A non-bactericidal cathelicidin provides prophylactic efficacy against bacterial infection by driving phagocyte influx
Abstract
The roles of bactericidal cathelicidins against bacterial infection have been extensively studied. However, the antibacterial property and mechanism of action of non-bactericidal cathelicidins are rarely known. Herein, a novel naturally occurring cathelicidin (PopuCATH) from tree frog (Polypedates puerensis) did not show any direct anti-bacterial activity in vitro. Intriguingly, intraperitoneal injection of PopuCATH before bacterial inoculation significantly reduced the bacterial load in tree frogs and mice, and reduced the inflammatory response induced by bacterial inoculation in mice. PopuCATH pretreatment also increased the survival rates of septic mice induced by a lethal dose of bacterial inoculation or cecal ligation and puncture (CLP). Intraperitoneal injection of PopuCATH significantly drove the leukocyte influx in both frogs and mice. In mice, PopuCATH rapidly drove neutrophil, monocyte/macrophage influx in mouse abdominal cavity and peripheral blood with a negligible impact on T and B lymphocytes, and neutrophils, monocytes/macrophages, but not T and B lymphocytes, were required for the preventive efficacy of PopuCATH. PopuCATH did not directly act as chemoattractant for phagocytes, but PopuCATH obviously drove phagocyte migration when it was cultured with macrophages. PopuCATH significantly elicited chemokine/cytokine production in macrophages through activating p38/ERK mitogen-activated protein kinases (MAPKs) and NF-κB p65. PopuCATH markedly enhanced neutrophil phagocytosis via promoting the release of neutrophil extracellular traps (NETs). Additionally, PopuCATH showed low side effects both in vitro and in vivo. Collectively, PopuCATH acts as a host-based immune defense regulator that provides prophylactic efficacy against bacterial infection without direct antimicrobial effects. Our findings reveal a non-bactericidal cathelicidin which possesses unique anti-bacterial action, and highlight the potential of PopuCATH to prevent bacterial infection.
Editor's evaluation
This manuscript describes for the first time a novel cathelicidin, namely, popuCATH which is able to prevent the development of infection by different bacterial species in two different animal models, frog and mouse. The mechanism of action is exerted through priming of neutrophil efflux.
https://doi.org/10.7554/eLife.72849.sa0Introduction
Antimicrobial peptides (AMPs) are a wide array of gene-encoded small defensive molecules that have been identified from prokaryotic to eukaryotic kingdoms, including bacteria, fungi, plantae, and animalia (Mygind et al., 2005; Radek and Gallo, 2007; Silva et al., 2014; Zhang and Gallo, 2016). In vertebrates, cathelicidins constitute one of the major antimicrobial peptide families (Wei et al., 2013). Cathelicidins are composed of an N-terminal signal peptide (about 30 amino acids), a highly conserved cathelin domain (99–114 residues) between signal peptide and mature peptide, and a C-terminal mature peptide (12–100 residues) with diverse structures (sequence and length) and functions (Zanetti et al., 2000). Based on the structural characterisation, the mature peptides of cathelicidins can be distinguished into amphipathic α-helical structure (e.g. human LL37), beta-sheet structure (e.g. porcine protegrin), and structure enriched in specific amino acids like proline/arginine residues (e.g. bovine Bac5 and Bac7; Zanetti et al., 2000). Cathelicidins were initially characterised for their direct antimicrobial activity (Gennaro et al., 1989), which act as natural amino acid-based antibiotics with broad spectrum that directly target bacteria (Snoussi et al., 2018). Due to their rapid and potent bactericidal property without significant toxicity, cathelicidins have been considered as promising peptide antibiotics for therapy of bacterial infection (Oyston et al., 2009; Zanetti, 2004). Several cathelicidin-derived peptide antibiotics have been tested in clinical trials (Gordon et al., 2005; Mwangi et al., 2019). In addition to direct antimicrobial activity, more and more studies demonstrated that cathelicidins possess diverse immunomodulatory activities (Sun et al., 2015a; Zhang et al., 2015).
Since the first purification of cathelicidins (Bac5 and Bac7) from bovine neutrophils (Gennaro et al., 1989), more than 1500 vertebrate cathelicidins have been identified from aquatic vertebrates to terrestrial vertebrates, including fishes, reptiles, amphibians, birds, and mammals (https://www.ncbi.nlm.nih.gov/protein/?term=cathelicidin). Amphibians, the evolutionary link of vertebrates from aquatic animals to terrestrial animals, possess an ancient but powerful innate immune system to thrive in a wide range of habitats (Xu and Lai, 2015). Gene-encoded AMPs form a first line of innate immunity in amphibians to defense noxious microbes (Li et al., 2007). In the last decades, a total of 8350 (Jun 21, 2021, https://amphibiaweb.org/) amphibian species have been documented, and more than 1900 AMPs have been identified from amphibians (Xu and Lai, 2015). However, cahtelicidins were absent in amphibians until cathelicidin-AL was characterised from Amolops loloensis (Anura: Ranidae), which filled the evolutionary gap of cathelicidin in vertebrates (Hao et al., 2012). So far, about 20 cathelicidins were identified from amphibians, including frog cathelicidins identified from Amolops loloensis (Hao et al., 2012), Paa yunnanensis (Wei et al., 2013), Rana catesbeiana (Ling et al., 2014), Limnonectes fragilis (Anura: Ranidae) (Yu et al., 2013), Microhyla heymonsivogt (Anura: Microhylidae) (Chai et al., 2021), Polypedates puerensis (Anura: Rhacophorinae) (Mu et al., 2017), (Anura: Dicroglossidae), toad cathelicidins identified from Duttaphrynus melanostictus (Gao et al., 2016), Bufo bufo gargarizans (Anura: Bufonidae) (Sun et al., 2015b), salamander cathelicidin identified from Tylototriton verrucosus (Mu et al., 2014), Andrias davidianus (Yang et al., 2017) (Caudata: Salamandridae), and others. Most of these cathelicidins from frogs, toads, and salamanders exhibited direct antimicrobial activities with broad spectrum via dual bactericidal-immunomodulatory activities. For example, cathelicidin-PY and cathelicidin-PP showed bactericidal activity and anti-inflammatory activity by disrupting bacterial membrane and blocking TLR4-mediated inflammatory response, respectively (Mu et al., 2017; Wei et al., 2013).
Overall, the anti-infective action and relative mechanism of bactericidal cathelicidins have been extensively studied. However, the role and mechanism of action of non-bactericidal cathelicidins against bacterial infection remain unknown. In this study, a novel naturally occurring glycine-rich cathelicidin, designated as PopuCATH, was identified from the tree frog of P. puerensis. PopuCATH did not show any direct antimicrobial activities. Interestingly, intraperitoneal injection of PopuCATH effectively prevented bacterial infection in tree frogs and mice, indicating an indirect antimicrobial mechanism of PopuCATH. The mechanism of action was investigated both in vitro and in vivo. Our study provides new insight for better understanding the anti-infective property and relative mechanism of non-bactericidal cathelicidin, and highlights a host-based immune defense regulator for preventing bacterial infection without drug-resistant risk.
Results
A novel naturally occurring cathelicidin was identified from the skin of tree frog, P. puerensis
To understand the peptidomics of P. puerensis skin, the skin secretions were firstly separated by molecular sieving fast pressure liquid chromatography (FPLC) as indicated in Figure 1—figure supplement 1A. The eluted peak containing the objective peptide in this study (marked by an arrow) was further purified by a reversed-phase high-performance liquid chromatography (RP-HPLC) C18 column for two times (Figure 1—figure supplement 1B and C, marked by an arrow). The purified peptide exhibited an observed molecular weight of 4295.9 Da (Figure 1—figure supplement 1D). Then, a total of 16 amino acids at N-terminus were determined as SRGGRGGRGGGGSRGG by automated Edman degradation. The N-terminus is enriched in glycine residues, which is possibly a novel member of cathelicidin antimicrobial peptides like those glycine-rich cathelicidins found in frog (Hao et al., 2012) and fish (Broekman et al., 2011).
According to this implication, we designed primer based on the conserved region of amphibian cathelicidins to clone the gene encoding the objective peptide. The nucleotide sequence (GenBank accession number: KY391886) encoding the precursor of the objective peptide was cloned from the skin cDNA library (Figure 1). The coding sequence of the precursor included 659 nucleotides that encodes a precursor containing 179 amino acid residues (Figure 1). The full-length amino acid sequence of the mature peptide (designated as PopuCATH) was determined as shown in Figure 1. BLAST comparison confirmed that the precursor of PopuCATH is definitely a novel member of cathelicidin antimicrobial peptide family, which shares a highly conserved signal peptide and cathelin domain at N-terminus with amphibian cathelicidins (Figure 1—figure supplement 2A). Phylogenetic tree analysis indicated that PopuCATH combined with amphibian and fish cathelicidins form the second cluster, showing close evolutionary relationship with amphibian cathelicidins and fish cathelicidins (Figure 1—figure supplement 3).
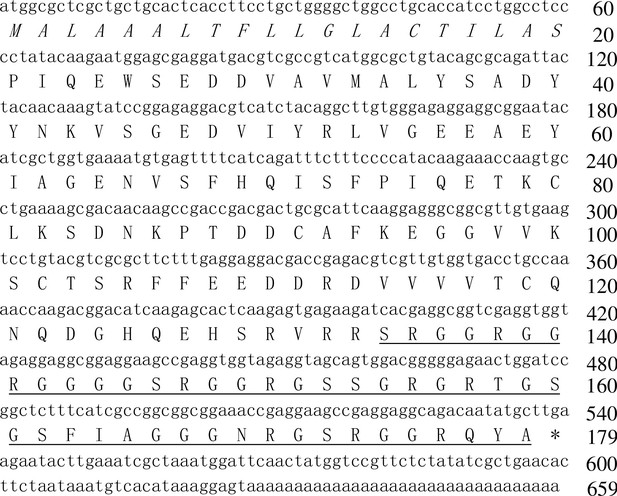
The nucleotide sequence encoding the precursor of PopuCATH and the deduced amino acid sequence.
(A) The amino acid sequence of mature peptide is underlined, and the putative signal peptide is italic. Asterisk (*) indicates stop codon. Deduced amino acid sequence of PopuCATH precursor was translated in ExPASy Translate Tool (http://web.expasy.org/translate/). Sequence Blast was performed with Blastx (https://blast.ncbi.nlm.nih.gov/Blast.cgi).
Primary structural analysis indicated that PopuCATH is composed of 46 amino acid residues, including 41 polar residues and 5 non-polar residues, which is a glycine-rich cathelicidin (21 glycine residues) like those found in frog and fish (Figure 1—figure supplement 2B, Supplementary file 1). PopuCATH has net charges of +10 and a theoretical isoelectric point of 12.60 (Supplementary file 1). The theoretical molecular weight is well matched with the observed molecular weight (Figure 1—figure supplement 1, Supplementary file 1). Secondary structural analysis indicated that PopuCATH mainly adopts random coil confirmation in both aqueous solution and membrane-mimetic solution (Figure 1—figure supplement 4, Supplementary file 2).
