifesciences.org/articles/71008/elife-71008-fig1-data1-v2.xlsx
-
Figure 1—source data 2
Microarray data: average raw expression and standard errors of Hox code genes across all fibroblast samples from the microarray analysis (n = 3).
- https://cdn.elifesciences.org/articles/71008/elife-71008-fig1-data2-v2.xlsx
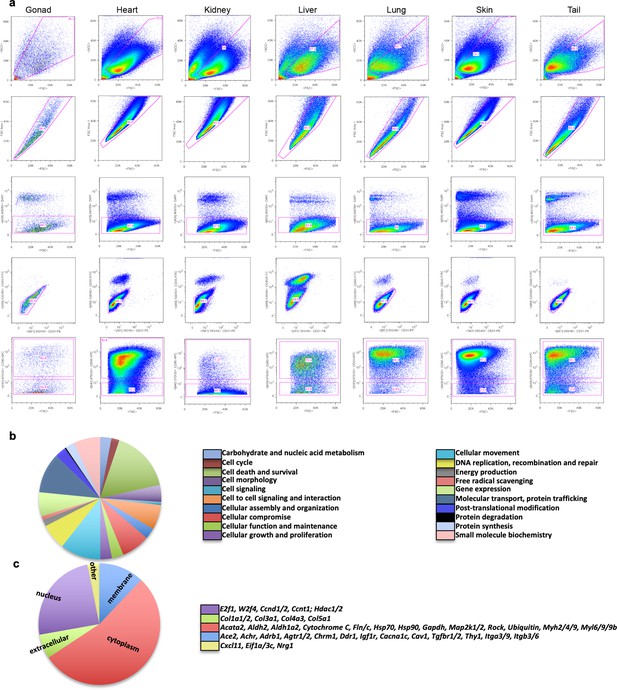
Isolation of fibroblasts from different organs and IPA analysis of common genes expressed across all organ fibroblasts.
(a) Representative FACS plot showing the gating strategy used to isolate CD45−, CD31−, CD90+ fibroblasts from different organs.(b, c) IPA analysis of the genes highly expressed by all fibroblast types in the microarray analysis. (b) Pie chart of the molecular pathways. (c) Pie chart of the cellular distribution of common highly expressed genes. The microarray analysis was performed on n = 3 biological replicates per fibroblast type. Refer to Figure 1—source data 1.
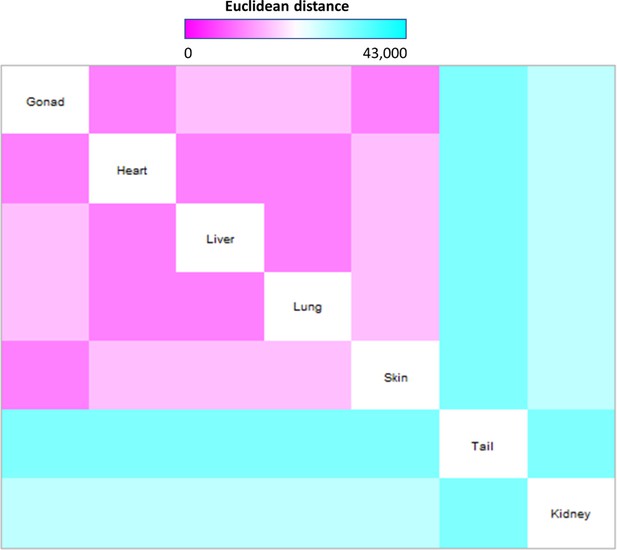
Pairwise comparison of Hox code across organ fibroblasts.
Heatmap plot showing the pairwise square Euclidean distances based on raw signal intensity of organ fibroblasts (n = 3). Smaller distance signifies closer transcriptional similarity.
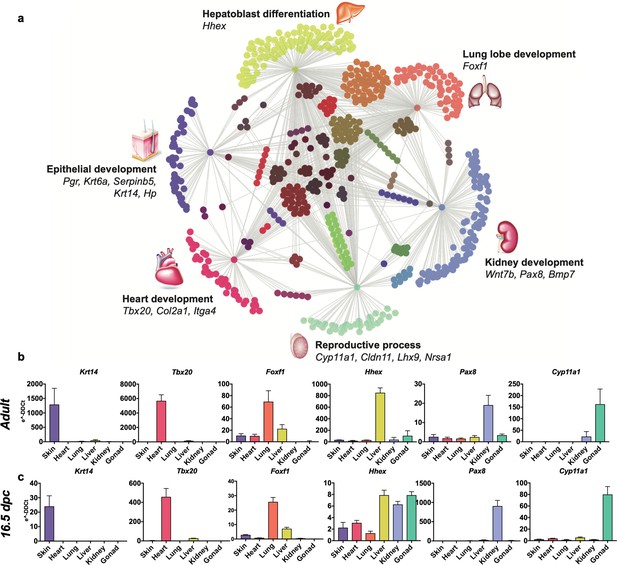
Embryological molecular signature of organ fibroblasts.
(a) Cytoscape representation of network of genes (dots) singularly expressed in organ fibroblasts (only one gray edge between the gene and the organ) or shared among organs (multiple gray edges linking the gene to several organs). Genes involved in organ development are highlighted. (b, c) Validation of the expression of selected organ-enriched, developmental related genes using qPCR on cultured organ-derived fibroblasts isolated from adult mice (top row) or E16.5 embryos (bottom row). Data are mean ± standard error of the mean (SEM) of the e−DDCt values, on three technical replicates of merged biological samples. The housekeeping gene is Hprt and the reference sample is tail fibroblasts. Refer to Figure 2—figure supplements 1–7, Figure 2—source data 1.
