Microbiome: What can we learn from honey bees?
The gut of an animal contains a staggering amount of microbial diversity (Sankar et al., 2015). However, the species present are often members of only a few taxonomic groups and therefore tend to share many metabolic and physiological features. This observation has fascinated microbial ecologists for decades, as it has long been believed that species that are similar can only coexist if they avoid directly competing for shared resources (Gause, 1934; Hardin, 1960; Schoener, 1974; Volterra, 1926).
Recently, several theories have been put forward to explain how related species of bacteria are able to coexist in complex communities such as the gut microbiota of animals (Caetano et al., 2021; Erez et al., 2020; Goyal et al., 2018). This has led to the hypothesis that a process called resource partitioning – that is, when different species use resources in different ways to avoid competition – allows similar bacterial species to live together in a single community. However, finding an experimental system where it is possible to disentangle the confounding effects of diet, host, and microbial interactions is deceptively difficult. Now, in eLife, Philipp Engel and co-workers from the University of Lausanne and ETH Zürich – including Silvia Brochet as first author – report a new model for studying how dietary resources regulate microbial communities in the gut of honey bees (Brochet et al., 2021).
The Western honey bee, Apis mellifera, has several advantages as a model system for studying the coexistence of related microbes. First, its diet consists of pollen and nectar (a mix of simple sugars, complex carbohydrates and proteins) which can be easily replicated in the laboratory. Second, 95% of the bacterial species in their gut belong to the same family which is called Lactobacillus Firm-5 (Kwong and Moran, 2016). Most of these microbial communities contain several genetically distinct Firm-5 species which reside in the bee’s rectum, where they consume a diet of pollen (Ellegaard and Engel, 2019).
To find out how closely related bacteria are maintained in the gut, Brochet et al. created an artificial microbial community that contains four Firm-5 species that are commonly found in all Western honey bees. These species were then grown in the guts of live honey bees that had been depleted of their gut microbiome or cultured in the laboratory. The experiments showed that the four species coexisted when the honey bees were fed a diet of pollen but not simple sugar, and this effect was also observed in vitro. On a diet of simple sugar, one species outcompeted all the others; the other three species reached higher population densities when they were grown on their own on pollen or sugars instead of in a community. These results suggest that competition for resources shapes how the different species behave in the community, but these distinct behaviors allow the bacteria to coexist when grown on pollen.
To reveal the mechanisms underlying this coexistence, Brochet et al. combined transcriptomics and metabolomics to investigate how each species consumed the different nutrients derived from pollen. Surprisingly, despite all four species having a similar genetic make-up, they rarely activated the same genes, suggesting that transcriptional regulation may constrain the bacteria from using the resources in pollen in the same way. This was supported by metabolomics which showed that each species consumed a different composition of metabolites: about a third of measured metabolites were consumed by only one species, while a third were consumed by more than one, and the last third were consumed by all four. Furthermore, bacterial species that used the same resources often consumed these at different rates.
Together, these experiments demonstrate that species in the Firm-5 community consume distinct but overlapping profiles of nutrients derived from the pollen diet of bees (Figure 1). This supports the idea that resource partitioning allows closely related microbes to coexist in the gut. A more unexpected observation is the extent to which species can overlap with respect to their functional gene content and still live together. This result highlights the importance of incorporating knowledge of transcriptional regulation and cellular physiology when studying the interactions of closely related bacteria.
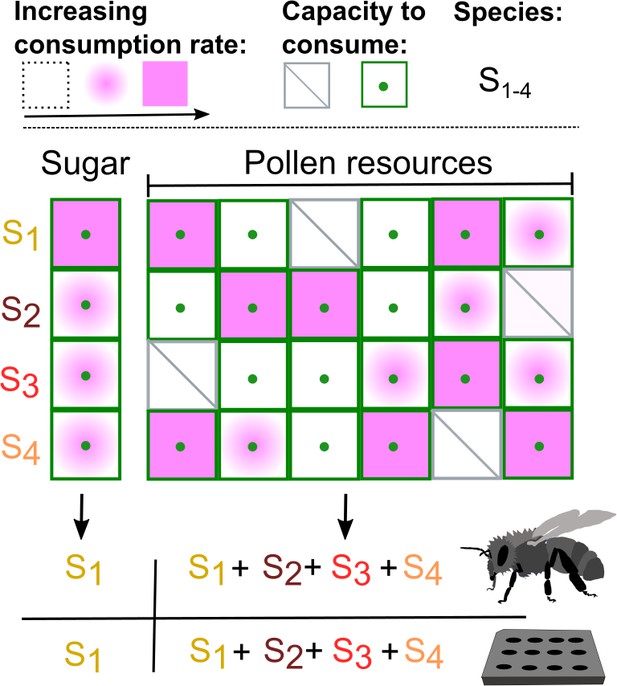
How a diet of pollen allows closely related species of bacteria to coexist in the gut of honey bees.