PopuCATH lacks direct antimicrobial activity but can prevent bacterial infection in tree frogs
Cathelicidins were initially described for their direct antimicrobial activity (Gennaro et al., 1989). Therefore, we first detected the direct antimicrobial activity of PopuCATH in vitro by MIC assay. To our surprise, PopuCATH didn’t show any antimicrobial activity against the tested bacteria (a total of 40 strains) at the concentration up to 200 µg/mL, including Gram-negative bacteria, Gram-positive bacteria, fungi, and aquatic pathogenic bacteria (Supplementary file 3). Similarly, in time-kill assays, 200 µg/mL of PopuCATH did not reduce the CFUs of E. coli, S. aureus, C. albicans, and A. hydrophila after incubation for 1, 2, 3, and 4 hr, respectively (Figure 2A). Furthermore, 200 µg/mL of PopuCATH did not alter bacterial metabolic activity during the exponential growth phase of E. coli, S. aureus, C. albicans, and A. hydrophila after incubation for 1, 2, 3 and 4 hr, respectively (Figure 2B). Cathelicidins are usually membrane-active agents which can alter the surface morphology of bacteria (Wei et al., 2013). As shown in Figure 2C, 200 µg/mL of PopuCATH did not alter the surface morphology of E. coli and S. aureus after PopuCATH treatment. While the positive control peptide PY (1× MIC, cathelicidin-PY), a previously described amphibian cathelicidin from P. yunnanensis (Wei et al., 2013) markedly inhibited bacterial growth (Supplementary file 3), showed bactericidal activity (Figure 2A), reduced bacterial metabolic activity (Figure 2B), and altered bacterial surface morphology (Figure 2C). These results indicated that PopuCATH lacks direct antimicrobial activity.
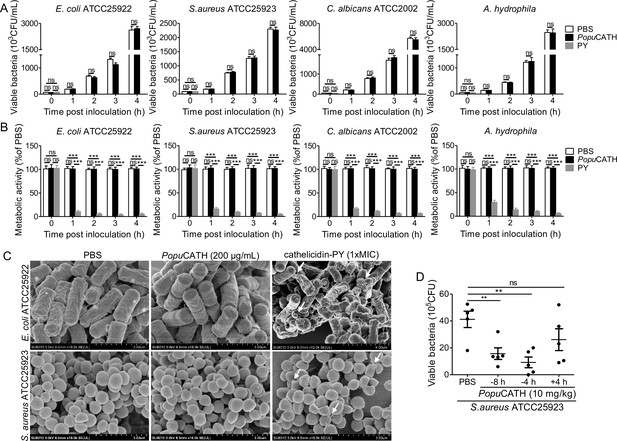
PopuCATH lacks direct antimicrobial activity but can prevent bacterial infection in tree frogs.
(A) Bacterial killing kinetic assay. E. coli, S. aureus, and C. albicans were diluted in Mueller-Hinton broth, and A. hydrophila were diluted in nutrient broth at density of 105 CFU/mL. PopuCATH (200 μg/mL), PY (cathelicidin-PY, 1× MIC, positive control) or PBS was added and incubated at 37℃ or 25℃. At indicated time points, the CFUs were counted. (B) Microbial metabolic activity assay. E. coli, S. aureus, C. albicans, and A. hydrophila were diluted in DMEM at density of 105 CFU/mL, and PopuCATH (200 μg/mL), PY (cathelicidin-PY, 1× MIC, positive control) or PBS was added. Microbial dilution (100 μL/well) and WST-8 (10 μL/well) was added to 96-well plates, respectively, and incubated at 37℃ or 25℃. At indicated time points, absorbance at 255 nm was monitored. Metabolic activity was expressed as the percentage of the PBS-treated group. (C) SEM assay. E. coli ATCC25922 and S. aureus ATCC25923 were washed and diluted in PBS (105 CFU/mL). PopuCATH (200 μg/mL), PY (1× MIC, positive control) or PBS was added into the bacterial dilution and incubated at 37℃. After incubation for 30 min, bacteria were centrifuged at 1000 g for 10 min, and fixed for SEM assay. The bacterial surface morphology was observed using a Hitachi SU8010 SEM. (D) Anti-bacterial activity in tree frogs. PopuCATH (10 mg/kg) was intraperitoneally injected into P. puerensis (n = 5, 21–30 g) at 8 or 4 hr prior to (–8 or –4 hr), or 4 hr after ( + 4 hr) S. aureus ATCC25923 inoculation (108 CFU/frog, intraperitoneal injection). At 18 hr post bacterial challenge, peritoneal lavage was collected for bacterial load assay. **p < 0.01, ***p < 0.001, ns, not significant.
In order to understand whether PopuCATH has antimicrobial activity in vivo, PopuCATH (10 mg/kg) was intraperitoneally injected into P. puerensis 8 hr, or 4 hr prior to (–8 hr or –4 hr), or 4 hr after ( + 4 hr) intraperitoneal bacterial inoculation, and the bacterial load was recorded. Compared to PBS treatment, PopuCATH (10 mg/kg) treatment at 8 hr or 4 hr before bacterial inoculation significantly reduced the bacterial load in tree frogs, but PopuCATH (10 mg/kg) treatment at 4 hr after bacterial inoculation did not significantly reduce the bacterial load (Figure 2D), indicating that pretreatment with PopuCATH significantly prevented bacterial infection in tree frogs.
PopuCATH exhibits low toxic side effects to mammalian cells and mice
In order to further investigate the mechanism of action of PopuCATH against bacterial infection in vivo, it was necessary to move from a frog system to a mouse system. Thus, the toxicity of PopuCATH to mammalian cells and mice were evaluated. At concentration up to 200 μg/mL, PopuCATH didn’t show any cytotoxicity to mouse peritoneal macrophages and humane monocyte THP-1 cells (Figure 3A), and didn’t show any hemolytic activity to mouse erythrocytes and rabbit erythrocytes (Figure 3B).
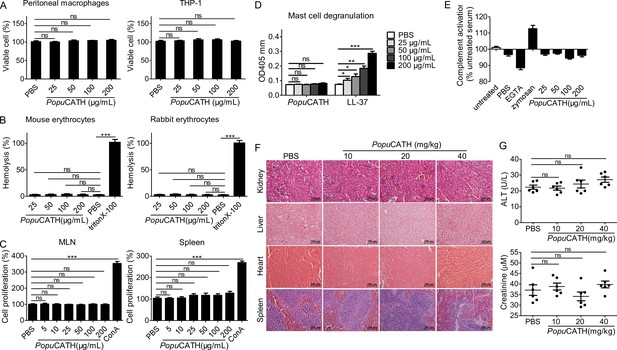
PopuCATH exhibits low toxic side effects to mammalian cells and mice.
(A) Cytotoxicity assay. Peritoneal macrophages or THP-1 cells were seeded in 96-well plates (5 × 105 cells/well, 200 µL). PopuCATH (25, 50, 100, or 200 μg/mL) was added and cultured for 24 hr. CCK-8 reagent (10 µL/well) was added and incubated for 1 hr. The absorbance at 450 nm was recorded. Viable cells was expressed as the percentage of the PBS-treated group. (B) Hemolysis assay. Mouse erythrocytes and rabbit erythrocytes were washed with 0.9% saline and incubated with PopuCATH (25, 50, 100, and 200 μg/mL), PBS or triton X-100 (1%, positive control) at 37℃. After incubation for 30 min, the erythrocytes were centrifuged at 1000 g for 5 min. The absorbance at 540 nm was measured. Hemolytic activity was expressed as the percentage of the triton X-100-treated group. (C) Immunogenicity assay. Lymphocytes isolated from mesenteric lymph node (MLN) and spleen of mice were suspended in RPMI 1640 (2%FBS) and seeded in 96-well plates (5 × 104 cells/well, 200 µL). PopuCATH (25, 50, 100, or 200 μg/mL) or concanavalin A (Con A, 2 μg/mL) was added and incubated at 37℃ for 24 hr. CCK-8 reagent (10 µL/well) was added. After incubation at 37℃ for 1 hr, the absorbance at 450 nm was measured. Cell proliferation was expressed as the percentage of PBS-treated group. (D) Hypersensitivity assay. RBL-2H3 cells were seeded in 96-well plates (2 × 104 cells/well, 200 µL) and cultured overnight. PopuCATH (25, 50, 100, or 200 μg/mL), PBS or LL-37 (25, 50, 100, or 200 μg/mL, positive control) was added and incubated at 37℃ for 0.5 hr. The supernatant was collected for mast cell degranulation assay. (E) Complement activation assay. Mouse serum was treated with PBS, EGTA inhibitor (10 mM), zymosan (0.5 mg/mL), PopuCATH (25, 50, 100, and 200 μg/mL) at 37℃ for 1 hr. C3a des-Arg was measured by ELISA. (F, G) In vivo acute toxicity assay. C57BL/6 mice (18–20 g, n = 6) were intraperitoneally injected with PopuCATH at dose of 10, 20 and 40 mg/kg. At 24 hr post injection, kidneys, livers, hearts and spleens were collected for H&E staining (F), scale bar: 100 μm. Serum was collected for the creatinine and alanine aminotransferase (ALT) measurement (G). *p < 0.05, **p < 0.01, ***p < 0.001, ns, not significant.
The immunogenicity of PopuCATH was evaluated by determination of its proliferative capacity on mouse lymphocytes isolated from mesenteric lymph node (MLN) and spleen. As shown in Figure 3C, PopuCATH did not induce proliferation of lymphocytes isolated from mouse MLN and spleen at any dose tested up to 200 μg/mL, unlike the positive control ConA.
Hypersensitivity to PopuCATH was assessed in a mast cell degranulation assay. Mast cells modulate immediate hypersensitivity reactions and nonspecific inflammatory reactions (Scott et al., 2007). PopuCATH did not induce mast cell degranulation at any dose tested up to 200 mg/mL (Figure 3D), while human cathelicidin LL-37 (positive control) markedly induced mast cell degranulation as described previously (Niyonsaba et al., 2001).
The effect of PopuCATH on complement activation was determined by measurement of C3a after incubation of PopuCATH with mouse serum. PopuCATH didn’t exhibit significant effect on complement activation at concentration up to 200 μg/mL, in contrast to the controls of ethylene glycol tetraacetic acid (EGTA) and zymosan (Figure 3E).
The in vivo acute toxicity of PopuCATH was assessed by histopathological study and blood routine examination at 24 hr after intraperitoneal injection of PopuCATH in C57BL/6 mice. Compared to PBS treatment, PopuCATH treatment did not cause pathological abnormality in kidney, liver, heart, and spleen at any dose tested up to 40 mg/kg (Figure 3F). The levels of alanine aminotransferase (ALT) and creatinine in the sera of mice between PopuCATH- and PBS-treated groups showed no significant difference (Figure 3G), suggesting that high or low doses of PopuCATH does not affect the hepatic and renal function of mice.
The highest amount of administered substance that does not kill tested animals was recorded as the maximum tolerable dose (Scott et al., 2007). The maximum tolerable dose of PopuCATH was tested in C57BL/6 mice by intravenous and intraperitoneal delivery. The maximum tolerable dose of PopuCATH by intravenous delivery was between 75 and 100 mg/kg, and intraperitoneal delivery was between 125 and 150 mg/kg. Markedly, PopuCATH was not toxic at substantially higher concentrations via the intraperitoneal route, well above the doses (10, 20, and 40 mg/kg) used in the mouse models.