-
Figure 2—source data 1
Microarray data: expression of genes that were enriched by tenfold change or more in single organ fibroblasts compared to tail fibroblasts (n = 3).
- https://cdn.elifesciences.org/articles/71008/elife-71008-fig2-data1-v2.xlsx
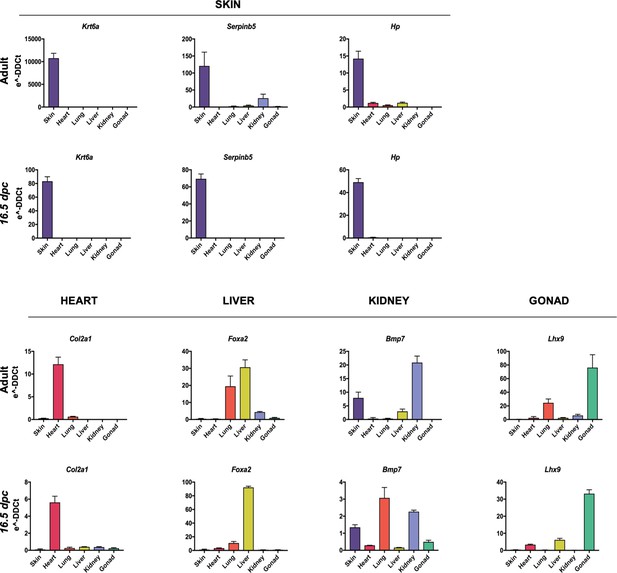
Embryological molecular signature of organ fibroblasts.
Additional validation of expression of organ-enriched, developmental related genes using qPCR on adult and embryonic derived fibroblasts. Data are mean ± standard error of the mean (SEM) on three technical replicates of merged biological samples.
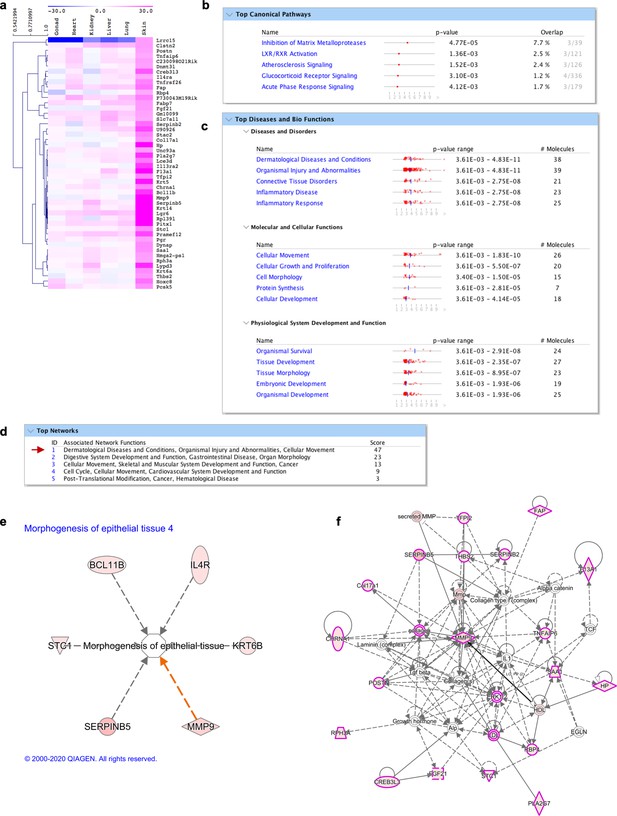
Molecular signature of skin fibroblasts.
(a) Heatmap highlighting genes differentially expressed over tenfold solely in skin fibroblasts. (b–f) IPA analysis on genes highly expressed in skin fibroblasts (shown in a). (b) Top 5 canonical pathways listed according to their p value and estimated percentage of overlap. (c) Top diseases and biological functions. (d) Top networks and associated functions. (e) Representation of a pathway related to skin embryonic development (morphogenesis of epithelial tissue) with related skin fibroblasts enriched genes. (f) Graphic representation of the top network (highlighted with a red arrow in d) overlaid with the expression of genes enriched in our dataset (circled in pink) associated with skin disease and function. Red arrows point to all skin/derma-related terms.
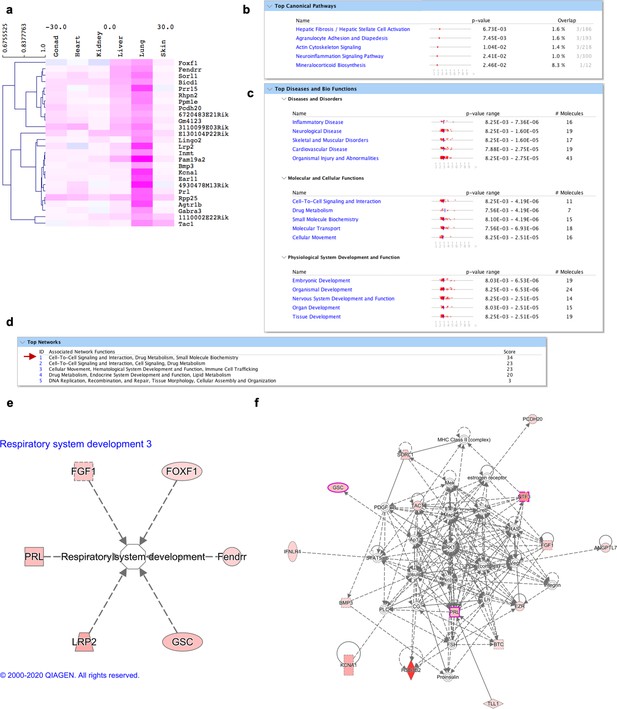
Molecular signature of lung fibroblasts.