Brochet et al. created an artificial microbial community made up of four species that are commonly found in the gut of honey bees (S1, S2, S3, S4) and studied the growth of this model community in vivo (bee symbol) and in vitro. When grown with just one resource (sugar) available, one species (S1 in this instance) consumed the resource at a faster rate than the other species (see key at top left), even though all four species were capable of consuming sugar (as indicated by green dots). However, pollen offers multiple nutrients (as represented by the six columns in the figure), which the four species of bacteria consume in different ways. For example, S3 is unable to consume the nutrient represented by column 1 (indicated by a diagonal line), but can consume the nutrients represented by the other five columns: moreover, it consumes some nutrients at a higher rate than other species. If the consumption profiles of the four species complement each other (as is the case for the four species studied), they can coexist when grown in the gut of honey bees fed a diet of pollen or when cultured on pollen in the laboratory.
The study by Brochet et al. opens several exciting avenues for future study. The system could be used to measure the degree of diversity required for multiple species to live in a single community: studies that systematically increase species-level diversity are likely to provide a sense of the ‘upper bound’ for this system. In addition, the in vivo and in vitro bee gut models provide an opportunity to examine how microbes with overlapping resource preferences behave in a community.
Further characterization of other Firm-5 species is needed to address which resource preferences and consumption behaviors are more ecologically stable than others. In addition, it would be interesting to compare resource partitioning in the bee gut to culture-based models of competition among closely related bacteria, such as Bacteroidetes in the human gut microbiota (Tuncil et al., 2017); this may reveal generic strategies of resource partitioning in gut microbial communities. Importantly, the establishment of the Firm-5 model presents an exciting opportunity for ‘cross-pollination’ between theory and experiment to understand the link between consumption and composition in gut microbial communities.
References
-
Genomic diversity landscape of the honey bee gut microbiotaNature Communications 10:446.https://doi.org/10.1038/s41467-019-08303-0
-
The competitive exclusion principleScience 131:1292–1297.https://doi.org/10.1126/science.131.3409.1292
-
Gut microbial communities of social beesNature Reviews Microbiology 14:374–384.https://doi.org/10.1038/nrmicro.2016.43
-
The human gut microbiome, a taxonomic conundrumSystematic and Applied Microbiology 38:276–286.https://doi.org/10.1016/j.syapm.2015.03.004
Article and author information
Author details
Publication history
- Version of Record published: August 31, 2021 (version 1)
Copyright
© 2021, Schwartzman
This article is distributed under the terms of the Creative Commons Attribution License, which permits unrestricted use and redistribution provided that the original author and source are credited.
Metrics
-
- 2,093
- views
-
- 182
- downloads
-
- 0
- citations
Views, downloads and citations are aggregated across all versions of this paper published by eLife.
Download links
Downloads (link to download the article as PDF)
Open citations (links to open the citations from this article in various online reference manager services)
Cite this article (links to download the citations from this article in formats compatible with various reference manager tools)
Further reading
-
- Ecology
Spatial and temporal associations between sympatric species underpin biotic interactions, structure ecological assemblages, and sustain ecosystem functioning and stability. However, the resilience of interspecific spatiotemporal associations to human activity remains poorly understood, particularly in mountain forests where anthropogenic impacts are often pervasive. Here, we applied context-dependent Joint Species Distribution Models to a systematic camera-trap survey dataset from a global biodiversity hotspot in eastern Himalayas to understand how prominent human activities in mountain forests influence species associations within terrestrial mammal communities. We obtained 10,388 independent detections of 17 focal species (12 carnivores and five ungulates) from 322 stations over 43,163 camera days of effort. We identified a higher incidence of positive associations in habitats with higher levels of human modification (87%) and human presence (83%) compared to those located in habitats with lower human modification (64%) and human presence (65%) levels. We also detected a significant reduction of pairwise encounter time at increasing levels of human disturbance, corresponding to more frequent encounters between pairs of species. Our findings indicate that human activities can push mammals together into more frequent encounters and associations, which likely influences the coexistence and persistence of wildlife, with potential far-ranging ecological consequences.
-
- Ecology
The success of an organism depends on the molecular and ecological adaptations that promote its beneficial fitness. Parasitoids are valuable biocontrol agents for successfully managing agricultural pests, and they have evolved diversified strategies to adapt to both the physiological condition of hosts and the competition of other parasitoids. Here, we deconstructed the parasitic strategies in a highly successful parasitoid, Trichopria drosophilae, which parasitizes a broad range of Drosophila hosts, including the globally invasive species D. suzukii. We found that T. drosophilae had developed specialized venom proteins that arrest host development to obtain more nutrients via secreting tissue inhibitors of metalloproteinases (TIMPs), as well as a unique type of cell—teratocytes—that digest host tissues for feeding by releasing trypsin proteins. In addition to the molecular adaptations that optimize nutritional uptake, this pupal parasitoid has evolved ecologically adaptive strategies including the conditional tolerance of intraspecific competition to enhance parasitic success in older hosts and the obligate avoidance of interspecific competition with larval parasitoids. Our study not only demystifies how parasitoids weaponize themselves to colonize formidable hosts but also provided empirical evidence of the intricate coordination between the molecular and ecological adaptations that drive evolutionary success.