PopuCATH provides prophylactic efficacy against bacterial infection in mice
We next examined whether PopuCATH protects against bacterial infection in mice as observed in tree frogs. Mice were intraperitoneally injected with PopuCATH at 8 or 4 hr (–8 or –4 hr) prior to, or 4 hr after ( + 4 hr) intraperitoneal inoculation of bacteria. Compared to PBS injection, PopuCATH injection at –8 or –4 hr significantly reduced the bacterial loads in the abdominal cavity of mice post Gram-negative bacteria (E. coli, A. baumannii) and Gram-positive bacteria (S. aureus, methicillin-resistant S. aureus, MRSA) inoculation (Figure 4A). Bacterial inoculation significantly elicited the production of pro-inflammatory cytokines (TNF-α, IL-1β, and IL-6) in mouse serum relative to control mice (sham), while PopuCATH injection at –8 or –4 hr significantly reduced the production of pro-inflammatory cytokines in mouse serum (Figure 4B). Consistent with these findings, E. coli or S. aureus inoculation markedly induced inflammatory damage in the lung, and PopuCATH injection at –4 or –8 hr obviously rescued this inflammatory damage induced by bacterial inoculation (Figure 4C), suggesting its prophylactic efficacy against bacterial infection, and PopuCATH (10 mg/kg) also showed prophylactic efficacy against bacterial infection via intravenous injection (Figure 4—figure supplement 1). However, at the dose of 10 mg/kg, PopuCATH treatment at +4 hr did not significantly reduce the bacterial loads in abdominal cavity, the production of pro-inflammatory cytokines in serum, and the inflammatory damage in lung as compared to control mice (sham, Figure 4A–C).
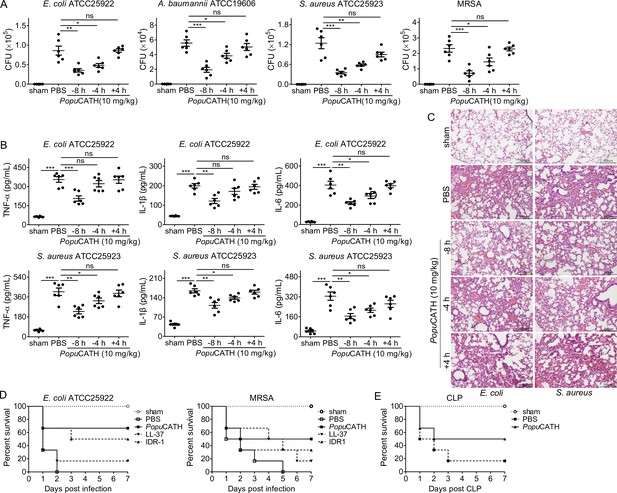
PopuCATH provides prophylactic efficacy against bacterial infection in mice.
(A–C) PopuCATH (10 mg/kg) was intraperitoneally injected into C57BL/6 mice (18–20 g, n = 6) 8 or 4 hr prior to (–8 or –4 hr), or 4 hr after ( + 4 hr) E. coli, A. baumannii, S. aureus or methicillin-resistant S. aureus (MRSA) inoculation (2 × 107 CFUs/mouse, intraperitoneal injection). At 18 hr post inoculation, peritoneal lavage was collected for bacterial load assay (A), serum was collected for cytokine assay (B), and lungs were taken for histopathological assay (C), scale bar: 200 μm. (D) C57BL/6 mice (18–20 g, n = 6) were intraperitoneally injected with PopuCATH (10 mg/kg), LL-37, or IDR-1 (control peptides) 4 hr prior to a lethal dose of E. coli (4 × 107 CFUs/mouse) or MRSA (6 × 108 CFUs/mouse, intraperitoneal injection) inoculation. The survival rates of mice were monitored for 7 days. (E) C57BL/6 mice (18–20 g, n = 6) were intraperitoneally injected with PopuCATH (10 mg/kg) at 8 and 4 hr (two times) prior to (–8 and –4 hr) CLP. At 0 hr, mice were anaesthesied with ketamine (100 mg/kg), and CLP was performed. The survival rates of mice were monitored for 7 days. *p < 0.05, **p < 0.01, ***p < 0.001, ns, not significant.
In order to further evaluate the prophylactic efficacy of PopuCATH against bacterial infection, mice were intraperitoneally injected with PopuCATH before a lethal dose of E. coli or MRSA inoculation, and the survival rates of mice were monitored for up to 7 days. Compared to PBS treatment, PopuCATH pretreatment markedly increased the survival rates of mice challenged by a lethal dose of E. coli or MRSA (Figure 4D). Besides, PopuCATH exhibited a better prophylactic efficacy than those of LL-37 (human cathelicidin) and IDR-1 (bovine cathelicidin derivative) against a lethal dose of bacterial infection (Figure 4D). We then evaluated the protective efficacy of PopuCATH in a CLP-induced sepsis model. We found that PopuCATH pretreatment markedly increased the survival rate of mice against CLP-induced sepsis (Figure 4E). These data suggested that PopuCATH (10 mg/kg) pretreatment effectively provided prophylactic efficacy against bacterial infection and prevented sepsis induced by a lethal dose of bacterial inoculation or CLP in mice.
Intraperitoneal injection of PopuCATH induces leukocyte influx in both mice and tree frogs
Successful clearance of bacterial infection depended on an efficient phagocyte migration into the infectious sites (Alves-Filho et al., 2010; Nathan, 2006; Scott et al., 2007). We thereby investigated whether PopuCATH elicits phagocyte recruitment in mice. As shown in Figure 5, an intraperitoneal injection of PopuCATH (10 mg/kg) significantly induced the recruitment of leukocytes in the abdominal cavity (Figure 5A&B) and peripheral blood (Figure 5C&D) of mice, and PopuCATH was mainly chemotactic to myeloid cells with a negligible impact on lymphoid cells (Figure 5, Figure 5—figure supplement 1). In detail, the main myeloid cells recruited by PopuCATH were neutrophils, Ly6Chigh macrophages and Ly6Chigh monocytes in mouse abdominal cavity (Figure 5A&B), and were neutrophils, Ly6Chigh monocytes and Ly6Clow monocytes in mouse peripheral blood (Figure 5C&D), indicating that intraperitoneal injection of PopuCATH significantly induced phagocyte influx in the abdominal cavity and peripheral blood of mice. Chemotactic kinetic assay indicated that the chemotactic effect induced by PopuCATH can last for 24 hr in abdominal cavity (Figure 5—figure supplement 2), and last for 48 hr in peripheral blood (Figure 5—figure supplement 3). Whereas an intraperitoneal injection of LPS (20 µg/mouse) elicited a different pattern of cellular influx in mice (Figure 5—figure supplement 4), suggesting that chemotaxis observed in mouse peritoneal cavity and peripheral blood were specifically due to PopuCATH rather than possible endotoxin contamination. In addition, we assayed if PopuCATH induce leukocyte influx in tree frogs. As shown in Figure 5—figure supplement 5, an intraperitoneal injection of PopuCATH obviously recruited leukocytes to the abdominal cavity of tree frogs, which is consistent with the data observed in mice.
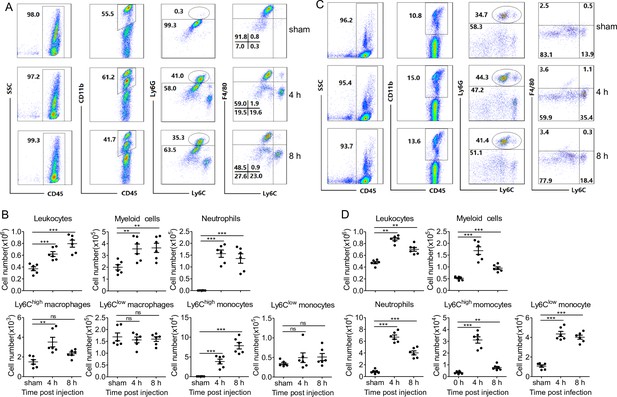
Intraperitoneal injection of PopuCATH induces leukocyte influx in mice.
(A, C) Representative flow cytometry plots and proportions of major population of myeloid cells in peritoneal lavage (A) and peripheral blood (C) are shown. (B, D) Quantitative summary of leukocytes, myeloid cells, neutrophils, monocytes/macrophages in peritoneal lavage (B) and peripheral blood (D). C57BL/6 mice (18–20 g, n = 6) were intraperitoneally injected with PopuCATH (10 mg/kg) dissolved in 0.2 mL PBS. Sham mice received the same volumes of PBS. At 4 and 8 hr post injection, cells in peritoneal lavage and peripheral blood were collected for flow cytometry assay. **p < 0.01, ***p < 0.001, ns, not significant.
Neutrophils and monocytes/macrophages, but not T and B cells, are required for the protective efficacy of PopuCATH in mice
PopuCATH was chemotactic to neutrophils and monocytes/macrophages in both mouse abdominal cavity and peripheral blood. To examine whether the protective capacity of PopuCATH depends on these phagocytic cell types, we evaluated the prophylactic efficacy of PopuCATH in mice after the neutrophils or monocytes/macrophages were depleted by anti-Ly6G or anti-CSF1R antibody (Figure 6—figure supplement 1). As shown in Figure 6, PopuCATH failed to provide prophylactic efficacy against E. coli (Figure 6A) and S. aureus (Figure 6B) infection in neutrophil-depleted mice, and PopuCATH was not efficacious against E. coli (Figure 6C) and S. aureus (Figure 6D) infection in monocyte/macrophage-depleted mice. As mentioned above, PopuCATH was primarily chemotactic to myeloid cells with a negligible impact on lymphoid cells. To confirm this finding, we next tested its prophylactic efficacy in Rag1–/– mice, which are T and B lymphocyte-deficient mice. In contrast to neutrophil and monocyte/macrophage depletion, PopuCATH still provided prophylactic efficacy against E. coli (Figure 6E) and S. aureus (Figure 6F) infection in Rag1–/– mice. These data suggested that myeloid cells, but not lymphoid cells, are required for PopuCATH-mediated protection against bacterial infection in mice.
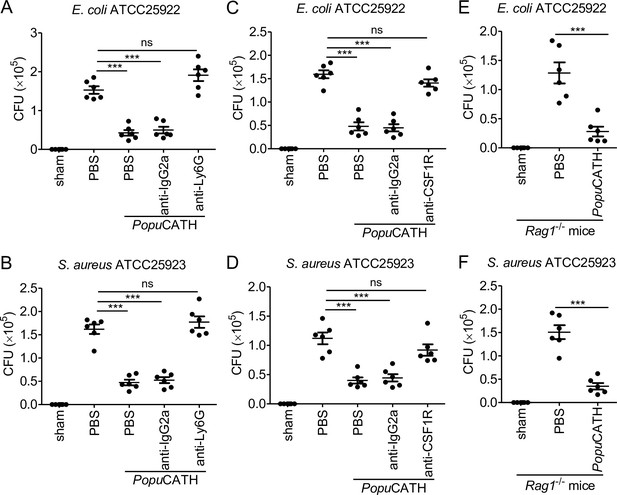
Neutrophils and monocytes/macrophages, but not T and B cells, are required for the prophylactic efficacy of PopuCATH in mice.
(A, B) Protective efficacy of PopuCATH against E. coli (A) or S. aureus (B) in neutrophil depletion mice. Anti-Ly6G antibody or rat IgG2a isotype antibody were intraperitoneally injected into C57BL/6 mice (18–20 g, n = 6) at doses of 500 µg/mouse on day 0 and day 2, respectively. (C, D) Protective efficacy of PopuCATH against E. coli (C) or S. aureus (D) in monocyte/macrophage depletion mice. Anti-CSF1R antibody or rat IgG2a isotype antibody were intraperitoneally injected into C57BL/6 mice (18–20 g, n = 6) at doses of 1 mg/mouse on day 0 followed by 0.3 mg/mouse on day 1 and day 2, respectively. (E, F) Protective efficacy of PopuCATH against E. coli (E) or S. aureus (F) in Rag1–/– mice (18–20 g, n = 6). At 4 hr before E. coli or S. aureus (2 × 107 CFUs/mouse) inoculation, PopuCATH (10 mg/kg) was intraperitoneally injected into neutrophil depletion mice (on day 3), monocyte/macrophage depletion mice (on day 3), and Rag1–/– mice. At 18 hr post bacterial inoculation, peritoneal lavage was collected for the bacterial load assay. ***p < 0.001, ns, not significant.