(a) Heatmap highlighting genes differentially expressed over tenfold solely in lung fibroblasts. (b–f) IPA analysis on genes highly expressed in lung fibroblasts (shown in a). (b) Top 5 canonical pathways with p value and estimated percentage of overlap. (c) Top diseases and biological functions. (d) Top networks and associated functions. (e) Representation of a development associated pathway (respiratory system development) with related lung fibroblasts enriched genes. (f) Graphic representation of the top network (highlighted with a red arrow in d) overlaid with the expression of genes enriched in our dataset (circled in pink) associated with lung development and function.
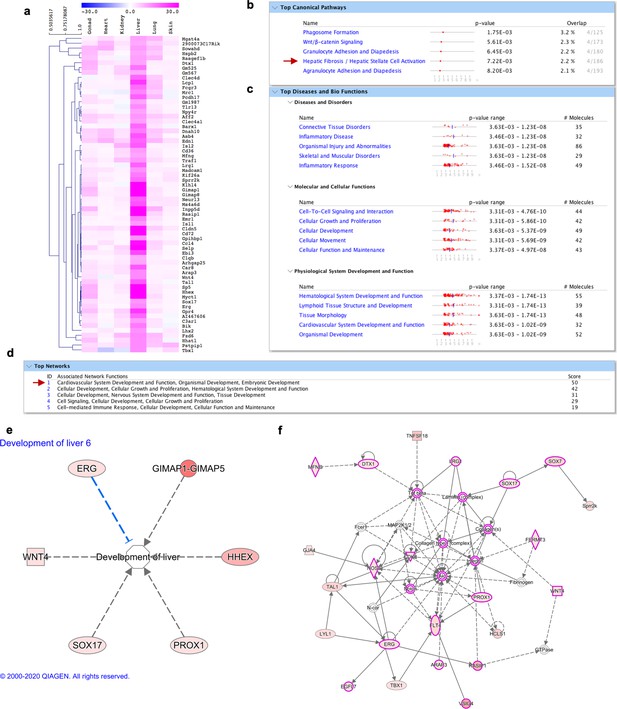
Molecular signature of liver fibroblasts.
(a) Heatmap highlighting genes differentially expressed over tenfold solely in liver fibroblasts. (b–f) IPA analysis on genes highly expressed in liver fibroblasts (shown in a). (b) Top 5 canonical pathways with p value and estimated percentage of overlap. (c) Top diseases and biological functions. (d) Top networks and associated functions. (e) Representation of a development associated pathway (development of liver) with related liver fibroblasts enriched genes. (f) Graphic representation of the top network (highlighted with a red arrow in d) overlaid with the expression of genes enriched in our dataset (circled in pink), associated with liver abnormal development and function.
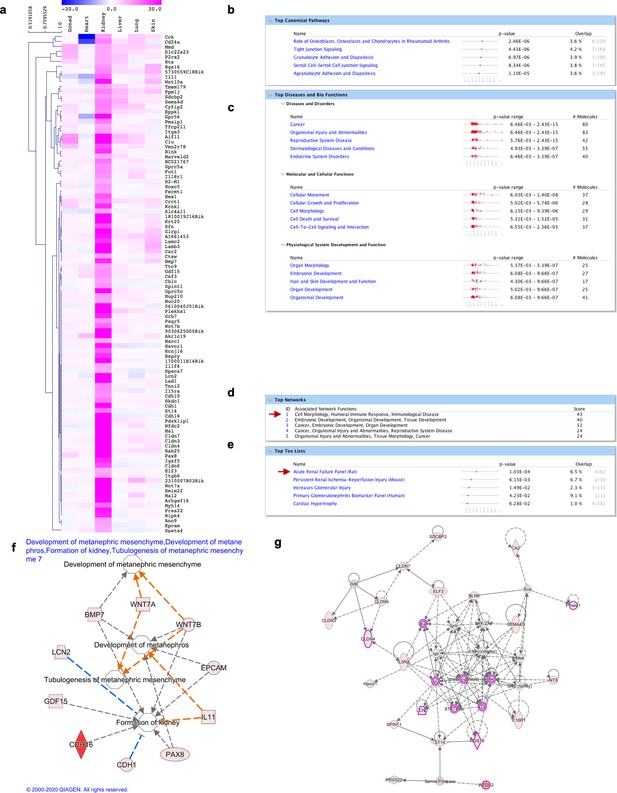
Molecular signature of kidney fibroblasts.
(a) Heatmap highlighting the genes differentially expressed over tenfold solely in kidney fibroblasts. (b–f) IPA analysis on genes highly expressed in kidney fibroblasts (shown in a). (b) Top 5 canonical pathways with p value and estimated percentage of overlap. (c) Top diseases and biological functions. (d) Top networks and associated functions. (e) Top toxicology list, including different genes associated with kidney injury. (f) Representation of development associated pathways (development of metanephric mesenchyme, metanephros, formation of kidneys) with related kidney fibroblasts enriched genes. (g) Graphic representation of the top network (highlighted with a red arrow in d) overlaid with the expression of genes enriched in our dataset (circled in pink), associated with kidney disease.
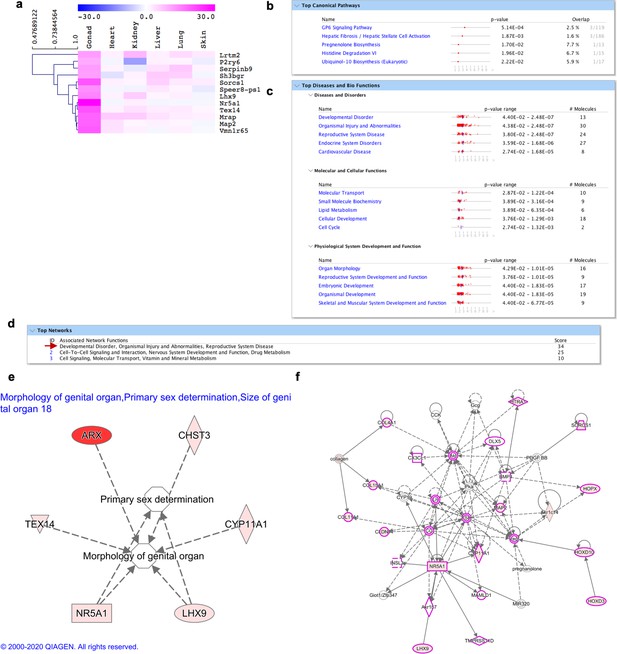
Molecular signature of gonad fibroblasts.
(a) Heatmap highlighting genes differentially expressed over tenfold solely in gonad (testis) fibroblasts. (b–f) IPA analysis on genes highly expressed in gonad fibroblasts (shown in a). (b) Top 5 canonical pathways with p value and estimated percentage of overlap. (c) Top diseases and biological functions. (d) Top networks and associated functions. (e) Representation of development associated pathways (morphology of genital organs, sex determination, size of genital organs), with related gonad fibroblasts enriched genes. (f) Graphic representation of the top network (highlighted with a red arrow in d) overlaid with the expression of genes enriched in our dataset (circled in pink), associated with reproductive system development and dysfunction.
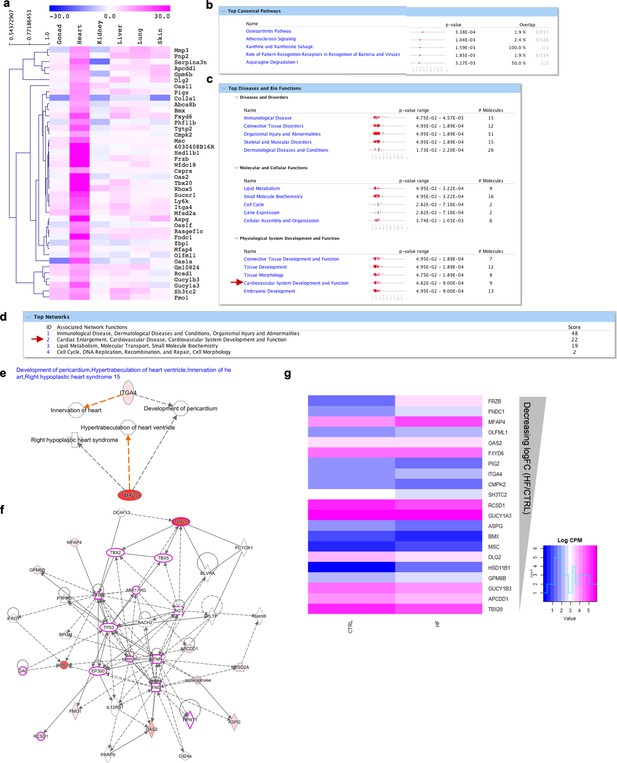
Molecular signature of cardiac fibroblasts.
(a) Heatmap highlighting the genes differentially expressed over tenfold solely in cardiac fibroblasts. (b–f) IPA analysis on genes highly expressed in cardiac fibroblasts (shown in a). (b) Top 5 canonical pathways with p value and estimated percentage of overlap. (c) Top diseases and biological functions. (d) Top networks and associated functions. (e) Representation of development associated pathways (development of pericardium, hypertrabeculation, innervation, hypoplastic heart syndrome) with related heart fibroblasts enriched genes. (f) Graphic representation of the second top network (highlighted with a red arrow in d) overlaid with the expression of genes enriched in our dataset (circled in pink), associated with cardiac diseases and disorders. (g) Heatmap showing the expression of cardiac fibroblasts-enriched genes in human left ventricular biopsies from healthy (N = 5) and chronic ischemic heart failure patients (N = 5). Refer to Figure 2—figure supplement 7—source data 1.
-
Figure 2—figure supplement 7—source data 1
Expression of cardiac fibroblast-enriched genes in human left ventricular biopsies from healthy and chronic ischemic heart failure patients.