PopuCATH-induced phagocyte migration relies on its effect on macrophages
Given the increase in neutrophils and monocytes/macrophages in the abdominal cavity and peripheral blood, we were interested to investigate if PopuCATH acts as a chemoattractant for neutrophils and macrophages. As shown in Figure 7, PopuCATH (10 μM) did not directly induce neutrophil migration (Figure 7A) and macrophage migration (Figure 7B), suggesting that PopuCATH cannot act as a chemoattractant for neutrophils and macrophages. Macrophages have been shown to produce chemokines/cytokines that recruit other cells, and macrophages are the major immune cells in mouse abdominal cavity (Scott et al., 2007; Yang et al., 2021). We next investigated whether PopuCATH induce phagocyte migration in the presence of macrophages. As shown in Figure 7, PopuCATH (10 μM) markedly induced neutrophil migration (Figure 7A) and macrophage migration (Figure 7B) in the presence of peritoneal macrophages. The addition of PopuCATH (10 μM) in the lower chamber elicited about 2.3 × 105 neutrophil migration (Figure 7A) and 2.0 × 103 macrophage migration (Figure 7B) when peritoneal macrophages were cultured in the lower chamber, implying that PopuCATH-induced phagocyte migration might rely on PopuCATH-triggered immune response in macrophages.
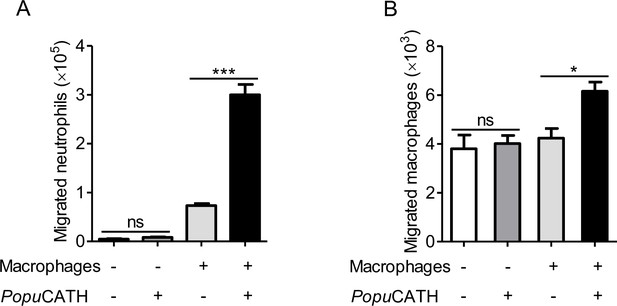
PopuCATH-induced phagocyte migration relies on its effect on macrophages.
For the direct chemotactic effect of PopuCATH to neutrophils or macrophages, 100 µL of neutrophil suspension (A) or macrophage suspension (B) (5 × 106 cells/mL) was added to the upper chamber, and 500 µL of PopuCATH (10 µM, dissolved in medium) or medium was added to the lower chamber. After neutrophils and macrophages were migrated at 37 ℃ for 8 hr, the increased cells in the lower chamber were collected and counted using a hemocytometer. For the co-cultured system, 500 µL of macrophage suspension (5 × 106 cells/mL) was seeded in the lower chamber. After macrophages were adherent to the lower chamber, 100 µL of neutrophil suspension (A) or macrophage suspension (B) (5 × 106 cells/mL) was added to the upper chamber. Then, the medium in the lower chamber was replaced with 500 µL of PopuCATH (10 µM, dissolved in medium) or fresh medium. Neutrophils and macrophages were migrated at 37 ℃ for 8 hr. The reduced cells in the upper chamber were counted using a hemocytometer. *p< 0.05, ***p < 0.001, ns, not significant.
PopuCATH selectively induced the production of chemokines/cytokines in macrophages and mice
To confirm whether PopuCATH-induced phagocyte migration relies on PopuCATH-triggered immune response in macrophages, we stimulated mouse peritoneal macrophages with a single dose of PopuCATH (10 μM) for 4 hr and analysed the mRNA levels of chemokines/cytokines. As shown in Figure 8A, the mRNA levels of Cxcl1, Cxcl2, Cxcl3, Il1b, and Il6 were significantly increased by 60.9-, 74.2-, 17.0-, 15.5-, and 18.6-fold in peritoneal macrophages post PopuCATH treatment relative to PBS treatment (p < 0.05). In contrast, the mRNA levels of the other chemokines and cytokines, including Ccl4, Ccl5, Ccl6, Ccl8, Ccl9, Ccl10, Ccl14, Csf1, Il4, Il12, and Tnfa were slightly upregulated in peritoneal macrophages post PopuCATH treatment relative to PBS treatment, ranging from 1.5- to 3-fold (p > 0.05). We next stimulated mouse peritoneal macrophages with different dose of PopuCATH (5, 10, and 20 μM) to verify the results observed in Figure 8A. As shown in Figure 8B, mRNA levels of chemokines (Cxcl1, Cxcl2, and Cxcl3) and cytokines (Il1b and Il6) were significantly upregulated in a dose-dependent manner (p < 0.05). The others didn’t generate a dose-dependent effect. To confirm the results observed by mRNA quantification, we detected the protein levels of the upregulated chemokines/cytokines by ELISA. PopuCATH significantly induced the protein production of CXCL1, CXCL2, and CXCL3 in a dose-dependent manner, whereas PopuCATH did not significantly induce the protein production of CXCL1, TNF-α, IL-1β, and IL-6 although their mRNA levels were upregulated (Figure 8C). In vivo assay showed that an intraperitoneal injection of PopuCATH (10 mg/kg) significantly induced the production of the chemokines (CXCL1, CXCL2, and CXCL3) as well as the pro-inflammatory cytokines (IL-1β and IL-6) in mouse abdominal cavity (Figure 8D). The results indicated that PopuCATH directly acted on macrophages and selectively induced the production of chemoattractant which are critical for the recruitment of phagocytes.
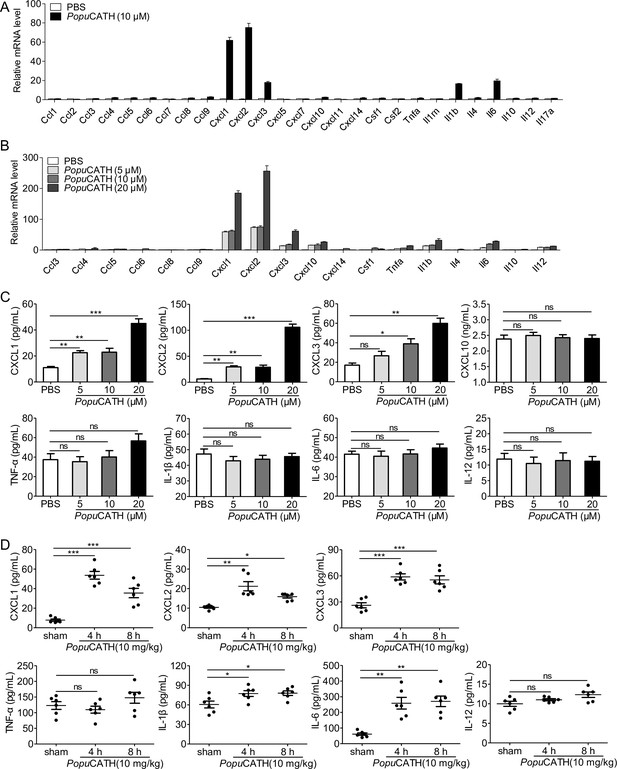
PopuCATH selectively induced the production of chemokines in macrophages and mice.
(A) The mRNA levels of chemokines/cytokines in macrophages induced by PopuCATH (10 µM). (B) Verification of the upregulated chemokines/cytokines observed in panel A by qPCR. (C) The protein levels of chemokine/cytokine production in macrophages induced by PopuCATH (10 µM). Macrophages (5 × 105 cells/well, in 2% FBS DMEM) were seeded in 24-well plates, and a single dose of PopuCATH (10 µM, dissolved in PBS) (A) or different doses of PopuCATH (5, 10, and 20 µM, dissolved in PBS) (B), or PBS was added. After incubation at 37℃ for 4 hr, cells were collected, and the mRNA levels of chemokines/cytokines were detected by qPCR analysis, the protein levels of chemokines/cytokines were quantified by ELISA (C). (D) The protein levels of chemokine/cytokines in mice induced by PopuCATH (10 mg/kg). C57BL/6 mice (18–20 g, n = 6) were intraperitoneally injected with PopuCATH (10 mg/kg) dissolved in 0.2 mL PBS. Sham mice received the same volumes of PBS. At 4 and 8 hr post injection, peritoneal lavage was collected for quantification of the protein levels of chemokines/cytokines by ELISA. *p < 0.05, **p < 0.01, ***p < 0.001, ns, not significant.
PopuCATH-induced chemokine production in macrophages were partially dependent on p38/ERK MAPKs and NF-κB signaling pathways
To investigate the signaling pathways by which chemokines were induced by PopuCATH in macrophages, mouse peritoneal macrophages were pretreated with various inhibitors, including p38, ERK1/2, JNK1/2, PI3K and NF-κB, and responses induced by PopuCATH were analysed. As shown in Figure 9A, chemokines (CXCL1, CXCL2, and CXCL3) induced by PopuCATH were markedly attenuated after p38/ERK MAPKs, or NF-κB blockade, whereas inhibitors of JNK MAPK and PI3K pathway had no significant effect on PopuCATH-induced chemokine production in macrophages. Consistent with these results, PopuCATH (10 μM) significantly activated p38/ERK MAPKs and NF-κB p65 (Figure 9B&C). But inhibition of p38/ERK MAPKs or NF-κB signaling pathways did not completely blocked PopuCATH-mediated chemokine production in macrophages, we cannot not exclude other possible signaling pathways were involved. The data suggested that PopuCATH-mediated chemokine production in macrophages partially depended on p38/ERK MAPKs and NF-κB signaling pathways.
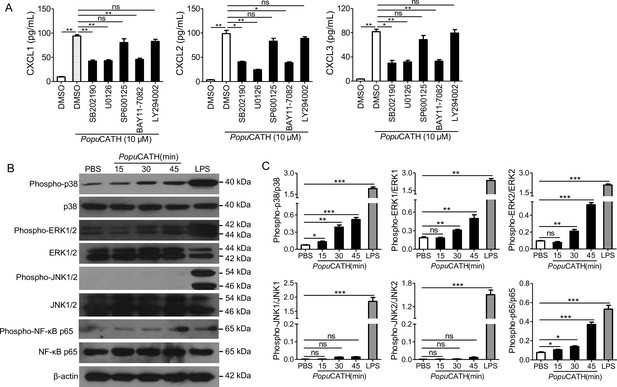
PopuCATH-induced chemokine production in macrophages were partially dependent on p38/ERK MAPKs and NF-κB signaling pathways.