- https://cdn.elifesciences.org/articles/71008/elife-71008-fig2-figsupp7-data1-v2.xlsx
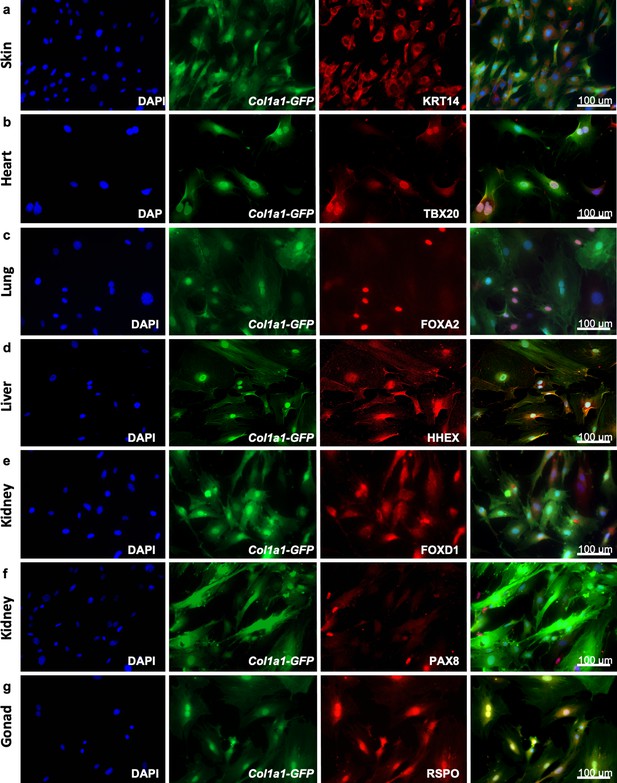
Adult fibroblasts express organ-specific transcription factors.
Immunocytochemistry for development-related organ-enriched markers (KRT14, TBX20, FOXA2, HHEX, FOXD1, PAX8, and RSPO) on adult fibroblasts obtained from different organs of Col1a1-GFP mice and cultured for 5 days: (a) Skin fibroblasts stained with a mouse monoclonal anti-KRT14 antibody; (b) heart fibroblasts stained with a mouse monoclonal anti-TBX20 antibody; (c) lung fibroblasts stained with a rabbit monoclonal anti-FOXA2 antibody; (d) liver fibroblasts stained with a rabbit monoclonal anti-HHEX antibody; (e) kidney fibroblasts stained with a rabbit polyclonal anti-FOXD1 antibody; (f) kidney fibroblasts stained with a mouse monoclonal anti-PAX8 antibody; (g) testis fibroblasts stained with a goat polyclonal anti-RSPO1 antibody. As secondary antibodies, goat anti-mouse Alexa Fluor 568 was used for (a, b), (f); goat anti-rabbit Alexa Fluor 555 for (c, d) and donkey anti-goat Alexa Fluor 568 for (f). Representative images from three independent experiments on three biological replicates. Scale bar = 100 µm. Refer to Figure 3—figure supplement 1.
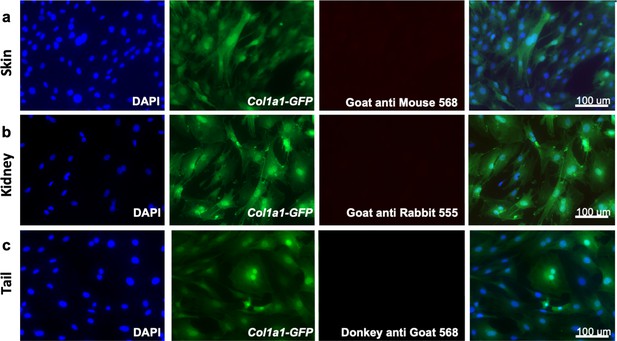
Adult fibroblasts express organ-specific transcription factors.
Negative controls were obtained by staining with secondary antibodies only on Col1a1-GFP + fibroblasts cultured for 5 days. Representative images of the staining with the secondary goat anti-mouse Alexa 568 on skin fibroblasts (a); goat anti-rabbit Alexa 555 on kidney fibroblasts (b); donkey anti goat Alexa 568 on tail fibroblasts (c). Representative images from three independent experiments on three biological replicates. Scale bar = 100 µm.
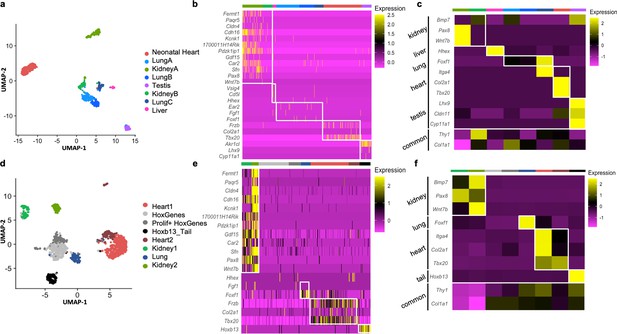
Analysis of organ-specific signatures at the single-cell level.
(a–c) Reanalysis of the Mouse Cell Atlas stromal cell dataset. (a) Uniform Manifold Approximation and Projection (UMAP) visualization of selected stromal cell populations (682 cells). (b) Heatmap representing the expression of top-organ-specific genes, identified from the bulk RNAseq comparison, on individual cells in the single-cell RNAseq (scRNAseq) dataset. (c) Heatmap showing the average expression per population of organ development-related genes (same as shown in Figure 3). (d–f) scRNAseq analysis of mixed cultured stromal cells of different origins. (d) UMAP visualization of the captured cells. (e) Heatmap representing the expression of top-organ-specific genes, identified from the bulk RNAseq comparison, on individual cells. (c) Heatmap showing the average expression per population of organ development-related genes (same as shown in Figure 3). Refer to Figure 4—figure supplements 1–2, Figure 4—source data 1, and Figure 4—source data 2.
-
Figure 4—source data 1
Analysis of the stromal cell aggregate from the Mouse Cell Atlas: markers genes per each population, and markers identified by pairwise comparison of the two kidney and three lung populations.
- https://cdn.elifesciences.org/articles/71008/elife-71008-fig4-data1-v2.xlsx
-
Figure 4—source data 2
Analysis of in-house single-cell RNAseq (scRNAseq) data of merged cultured fibroblasts from different organs: markers genes per each population, and markers identified by pairwise comparison of the two kidney and two cardiac populations.
- https://cdn.elifesciences.org/articles/71008/elife-71008-fig4-data2-v2.xlsx
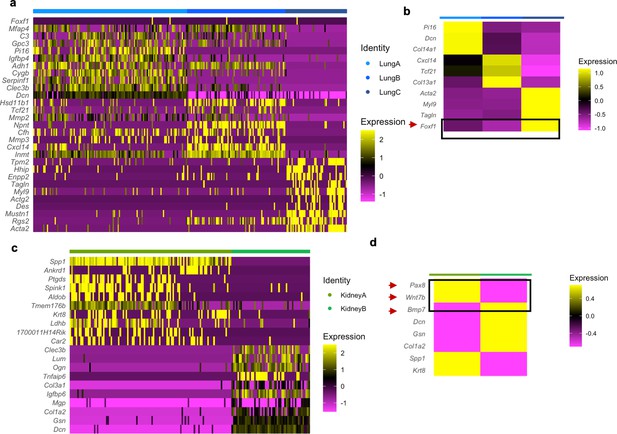
Analysis of the organ-specific fibroblasts heterogeneity in freshly isolated cells at single-cell level.
Data derived from the reanalysis of the ‘Mouse Cell Atlas’ stromal cell dataset. (a) Heatmap showing the top differentially expressed genes in each cell of the subcluster Lung A–B–C. Genes were identified by pairwise differential expression (Lung C versus Lung A, Lung C versus Lung B, and Lung B versus Lung A). (b) Heatmap showing the average expression per subpopulation of key defining gene and the lung development-related genes (Foxf1). (c) Heatmap showing the top differentially expressed genes in Kidney A–B. Genes were identified by pairwise differential expression. (d) Heatmap showing the average expression per subpopulation of key defining genes and the kidney development-related genes (Pax8, Wnt7b, and Bmp7).
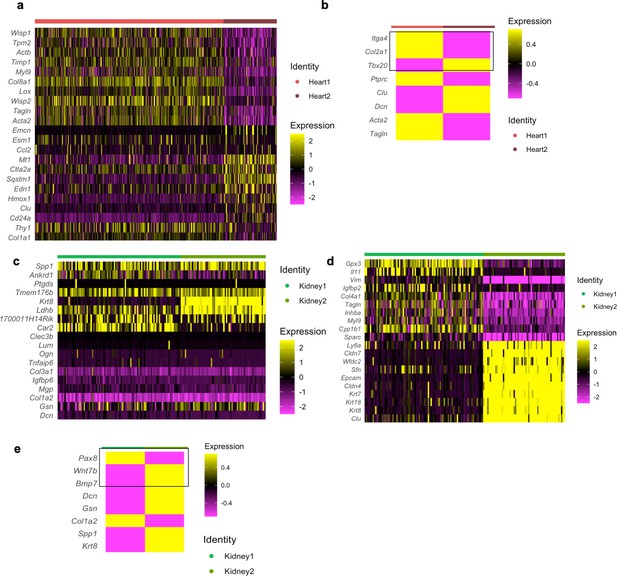
Analysis of the organ-specific fibroblast heterogeneity in cultured cells at single-cell level.
Data derived from cultured fibroblast single-cell RNAseq (scRNAseq) data generated in this manuscript. (a) Heatmap showing top differentially expressed genes between Heart 1 and 2. (b) Heatmap showing the average expression per subpopulation of key defining genes and the heart development-related genes (Itga4, Col2a1, and Tbx20). (c) Heatmap showing the expression of genes identified by pairwise differential expression analysis of freshly isolated kidney fibroblasts for comparison (same as in Figure 4—figure supplement 1c). (d) Heatmap showing top differentially expressed genes between Kidney 1 and 2. (e) Heatmap showing the average expression per subpopulation of key defining genes and the kidney development-related genes (Pax8, Wnt7b, and Bmp7).
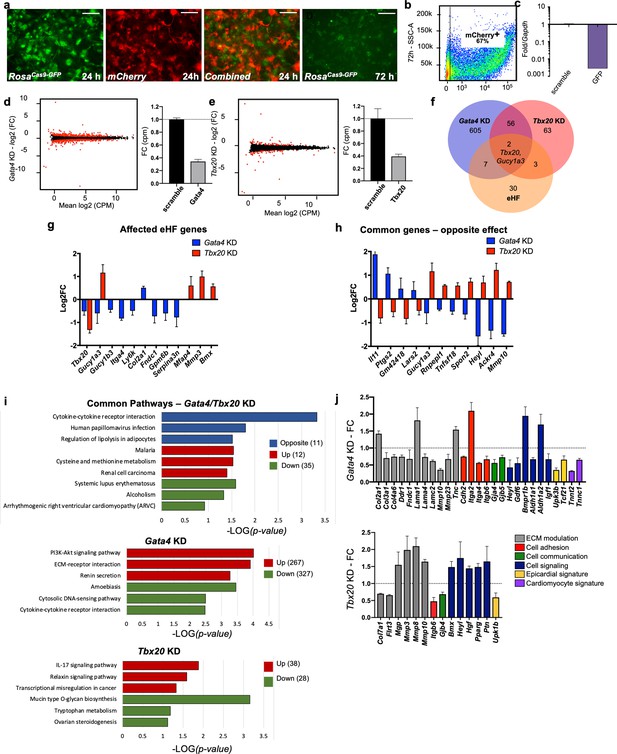
In vitro knockdown of core cardiac transcription factors.