(A) Effects of MAPK, PI3K, and NF-κB inhibitors on PopuCATH-induced chemokine production in macrophages. Macrophages (5 × 105 cells/well, in 2% FBS DMEM) were seeded in 24-well plates. The adherent macrophages were pre-incubated with p38 inhibitor (SB202190, 10 µM), ERK inhibitor (U0126, 10 µM), JNK inhibitor (SP600125, 10 µM), NF-κB inhibitor (BAY11-7082, 2 µM), or PI3K inhibitor (LY294002, 10 µM) for 1 hr, respectively. Then, cells were stimulated with PopuCATH (10 µM) for 4 hr. The protein levels of chemokines were quantified by ELISA. (B) Western blot analysis of the effects of PopuCATH on MAPKs and NF-κB. Macrophages (2 × 106 cells/well, in 2% FBS DMEM) were seeded in 6-well plates. PopuCATH (10 µM) was added and incubated at 37℃ for 15, 30, and 45 min, respectively. LPS (100 ng/mL, positive control) or PBS (solvent of peptide) was added and incubated for 30 min. The cells were collected for western blot analysis. (C) Ratio analysis. The ratios of phosphorylated-p38, JNK, ERK, and NF-κB p65 to total p38, JNK, ERK, and NF-κB p65 were assayed by image J, respectively. *p < 0.05, **p < 0.01, ***p < 0.001, ns, not significant.
-
Figure 9—source data 1
The original images of the unedited blots and images with the uncropped blots with the relevant bands clearly labelled.
- https://cdn.elifesciences.org/articles/72849/elife-72849-fig9-data1-v1.zip
PopuCATH promoted neutrophil phagocytosis through eliciting neutrophil extracellular traps
It is noted that PopuCATH primarily drove neutrophil influx in both peritoneal cavity and peripheral blood, and peaked at 4 hr post intraperitoneal injection of PopuCATH with an increment of approximately 1.54 × 105 neutrophils in mouse abdominal cavity (Figure 5A&B) and 5.64 × 106 neutrophils in mouse peripheral blood (Figure 5C&D) relative to control mice (sham), indicating that neutrophils exhibited a rapid response to PopuCATH. We herein tried to understand whether PopuCATH directly act on neutrophils to promote bacterial clearance. As illustrated in Figure 10A, PopuCATH significantly promoted phagocytic uptake of bacterial particles by mouse neutrophils. To investigate the mechanism by which PopuCATH promoted the phagocytic activity of neutrophils, the capacity of PopuCATH to induce neutrophil extracellular traps (NETs) were detected as indicated in Figure 10B. Single treatment of PopuCATH or PMA (positive control) markedly induced the formation of NETs as compared to PBS treatment, and co-treatment of PopuCATH and PMA increased the formation of NETs relative to single PopuCATH or PMA treatment, indicating that PopuCATH-enhanced neutrophil phagocytosis is attributed to PopuCATH-induced NETs formation.
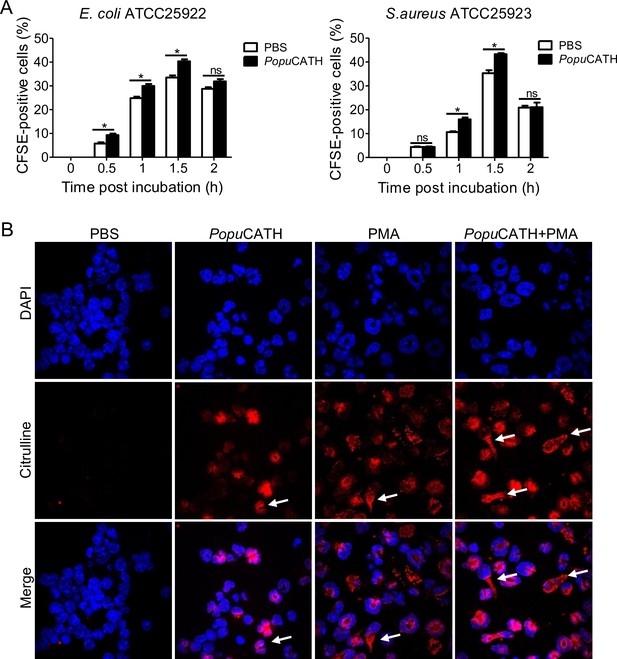
PopuCATH promoted neutrophil phagocytosis through enhancing neutrophil extracellular traps formation.
(A) Enhancement of neutrophil phagocytosis of S. aureus and E. coli by PopuCATH. Neutrophils were pre-incubated with PopuCATH (10 µM) or PBS (solvent of peptide) for 1 hr, and CFSE-labelled bacterial particles were added and incubated for indicated time points. The CFSE fluorescence were analysed by flow cytometry as a measure of the phagocytic uptake of the bacterial particles. (B) Enhancement of neutrophil extracellular formation. Neutrophils were incubated with PBS, PopuCATH (10 µM), PMA (100 nM) or PopuCATH (10 µM)+ PMA (100 nM) at 37℃ for 4 hr, respectively. Nuclei and NETs were stained with DAPI (blue) or anti-Cit-H3 (red), respectively. NETs were observed using a confocal microscope ( × 60). *p < 0.05, ns, not significant.
Discussion
We herein identified a novel amphibian cathelicidin designated as PopuCATH. In vitro antimicrobial assay, PopuCATH was devoid of any antimicrobial activity, including no significant effects on bacterial growth, metabolic activity and surface morphology, which is different from the bactericidal amphibian cathelicidins in previous studies. Intriguingly, although PopuCATH lacks direct anti-bacterial activities in vitro, it effectively provides prophylactic efficacy against bacterial infection in vivo with a broad spectrum, including Gran-negative bacteria, Gram-positive bacteria, and even clinically isolated methicillin-resistant S. aureus. Intraperitoneal injection of PopuCATH before bacterial inoculation significantly attenuated the bacterial load in tree frogs and mice, reduced inflammatory responses induced by bacterial inoculation in mice, and increased the survival rates of septic mice induced by lethal dose of bacterial inoculation and CLP. Except for the intraperitoneal injection, intravenous injection of PopuCATH also effectively provides prophylactic efficacy against bacterial infection (Figure 4—figure supplement 1). The results indicates that PopuCATH can provide preventive capacity via both intraperitoneal and intravenous injection routes. While intramuscular injection had no significant preventive effects against bacterial infection (Figure 4—figure supplement 1). It is more likely that muscle is not rich in neutrophils, monocytes/macrophages, which are the key effector cells of PopuCATH. At the dose of 10 mg/kg, PopuCATH did not exhibit therapeutic efficacy against bacterial infection. In order to evaluate whether it has therapeutic efficacy at high doses, we increased the dose of PopuCATH. At doses of 20 and 40 mg/kg, PopuCATH significantly reduced the bacterial load when it was given at 4 hr after E. coli inoculation (Figure 4—figure supplement 2). It is possibly that bacteria have colonised in mice at 4 hr after bacterial inoculation, which need a higher dose of PopuCATH to drive more phagocytes for bacterial clearance. To the best of our knowledge, this is the first report of a non-bactericidal cathelicidin that can protect bacterial infection in vivo.
The extent of microbial infection-mediated host damage largely depends on host’s immune status. If the host can effectively initiate an immune defense, the invading microbes will be cleared, and host damage induced by microbial infection will be prevented or controlled. On the contrary, if the host cannot effectively initiate an immune defense, host will lose a balanced protection and microbial infection-mediated host damage will follow (Silva, 2010). Neutrophils and macrophages are two professional phagocytic cell types, which comprise a myeloid phagocyte system of host. Neutrophils and macrophages usually work together in innate immunity as complementary partners of the myeloid phagocytic system. The local and global distribution patterns of neutrophils and macrophages are key immune parameter of host, which play critical roles in initiating effective immune defense against invading microbes. Our findings revealed that intraperitoneal injection of PopuCATH effectively elicited neutrophil and monocyte/macrophage influx in mice, and depletion of neutrophils or monocytes/macrophages blocked PopuCATH-mediated protection, indicating that PopuCATH-mediated protection depends on PopuCATH-induced neutrophil and monocyte/macrophage influx. Previous investigations have demonstrated that successful clearance of invading microbes largely depends on efficient migration of these cell types into the infectious sites (Alves-Filho et al., 2010; Li et al., 2013; Nathan, 2006; Scott et al., 2007). These suggest that intraperitoneal injection of PopuCATH enhanced the myeloid phagocytic system of mice, thus providing prophylactic efficacy against bacterial infection.
Macrophages have been shown to phagocytose and directly kill bacteria (Nijnik et al., 2010; Scott et al., 2007). In our study, we found that PopuCATH did not promote in vitro phagocytosis of fluorescently labelled bacterial particles by mouse peritoneal macrophages (Figure 8—figure supplement 2), suggesting no direct stimulation of the phagocytic activity of macrophages by PopuCATH. But PopuCATH significantly exhibited immunomodulatory effects on macrophages to induce phagocyte influx. In addition, neutrophils are principal phagocytes in the innate defense system and kill pathogens through mechanisms like oxidative killing activity and release of neutrophil extracellular traps (Neumann et al., 2014; Niyonsaba et al., 2013; Rowe-Magnus et al., 2019), and an influx of neutrophils to the site of infection is pivotal for the clearance of infectious bacteria (Alves-Filho et al., 2010). In our study, PopuCATH was merely demonstrated to promote neutrophil phagocytosis through inducing NET formation, but not significantly elicited oxidative killing activity of neutrophils (Figure 10—figure supplement 2). These results indicated that macrophages and neutrophils responded to PopuCATH in their own manner.
The expression profiles of chemokines/cytokines in vitro and in vivo are somewhat different, but we observed that the profiles of the major chemokines/cytokines induced by PopuCATH, such as CXCL1, CXCL2, and CXCL3, are similar. We presumed that PopuCATH-induced chemokines/cytokines in vivo are consumed timely. In addition, macrophage is the unique effector cell type of PopuCATH in vitro. While there are many other cell types in vivo, such as monocytes and neutrophils, and we cannot exclude these cells are responsive to PopuCATH and subsequently produce chemokines/cytokines. These may explain the subtle differences of PopuCATH-mediated chemokine/cytokine production in macrophages and mice. In mouse model, the production of chemokines/cytokines in mouse abdominal cavity peaked at 4 hr post injection of PopuCATH. The dynamic of CXCL1, CXCL2, and CXCL3 production is consistent with the dynamic of neutrophil, monocyte/macrophage recruitment in the mouse abdominal cavity and peripheral blood. Although the pretreatment with PopuCATH significantly induced the production of chemokines (CXCL1, CXCL2, and CXCL3) as well as pro-inflammatory cytokines (IL-1β and IL-6), PopuCATH ultimately attenuated the inflammatory response by decrease of TNF-α, IL-1β, and IL-6 levels post Gram-negative and Gram-positive bacterial infection. Cathelicidins are able to block Toll-like receptor (TLR)-mediated inflammatory responses, including those mediated by TLR2 and TLR4 (Coorens et al., 2017; Mookherjee et al., 2006; Wei et al., 2013). In this study, PopuCATH did not affect LTA- and LPS-stimulated inflammatory responses in mouse peritoneal macrophages (Figure 8—figure supplement 3), suggesting that the anti-inflammatory effects of PopuCATH are independent of TLRs, and the attenuation of the inflammation may be secondary to the decrease of bacterial growth.