(a) Images of adult cardiac fibroblasts derived from ROSACas9-GFP mice, 24 and 72 hr post-transfection with two guide RNAs for GFP and CleanCap mCherry mRNA. (b) Flow cytometry plot showing the expression on mCherry in 67% of transfected cells (representative image of three independent experiments). (c) Relative quantification qPCR of GFP, normalized by Gapdh expression, in cells transfected with scrambled or GFP guide RNAs. (d, e) Volcano plots showing genes differentially expressed in Gata4 (left) and Tbx20 wns (right). Fold change in expression of Gata4 (left) and Tbx20 (bottom) in cells transfected with specific gRNAs versus scramble RNA, quantified through RNA sequencing. (f) Venn diagram showing the overlap among genes affected by Gata4 (Gata4KD) or Tbx20 (Tbx20KD) knockdown and number of genes upregulated by tenfold or more in heart fibroblasts (eHF) compared to other organs. (g) Plot showing changes in expression of eHF genes affected by Gata4 (in blue) or Tbx20 (in red) knockdown. (h) Genes regulated by both Gata4 and Tbx20 in opposing manner. (i) KEGG pathway analyses. Top panel: 58 genes affected in both Tbx20 and Gata4 knockdown; middle panel: 594 genes affected by Gata4 knockdown; bottom panel: 66 genes affected by Tbx20 knockdown. Blue – pathway changed in opposite directions, red – upregulated pathways, and green – downregulated pathways. (j) Hand-picked genes illustrate alterations in processes known to affect the cardiac fibrotic response for Gata4 or Tbx20 knockdown. All data are represented as fold changes over scrambled control (average ± standard error of the mean [SEM]; d–j) from bulk RNAseq of three biological replicates per condition. Selected significant genes have an FDR < 0.05. Refer to Figure 5—source data 1.
-
Figure 5—source data 1
CRISPR-Cas9 experiments: sequence of the guide RNAs; differentially expressed genes between Tbx20KD and Gata4 KD and corresponding controls.
KEGG pathways analysis.
- https://cdn.elifesciences.org/articles/71008/elife-71008-fig5-data1-v2.xlsx
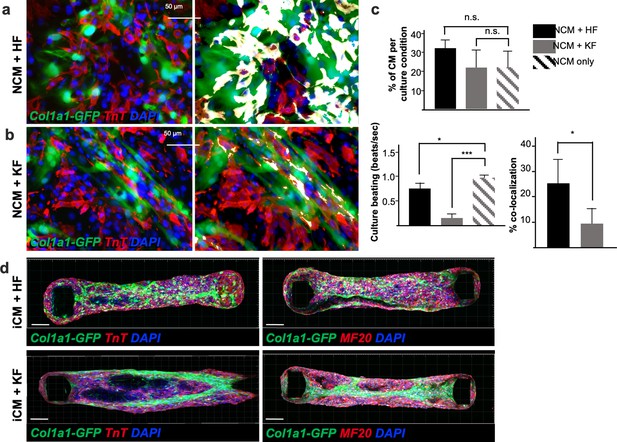
Adult fibroblasts retain tissue-specific function in vitro: impaired neonatal cardiomyocyte beating in presence of kidney-derived fibroblasts.
Immunocytochemistry for TnT on 2D coculture of neonatal ventricular cardiomyocytes (NCM, TnT+ in red) with either adult cardiac fibroblasts (HF) in (a) or kidney fibroblasts (KF) in (b), isolated from Col1a1-eGFP mice (in green). Nuclei are labeled with 4′,6-diamidino-2-phenylindole (DAPI) (in blue). The right panel shows colocalization of the two cell types (green+ and red+) in white. (c) Quantifications of the percentage of cardiomyocytes per culture condition (top), beating of the 2D cultures expressed in beats per second (bottom left) and percentage of colocalization (bottom right). (d) Confocal Z-stack images reconstructed with Imaris, of cardiac microtissues constituted of 85% hiPSCs derived cardiomyocytes (iCM) and 15% of either cardiac (HF) or kidney (KF) fibroblasts, stained for TnT (in red, left panels) or MF20 (in red right panels). The adult fibroblasts were isolated from Col1a1-eGFP mice (in green), nuclei stained with DAPI (in red). Forty-eight organoids were generated per each fibroblasts cell type. Scale bar = 50 μm. All data in (c) are mean ± standard error of the mean (SEM) on three independent experiments, p values were calculated by two-sample t-test; * = p<0.05, *** = p<0.005 Refer to Video 1 and Video 2.
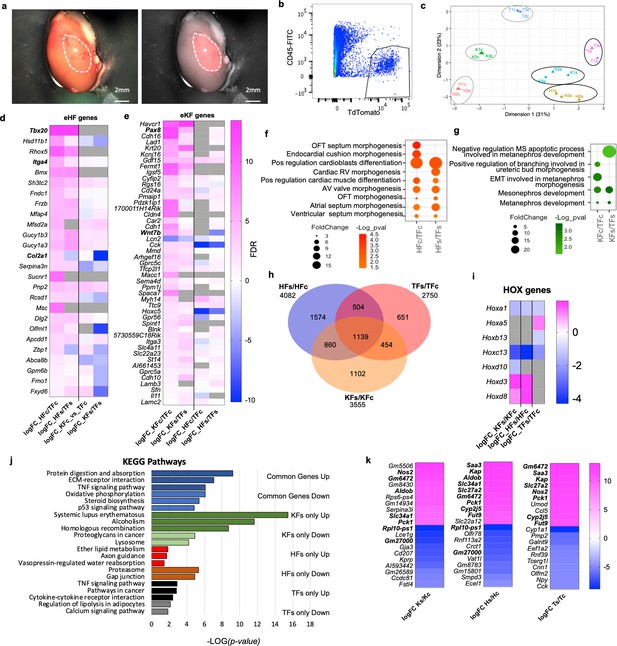
Fibroblast tissue-specific response to in vivo ectopic transplantation under the kidney capsule.