Some of the properties of PopuCATH are reminiscent of the activities of other cathelicidins like LL-37 (Chen et al., 2000), CRAMP (Kurosaka et al., 2005), and OH-CATH30 (Li et al., 2013), which selectively modulated innate immune responses and have been proposed to mediate protection in animal models. Compared to theses bactericidal cathelicidins with immunomodulatory properties, (i) the usage of PopuCATH is unlikely to induce drug-resistance because the peptide is unable to directly elicit stress on microbes. (ii) PopuCATH showed low side effects unlike LL-37 (Bąbolewska and Brzezińska-Błaszczyk, 2015). (iii) The expression profile of chemokines/cytokines in response to PopuCATH were largely different from those of other cathelicidins. (iv) Key effector cells for PopuCATH were also largely different from those of LL-37, CRAMP and OH-CATH30. For example, human cathelicidin peptide LL-37 has been shown to directly recruit neutrophils, monocytes, mast cells, and T lymphocytes (Chen et al., 2000; Niyonsaba et al., 2002). While PopuCATH did not directly recruited leukocytes, it just recruited neutrophils and monocytes/macrophages via inducing chemokine/cytokine production in macrophages. In addition, PopuCATH just elicited neutrophil and monocyte/macrophage recruitment, but not T and B lymphocytes. Intraperitoneal injection of PopuCATH significantly drove phagocyte influx in both abdominal cavity and peripheral blood, demonstrating that it effectively regulated both local and global innate immune response. As a result, PopuCATH pretreatment effectively reduced the bacterial load in both abdominal cavity and peripheral blood (Figure 4—figure supplement 3). (v) Intriguingly, PopuCATH is a glycine-rich cathelicidin containing 21 glycine residues. The amino acid component is different from LL-37, CRAMP, and OH-CATH30, which are not special residue-rich cathelicidins. The substitution of glycine residues of PopuCATH with alanine residues significantly resulted in a reduced efficacy against bacterial infection (Figure 4—figure supplement 4). In addition, PopuCATH contains 10 arginine residues and seven serine residues (Supplementary file 1). The substitution of arginine residues or serine residues with alanine residues also significantly led to a decreased efficacy against bacterial infection (Figure 4—figure supplement 4). These data demonstrated that these enriched amino acid residues, including 21 glycine residues, 10 arginine residues, and 7 serine residues, are key structural requirements for PopuCATH-mediated protective efficacy against bacterial infection, and PopuCATH-mediated protection were specifically due to its unique structure. The first frog-derived cathelicidin is also rich in glycine residues. But it has different amino acid sequence with PopuCATH and exhibits direct antibacterial activity unlike PopuCATH (Hao et al., 2012). Cathelicidin antimicrobial peptides display a high structural diversity, and the diverse structures are responsible for their diverse functions. Accordingly, it is not difficult to understand that these two frog cathelicidins have different functions against bacteria.
Recently, many progresses have been achieved in the development of anti-resistance therapy for combatting multidrug resistant bacterial infection. Pre-clinical and clinical data pointed out host-directed therapeutic approaches to enhance ‘pauci-inflammatory’ microbial killing in myeloid phagocytes merited particular attention (Watson et al., 2020). Host-based therapeutic strategies can maximise microbial clearance and minimise host’s harmful consequences induced by inflammatory response, which has great promise. PopuCATH did not show any direct effects on bacteria, but effectively prevented bacterial infection through eliciting phagocyte influx and slightly promoting neutrophil phagocytosis. PopuCATH-mediated protection against bacterial infection can be considered as a classic host-based therapeutic strategy, and the non-bactericidal nature of PopuCATH may reduce the selective pressures that drive bacterial resistance. In an era of emerging and re-emerging infectious diseases, discovery and development of naturally occurring non-bactericidal antimicrobial peptides like PopuCATH may facilitate us to prevent and overcome multidrug-resistant bacterial infection.
In summary, a glycine-rich amphibian cathelicidin, PopuCATH, was identified from tree frog. PopuCATH didn’t show any direct effects on bacteria but provided protection against bacterial infection in vivo. PopuCATH acted as an immune defense regulator against bacterial infection by selective modulation of innate immune response. Our findings provide new insights into the development of non-bactericidal cathelicidins to prevent bacterial infection.
Materials and methods
Reagent type (species) or resource | Designation | Source or reference | Identifiers | Additional information |
---|---|---|---|---|
Cell line (Homo sapiens) | THP-1 | National Collection of Authenticated Cell Cultures (https://www.cellbank.org.cn/) | CSTR:19375.09.3101HUMSCSP567 | |
Cell line (Rattus norvegicus) | RBL-2H3 | National Collection of Authenticated Cell Cultures (https://www.cellbank.org.cn/) | CSTR:19375.09.3101RATTCR7 | |
Cell line (Mus musculus) | Macrophage | Peritoneal macrophages from C57BL/6 mice | A primary cell line identified by flow cytometry | |
Cell line (Mus musculus) | Neutrophil | Bone marrow-derived neutrophils from C57BL/6 mice | A primary cell line identified by flow cytometry | |
Commercial assay or kit | SMART cDNA Library Construction Kit | Clontech | Cat#: 634,901 | |
Commercial assay or kit | Cell Counting Kit-8 | Dojindo | Cat#: CK04-500T | |
Commercial assay or kit | Mouse C3a ELISA Kit | Wuhan Fine Biotech Co., Ltd | Cat#: EM0882 | |
Commercial assay or kit | Alanine aminotransferase Assay Kit | Nanjing Jiancheng Bioengineering Institute | Cat#: C009-2-1 | |
Commercial assay or kit | Creatinine Assay Kit | Nanjing Jiancheng Bioengineering Institute | Cat#: C011-2-1 | |
Commercial assay or kit | Wright-Giemsa stain solution | Solarbio Life Sciences | Cat#: G1020 | |
Commercial assay or kit | Trizol reagent | Life Technologies | Cat#: 15596018 | |
Commercial assay or kit | PrimeScript RT reagent kit | Takara | Cat#: RR037A | |
Commercial assay or kit | Mouse TNF-α ELISA Kit | eBioscience | Cat#:88-7324-88, RRID:AB_2575080 | |
Commercial assay or kit | Mouse IL-1β ELISA Kit | eBioscience | Cat#:88-7013-88, RRID:AB_2574946 | |
Commercial assay or kit | Mouse IL-6 ELISA Kit | eBioscience | Cat#: 88-7064-88, RRID:AB_2574990 | |
Commercial assay or kit | Mouse IL-12 ELISA Kit | MultiSciences Biotech Co., Ltd. | Cat#:70-EK212/3-96 | |
Commercial assay or kit | Mouse CXCL1 ELISA Kit | MultiSciences Biotech Co., Ltd. | Cat#:70-EK296/2-96 | |
Commercial assay or kit | Mouse CXCL2 ELISA Kit | MultiSciences Biotech Co., Ltd. | Cat#:70-EK2142/2-96 | |
Commercial assay or kit | Mouse CXCL3 ELISA Kit | Rockland | Cat#:KOA0825 | |
Commercial assay or kit | mouse CXCL10 ELISA Kit | MultiSciences Biotech Co., Ltd. | Cat#:70-EK268/2-96 | |
Antibody | Mouse monoclonal anti-FcγR blocking mAb | BD Biosciences | Clone: 2.4G2, Cat#: 553141, RRID:AB_394656 | FC (1: 100) |
Antibody | Mouse monoclonal APC/Cy7 conjugated anti-CD45 | BioLegend | Clone: 30-F11, Cat#: 103116, RRID:AB_312981 | FC (1: 100) |
Antibody | Mouse monoclonal PE conjugated anti-CD11b | BioLegend | Clone: M1/70, Cat#: 101207, RRID:AB_312790 | FC (1: 100) |
Antibody | Mouse monoclonal PE/Cy7 conjugated anti-Ly6G | BioLegend | Clone: 1A8, Cat#: 127618, RRID:AB_1877261 | FC (1: 100) |
Antibody | Mouse monoclonal FITC conjugated anti-Ly6C | BD Biosciences | Clone: AL-21, Cat#: 553104, RRID:AB_394628 | FC (1: 100) |
Antibody | Mouse monoclonal APC conjugated anti-F4/80 | BioLegend | Clone: BM8, Cat#: 123116, RRID:AB_893481 | FC (1: 100) |
Antibody | Mouse monoclonal APC conjugated anti-CD45 | BioLegend | Clone: 30-F11, Cat#: 103112, RRID:AB_312977 | FC (1: 100) |
Antibody | Mouse monoclonal FITC conjugated anti-CD3 | BD Biosciences | Clone: 17A2, Cat#: 555274, RRID:AB_395698 | FC (1: 100) |
Antibody | Mouse monoclonal APC conjugated anti-CD4 | BD Biosciences | Clone: H129.19, Cat#: 553650, RRID:AB_394970 | FC (1: 100) |
Antibody | Mouse monoclonal PE/Cy7 conjugated anti-CD8 | BioLegend | Clone: 53–6.7, Cat#: 100721, RRID:AB_312760 | FC (1: 100) |
Antibody | Mouse monoclonal PE conjugated anti-B220 | BD Biosciences | Clone: RA3-6B2, Cat#: 553090, RRID:AB_394620 | FC (1: 100) |
Antibody | Mouse monoclonal anti-Ly6G antibody | BioXcell | Clone: 1A8, Cat#: BP0075-1, RRID:AB_1107721 | In vivo depletion of neutrophils |
Antibody | Mouse monoclonal anti-CSF1R | BioXcell | Clone: AFS98, Cat#: BE0213, RRID:AB_2687699 | In vivo depletion of monocytes/macrophages |
Antibody | Rat monoclonal anti-IgG2a | BioXcell | Clone: 2A3, Cat#: BE0089, RRID:AB_1107769 | Isotype control for anti-mouse Ly6G and anti-mouse CSF1R |
Antibody | Rabbit monoclonal anti-p38 MAPK | Cell Signaling Technology | Cat#: 9,212 S, RRID: AB_330713 | WB (1: 1000) |
Antibody | Rabbit monoclonal anti-phospho-p38 MAPK | Cell Signaling Technology | Cat#: 9,211 S, RRID:AB_331641 | WB (1: 1000) |
Antibody | Rabbit monoclonal anti-ERK MAPK | Cell Signaling Technology | Cat#: 9,102 S, RRID:AB_330744 | WB (1: 1000) |
Antibody | Mouse monoclonal anti-phospho-ERK MAPK | Cell Signaling Technology | Cat#: 9,106 S, RRID:AB_331768 | WB (1: 1000) |
Antibody | Rabbit monoclonal anti-JNK MAPK Antibody | Cell Signaling Technology | Cat#: 9,252 S, RRID:AB_2250373 | WB (1: 1000) |
Antibody | Mouse monoclonal anti-phospho-JNK MAPK | Cell Signaling Technology | Cat#: 9,255 S, RRID:AB_2307321 | WB (1: 1000) |
Antibody | Rabbit monoclonal anti-NF-κB p65 | Cell Signaling Technology | Cat#: 8,242 S, RRID:AB_10859369 | WB (1: 1000) |
Antibody | Rabbit monoclonal anti-phospho-NF-κB p65 | Cell Signaling Technology | Cat#: 3,033 S, RRID:AB_331284 | |
Chemical compound, drug | Thioglycollate medium | Sigma-Aldrich | Cat#: B2551 | |
Chemical compound, drug | EGTA | Sigma-Aldrich | Cat#: 324,626 | |
Chemical compound, drug | Zymosan | Sigma-Aldrich | Cat#: Z4250 | |
Chemical compound, drug | Mueller-Hinton broth | Qingdao Rishui Biotechnologies Co., Ltd | Cat#: 11,816 | |
Chemical compound, drug | Nutrient Broth | Qingdao Rishui Biotechnologies Co., Ltd | Cat#: 10,204 | |
Chemical compound, drug | WST-8 | Cayman | Cat#: 18,721 | |
Chemical compound, drug | Ketamine hydrochloride | R&D Systems | Cat#: 3131/50 | |
Chemical compound, drug | LPS | Sigma-Aldrich | Cat#: L2630 | |
Chemical compound, drug | SB202190 | Cell Signaling Technology | Cat#: 8,158 S | |
Chemical compound, drug | U0126 | Cell Signaling Technology | Cat#: 9,903 S | |
Chemical compound, drug | SP600125 | Cell Signaling Technology | Cat#: 8,177 S | |
Chemical compound, drug | BAY11-7082 | Cell Signaling Technology | Cat#: 78,679 S | |
Chemical compound, drug | LY294002 | Cell Signaling Technology | Cat#: 9,901 S |
Cells, bacteria, and peptides
Request a detailed protocolHuman monocyte THP-1 cells and rat RBL-2H3 cells were purchased from National Collection of Authenticated Cell Cultures (https://www.cellbank.org.cn/). THP-1 cells were cultured in RPMI 1640 medium supplemented with 10% fetal bovine serum (FBS, Gibco, USA) and antibiotics (100 U/mL penicillin and 100 µg/mL streptomycin). Human THP-1 cell line has been authenticated by STR profiling (Supplementary file 4). RBL-2H3 cells were cultured in MEM medium supplemented with NaHCO3 (1.5 g/L), sodium pyruvate (0.11 g/L), 15% FBS (Gibco, USA) and antibiotics (100 U/mL penicillin and 100 µg/mL streptomycin). Peritoneal macrophages and bone marrow-derived neutrophils were isolated from C57BL/6 mice (Yang et al., 2021). C57BL/6 mice were intraperitoneally injected with sterile thioglycollate medium (4%, 2 mL). At 4 days post injection, the peritoneal macrophages were collected by flushing with DMEM medium. Mouse bone marrow was rinsed with 5 mL PBS and filtered through a cell strainer (70 micron). After centrifugation at 500 g for 5 min, the bone marrow-derived neutrophil pellet was re-suspended in 2 mL PBS. RPMI 1640 diluted Percoll gradient with 72%, 64%, and 54% layers was prepared, and cell suspension was over-layered onto this gradient. Percoll gradient was centrifuged at 950 g for 25 min. Neutrophils were collected from the 72%/64% interface, washed with PBS, and centrifuged at 500 g for 5 min. Peritoneal macrophages and neutrophils were confirmed by flow cytometry (Figure 8—figure supplement 1, Figure 10—figure supplement 1), and were cultured in DMEM and RPMI 1640 medium, respectively, supplemented with 10% FBS (Gibco, USA) and antibiotics (100 U/mL penicillin and 100 µg/mL streptomycin). Cells were maintained under an atmosphere of 5% CO2 at 37℃. Fluorescent quantitative PCR (qPCR, forward primer, 5’-GGGAGCAAACAGGATTAGATACCCT-3’, reverse primer, 5’-TGCACCATCTGTCACTCTGTTAACCTC-3’) was performed to confirm that the cell lines were negative for mycoplasma contamination.