(a) Representative images of a dissected kidney to highlight the area where TdTomato+ cells were transplanted; brightfield image on the left; brightfield overlaid with the fluorescence image acquired in the red channel on the right. (b) Flow cytometry plot showing the gating strategy used to isolate live CD45−;TdTomato+ cells 3 days post-transplantation in the kidney capsule. (c) Multidimensional scaling plot calculated on the top 500 genes postnormalization to visualize the transcriptomic similarity among all samples. (d–h) Comparison of HF and KF gene expression to TF in culture (HFc/TFc, KFc/KFc) and post-transplant in the kidney capsule (HFs/TFs, KFs/KFs). (d) Heatmap showing expression of significantly regulated eHF genes in the four conditions. (e) Heatmap showing the expression of eKF genes in the four conditions. (f) Dot plot indicating GO terms associated with cardiac development, identified from the DAVID database analysis of HFs or HFc-enriched genes. (g) Dot plot indicating the GO terms associated with cardiac development, identified from the DAVID database analysis of KFs or KFc-enriched genes. (h–k) Analysis of the differential expression by experimental condition: transplanted cells versus cells in culture HFs/HFc, TFs/TFc, and KFs/KFc. (h) Venn diagram showing the significant differentially regulated genes (FDR < 0.05) in the three comparison sets. Only genes with a logFC >1 or <−1 were considered. (i) Heatmap of differentially regulated Hox genes. (j) Bar plot of the KEGG pathway analysis on the common regulated genes (light blue – downregulated, dark blue – upregulated), and genes uniquely modulated in KF (dark green – upregulated, light green – downregulated), HF (red – upregulated, brown – downregulated), and TF (black – upregulated, gray – downregulated). (k) Heatmaps showing the top 10 upregulated and top 10 downregulated genes for each dataset. In bold are the genes shared in two different sets of comparisons. Data are means of three biological replicates per condition. All the heatmaps (d, e, j, k) The average log fold change. For (d, e) Genes were ordered based on the FDR (smaller to larger value) of the comparison in the first column, HFc/TFc and KFc/TFc, respectively. Dot plots (f, g) and bar plot (j) data were organized based on the log transformation of the p values (−log(p value)). eKF – kidney-fibroblast-enriched genes, same as shown in Figure 2—figure supplement 5, eHF – heart-fibroblast-enriched genes, same as shown in Figure 2—figure supplement 7, KFs – transplanted kidney fibroblasts, HFs – transplanted heart fibroblasts, TFs – transplanted tail fibroblasts, KFc – kidney fibroblasts in culture, HFc – heart fibroblasts in culture, and TFc – tail fibroblasts in culture. Refer to Figure 7—figure supplement 1.
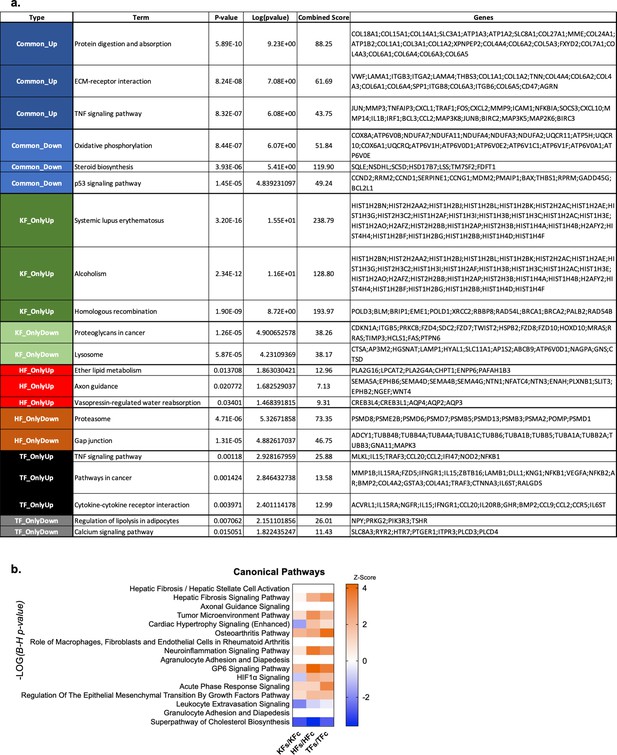
Analysis of organ fibroblast-specific responses to transplant under the kidney capsule.
(a) Table showing genes associated to the top KEGG pathways in Figure 7j, (b) canonical pathways identified through Ingenuity Pathway Analysis of differentially expressed genes, ordered by significance (−LOG of the B–H corrected p value) and colored by the activation z-score predicted for the three comparisons HFs/HFc, TFs/TFc, and KFs/KFc.
Videos
Coculture of adult cardiac fibroblasts with neonatal ventricular cardiomyocytes.
×20 magnification.
Coculture of adult kidney fibroblasts with neonatal ventricular cardiomyocytes.
×20 magnification.
Additional files
-
Transparent reporting form
- https://cdn.elifesciences.org/articles/71008/elife-71008-transrepform1-v2.pdf
-
Supplementary file 1
Sequence of all the qPCR primers used in the study.
- https://cdn.elifesciences.org/articles/71008/elife-71008-supp1-v2.xlsx