Gram-positive bacteria, Gram-negative bacteria, and fungi were cultured at 37℃ in Luria-Bertani (LB) broth. Aquatic pathogenic bacteria were cultured at 25℃ in nutrient broth.
Synthetic peptides were purchased from Synpeptide Co. Ltd (Shanghai, China). The crude peptide was purified by reversed-phase high performance liquid chromatography (RP-HPLC) and analysed by mass spectrometry to confirm the purity higher than 98%.
Experiment animals
Request a detailed protocolBoth adult healthy tree frogs of P. puerensis (21–30 g) were captured from Pu’er, Yunnan Province, China (24.786°N, 101.362°E). P. puerensis was not endangered or protected species, and no specific permissions were required for the sampling location/activity. Tree frogs were randomly housed in freshwater tanks in a recirculating system with filtered water, fed with mealworm larvae Tenebrio molitor and refreshed with water once a day. C57BL/6 mice (female, 18–20 g) were purchased from Shanghai Slac Animal Inc, and Rag1–/– mice (female, 18–20 g) were purchased from Model Animal Research Center of Nanjing University. Mice were housed in pathogen-free facility. Animal experiments were performed in accordance with the Institutional Animal Care and Use Committee of Soochow University, and all research protocols were approved by the Animal Ethical Committee of Soochow University. All surgery of animals was performed under pentobarbital sodium anaesthesia with minimum fear, anxiety, and pain.
Mature peptide isolation
Request a detailed protocolSkin secretions were collected according to previous study (Li et al., 2007). Briefly, frogs were stimulated by anhydrous ether, and a total of about 500 mL skin secretions in PBS were quickly collected, centrifuged, and lyophilised. Lyophilised P. puerensis skin secretion was dissolved in phosphate-buffered saline (PBS, 0.1 M, pH 6.0) and separated by molecular sieving fast protein liquid chromatography (FPLC) on GE ÄKTA pure system using a Superdex 75 10/300 GL column (10 × 300 mm, 24 mL volume, GE, USA). Fractions were pooled and further purified by RP-HPLC on a C18 column (25 × 0.46 cm, Waters, USA) for two times. The eluted peaks from RP-HPLC were collected for purity assay using matrix-assisted laser desorption ionisation time-of-flight mass spectrometry (MALDI-TOF MS) on an UltraFlex I mass spectrometer (Bruker Daltonics, Germany). The amino acid sequence of the purified peptide was obtained by automated Edman degradation analysis on an Applied Biosystemspulsed liquid-phase sequencer (model ABI 491, USA).
cDNA cloning
Request a detailed protocolSkin total RNA extraction, mRNA isolation and cDNA library construction were performed according to previous methods (Wei et al., 2015). About 5.6 × 105 independent colonies were produced in the cDNA library. Two primers, an antisense primer, 5’-TTGTCTGCCTCCTCGGCTTCC-3’, designed according to the conserved domain of amphibian cathelicidins, and the 5’ PCR primer, 5’-AAGCAGTGGTATCAACGCAGAGT-3’ supplied by cDNA library construction kit, were used to clone the 5’ fragment that encoding the precursor of PopuCATH. The full length cDNA encoding the precursor of PopuCATH was obtained by a sense primer, 5'-ATGGCGCTCGCTGCTGCACTC-3' designed according to the 5’ fragment of PopuCATH precursor, and 3’ PCR primer, 5'-ATTCTAGAGGCCGAGGCGGCCG-3’ provided by the kit. PCR procedure for cDNA cloning was 95 ℃ for 5 min, and 30 cycles of 95 ℃ for 30 s, 56 ℃ for 30 s, 72 ℃ for 1 min, followed by an extension step at 72 ℃ for 8 min.
Toxic side effects to mammalian cells and mice
Request a detailed protocolFor cytotoxicity assay, mouse peritoneal macrophages or THP-1 cells were seeded into 96-well plates (5 × 105 cells/well, 200 µL). PopuCATH (25, 50, 100, and 200 μg/mL) was added to each well. After culture for 24 h, 10 µL of CCK-8 reagent was added to each well. The absorbance at 450 nm was recorded on a microplate reader after incubation for 1 h (Yang et al., 2021).
For hemolysis assay, mouse erythrocytes and rabbit erythrocytes were washed with 0.9% saline and incubated with a series of two-fold dilutions of PopuCATH (25, 50, 100, and 200 μg/mL) at 37 ℃. After incubation for 30 min, the erythrocytes were centrifuged at 1,000 g for 5 min and monitored at 540 nm. Triton X-100 (1%) treatment was determined as 100% hemolysis. Hemolytic activity was expressed as the percentage of the Triton X-100-treated group (Wei et al., 2013).
For immunogenicity assay, mesenteric lymph nodes (MLN) and spleen were collected and filtered through at 70 μm cell strainer (Falcon, Corning, USA). After erythrocytes were lysed with ACK Lysis Buffer (Solarbio, Beijing, China) for 5 min, cells were suspended in RPMI 1640 (2%FBS), and added to 96-well plates (5 × 104 cells/well, 200 µL). A final concentration of 25, 50, 100, or 200 μg/mL of PopuCATH, or 2 μg/mL of concanavalin A (Con A, Sigma-Aldrich, Shanghai, China) was added and incubated at 37℃ for 24 h. CCK-8 reagent (10 µL, Dojindo, Shanghai, China) was added. After incubation at 37℃ for 1 h, the absorbance at 450 nm was measured on a microplate reader (Mendez et al., 2005).
For hypersensitivity assay, RBL-2H3 cells were seeded in 96-well plates (2 × 104 cells/well, 200 µL) and cultured overnight. A final concentration of 25, 50, 100, or 200 μg/mL of PopuCATH or human cathelicidin LL-37 (positive control) was added and incubated at 37℃ for 0.5 hr. The supernatant was collected and incubated with 4-nitrophenyl-N-acetyl-B-D-glucosaminide substrate at 37℃ for 1 hr. The absorbance at 405 nm was measured on a microplate reader (Scott et al., 2007).
For complement assay, mouse serum was treated with PBS, EGTA inhibitor (10 mM, Sigma-Aldrich, Shanghai, China), zymosan (0.5 mg/mL, Sigma-Aldrich, Shanghai, China), PopuCATH (25, 50, 100, and 200 μg/mL) at 37℃ for 1 hr. C3a des-Arg was measured by ELISA (Wuhan Fine Biotech, China) (Scott et al., 2007).
For in vivo acute toxicity assay, C57BL/6 (female, 18–20 g, n = 6) were intraperitoneally injected with PopuCATH at dose of 10, 20, and 40 mg/kg, respectively. At 24 hr post injection, kidneys, livers, hearts and spleens were collected for H&E staining. The alanine aminotransferase (ALT) and creatinine in the serum were measured by ALT assay kit (Nanjing Jiancheng Bioengineering Institute, China) and the creatinine assay kit (Nanjing Jiancheng Bioengineering Institute, China), respectively (Yu et al., 2017).
In vitro antimicrobial assay
Request a detailed protocolA standard two-fold broth microdilution method was used to evaluate the MIC of PopuCATH against microbes. Gram-positive bacteria, Gram-negative bacteria, and fungi were diluted with Mueller-Hinton broth, and aquatic pathogenic bacteria were diluted with nutrient broth to 105 CFU/mL. Series of two-fold PopuCATH dilutions were prepared in 96-well plates (50 μL/well). An equal volume of microbial dilution was added and cultured at 37℃ (for Gram-positive bacteria, Gram-negative bacteria, and fungi) or 25℃ (for aquatic pathogenic bacteria) for 18 hr. Cathelicidin-PY from P. yunnanensis served as positive control. The minimal concentrations at which no visible growth of microbes occurred were defined as MIC values (Wei et al., 2013).
Bacterial killing kinetics were examined as described previously (Wei et al., 2013). Microbes in exponential phase were diluted in Mueller-Hinton broth (E. coli ATCC25922, S. aureus ATCC25923, and C. albicans ATCC2002) or nutrient broth (A. hydrophila) at density of 105 CFU/mL. PopuCATH (200 μg/mL), cathelicidin-PY (PY, 1× MIC) or an equal volume of PBS (solvent of peptide) was incubated with microbial dilution at 37℃ or 25℃ for 0, 1, 2, 3, and 4 hr, respectively. At each time point, mixture of peptide and microbe was diluted in Mueller-Hinton or nutrient broth for 1000 folds, and microbial dilution (50 μL) was coated on Mueller-Hinton or nutrient broth agar plates. Microbial colonies were counted after culture at 37℃ or 25℃ for 12 hr.
Microbial metabolic activities were assayed according previous method (Scott et al., 2007). E. coli ATCC25922, S. aureus ATCC25923, C. albicans ATCC2002, and A. hydrophila in exponential phase were diluted in DMEM at density of 105 CFU/mL, and PopuCATH (200 μg/mL), cathelicidin-PY (PY, 1× MIC) or PBS (solvent of peptide) was added. Microbial dilution (100 μL/well) was added to 96-well plates. After the addition of WST-8 (10 μL/well, Cayman, Ann Arbor, USA), the plates were incubated at 37℃ or 25℃ for 1, 2, 3, and 4 hr, and absorbance was monitored at 255 nm. Metabolic activity was expressed as the percentage of the PBS-treated group.
Scanning electron microscope (SEM) assay was used to examine if PopuCATH impairs the bacterial surface morphology. E. coli ATCC25922 and S. aureus ATCC25923 were cultured in Mueller-Hinton broth to exponential phase, washed and diluted using PBS (105 CFU/mL). PopuCATH (200 μg/mL), cathelicidin-PY (PY, 1× MIC) or PBS was added into the bacterial dilution and incubated at 37℃. After incubation for 30 min, bacteria were centrifuged (1000 g for 10 min) and fixed for SEM assay according to standard operating protocols. The bacterial surface morphology was observed using a Hitachi SU8010 SEM (Wei et al., 2013).
In vivo antimicrobial assay
Request a detailed protocolIn tree frogs (n = 5, 21–30 g), PopuCATH (10 mg/kg) was intraperitoneally injected at 8 or 4 hr prior to (–8 or –4 hr), or 4 hr after ( + 4) S. aureus ATCC25923 inoculation (108 CFU/frog, intraperitoneal injection). At 18 hr post bacterial challenge, peritoneal lavage was collected for bacterial load assay.
In C57BL/6 mice (female, 18–20 g, n = 6), PopuCATH (10 mg/kg) was intraperitoneally injected at eight or 4 hr prior to (–8 or –4 hr), or 4 hr after ( + 4) Gram-negative (E. coli, A. baumannii) or Gram-positive (S. aureus or methicillin-resistant S. aureus, MRSA) bacterial inoculation (2 × 107 CFUs/mouse, intraperitoneal injection). At 18 hr post bacterial inoculation, peritoneal lavage was collected for bacterial load assay, serum was collected for cytokine assay, and lungs were taken for histopathological assay (Yang et al., 2021).
In order to further investigate its prophylactic efficacy against bacterial infection, PopuCATH (10 mg/kg) was given through intravenous or intramuscular injection at 4 hr prior to E. coli inoculation (2 × 107 CFUs/mouse, intraperitoneal injection). At 18 hr post bacterial inoculation, peritoneal lavage was collected for bacterial load assay.
The protective efficacy of PopuCATH was also evaluated in septic mice induced by a lethal bacterial inoculation or CLP. For lethal bacterial challenge, C57BL/6 mice (female, 18–20 g, n = 6) were intraperitoneally injected with PopuCATH (10 mg/kg) 4 hr prior to E. coli (4 × 107 CFUs/mouse, intraperitoneal injection) or MRSA (6 × 108 CFUs/mouse, intraperitoneal injection) inoculation. The survival rates of mice were monitored for 7 days (Yang et al., 2021). To compare the protective efficacy of PopuCATH with other peptides, the protective efficacy of LL-37 and IDR-1 were simultaneously evaluated at the same condition. For CLP-induced sepsis, C57BL/6 mice (female, 18–20 g, n = 6) were intraperitoneally injected with PopuCATH (10 mg/kg) at 8 and 4 hr (two times) prior to CLP. At 4 hr post the last injection of PopuCATH, mice were anaesthesied with ketamine hydrochloride (100 mg/kg), and the abdominal cavity of mice was opened in layers. The cecum was ligated 1.0 cm from the end, a through-and-through puncture was operated using an 18-gauge needle. A small droplet of faeces was extruded for ensuring the patency of the puncture site. Then, the cecum was returned back to the abdominal cavity. A laparotomy but no CLP mice served as control. After CLP, the survival rates of mice were monitored for 7 days (Yang et al., 2021).
In vivo chemotaxis assay
Request a detailed protocolC57BL/6 mice (female, 18–20 g, n = 6) were intraperitoneally injected with PopuCATH (10 mg/kg) dissolved in 0.2 mL PBS. The same volumes of PBS and LPS (20 μg/mouse, from E. coli O111:B4, Sigma-Aldrich, Shanghai, China) were used as negative control and positive control, respectively. Cells in peritoneal lavage and peripheral blood were collected at 4 and 8 hr post injection, respectively. For chemotactic kinetics assay, cells in peritoneal lavage and peripheral blood were collected at 4, 8, 24, 48, 72 hr post injection, respectively. Collected cells were incubated with anti-FcγR blocking mAb (clone 2.4G2). After incubation at 4℃ for 30 min, cells were washed with PBS and re-suspended in PBS. For myeloid cell analysis, cells were stained with APC-Cy7/anti-CD45 (clone 30-F11), PE/anti-CD11b (clone M1/70), PE/Cy7/anti-Ly-6G (clone 1A8), FITC/anti-Ly6C (clone AL-21), and APC/F4/80 (clone BM8) at 4℃ for 30 min. For lymphoid cell analysis, cells were stained with APC/anti-CD45 (clone 30-F11), FITC/anti-CD3 (clone 17A2), ACP-Cy7/anti-CD4 (clone H129.19), PE/Cy7/ anti-CD8 (clone 53–6.7), and PE/B220 (clone RA3-6B2) at 4℃ for 30 min. The stained cells were washed and analysed by a flow cytometer FACS Canto II (BD Biosciences) with FlowJo seven software (Tree Star) (Scott et al., 2007; Yang et al., 2021).
P. puerensis (21–30 g, n = 5) were intraperitoneally injected with PopuCATH (10 mg/kg, dissolved in 0.2 mL PBS) or PBS. At 4 and 8 hr post injection, total cells in peritoneal lavage were counted using a hemocytometer, and the cells were observed under an optical microscope after Wright-Giemsa staining.
Protective efficacy of PopuCATH in myeloid or lymphoid cell-deficient mice
Request a detailed protocolNeutrophils and monocytes/macrophages were depleted by intraperitoneal injection of anti-Ly6G antibody and anti-CSF1R antibody, respectively (Yang et al., 2021). Anti-Ly6G antibody (500 µg/mouse) or anti-rat IgG2a isotype antibody was injected into C57BL/6 mice (female, 18–20 g, n = 6) on day 0, and day 2, respectively. Anti-CSF1R antibody or anti-rat IgG2a isotype antibody was injected into C57BL/6 mice (female, 18–20 g, n = 6) at doses of 1 mg per mouse on day 0 followed by 0.3 mg per mouse on day 1 and day 2, respectively.
PopuCATH (10 mg/kg) was intraperitoneally injected into neutrophil-depleted mice (on day 3), monocyte/macrophage-depleted mice (on day 3), and Rag1–/– mice (female, 18–20 g, n = 6) 4 hr prior to E. coli or S. aureus inoculation (2 × 107 CFUs/mouse, intraperitoneal injection). At 18 hr post bacterial challenge, the peritoneal lavage was collected for bacterial load assay (Yang et al., 2021).
In vitro chemotaxis assay
Request a detailed protocolNeutrophils or macrophages were suspended in RPMI 1640 (5 × 106 cells/mL, 2% FBS), and 100 µL of cell suspension was added to the 3.0 µm (for neutrophils) or 5.0 µm (for macrophages) pore-size Transwell filters (the upper chamber) in a 24-well format. A total of 500 µL of PopuCATH (10 µM, dissolved in 2% FBS RPMI 1640 medium) or medium was added to the lower chamber. After culture at 37℃ for 8 hr, neutrophils or macrophages in the lower chamber were counted using a hemocytometer. The increased cells in the lower chamber were determined as the migrated cells (Yang et al., 2021).
For the co-cultured system, 500 µL of macrophage suspension in RPMI 1640 (5 × 106 cells/mL, 2% FBS) were added to the lower chamber, and 100 µL of neutrophil or macrophage suspension in RPMI 1640 (5 × 106 cells/mL, 2% FBS) was added to the 3.0 µm (for neutrophils) or 5.0 µm (for macrophages) pore-size Transwell filters (the upper chamber). A total of 500 µL of PopuCATH (10 µM, dissolved in medium) or medium was added to the lower chamber. After culture at 37℃ for 8 hr, neutrophils or macrophages in the upper chamber were counted using a hemocytometer. The reduced cells in the upper chamber were determined as the migrated cells (Yang et al., 2021).
Regulatory effects of PopuCATH on macrophages
Request a detailed protocolFor chemokine/cytokine production assay, macrophages were seeded in 24-well plates (5 × 105 cells/well, 2%FBS DMEM) and cultured with PopuCATH (5, 10, and 20 µM). After incubation at 37℃ for 4 hr, the cells were collected for total RNA extraction using Trizol reagent. SYBR green qPCR master mix was used to for two-step qPCR assay after cDNA synthesis using PrimeScript RT reagent kit. Gene expression was normalised to the expression level of Actb. Primers for qPCR were listed in Supplementary file 5. The supernatant was collected for chemokine/cytokine production determination using ELISA kits (Yang et al., 2021).
For signaling pathway assay, macrophages seeded in 24-well plates (5 × 105 cells/well, 2%FBS DMEM) were pre-incubated with inhibitor of p38 (SB202190, 10 µM), ERK (U0126, 10 µM), JNK (SP600125, 10 µM), NF-κB (BAY11-7082, 2 µM), or PI3K (LY294002, 10 µM) for 1 hr, respectively, and PopuCATH (10 µM) was added and incubated for 4 hr. Chemokine production in the supernatant were quantified by ELISA. Next, macrophages were plated to six-well plates (2 × 106/well, 2%FBS DMEM) and cultured with PopuCATH (10 µM) for 15, 30, and 45 min, respectively. LPS (100 ng/mL, incubation for 30 min) served as positive control. Macrophages were lysed for detecting the protein levels of p38, phospho-p38, ERK, phospho-ERK, JNK, phospho-JNK, p65, and phospho-p65 by western blot analysis (Yang et al., 2021).
For macrophage phagocytosis assay, macrophages were pre-incubated with PopuCATH (10 µM) for 2 hr, S. aureus and E. coli bacteria were preloaded with 10 μM CFSE fluorescent dye (Molecular Probes, Invitrogen) in PBS for 30 min at 37℃. Bacteria were killed by incubation with 1% paraformaldehyde in PBS for 1 hr at 37℃, and washed five times in PBS. Macrophages were incubated with the CFSE-loaded bacterial particles at multiplicity of infection 100. After incubation for indicated time points, the cells were washed, the extracellular fluorescence were quenched with trypan blue (15 mg/mL) in PBS, and the CFSE fluorescence were analysed by flow cytometry as a measure of the phagocytic uptake of the bacterial particles (Nijnik et al., 2010).
Regulatory effects of PopuCATH on neutrophils
Request a detailed protocolThe effects of PopuCATH (10 µM) on neutrophil phagocytosis was assayed similar with macrophages phagocytosis as mentioned above. Neutrophils were pre-incubated with PopuCATH (10 µM) for 2 hr, and were incubated with CSFE-loaded S. aureus and E. coli particles at multiplicity of infection 100. At indicated time points, CFSE fluorescence were analysed by flow cytometry (Nijnik et al., 2010).
For neutrophil extracellular traps (NETs) assay, neutrophils suspended in 2% FBS RPMI 1640 was seeded in an eight well-cover slip chamber (200 μL/well, 1 × 106 cells/mL). PopuCATH (10 µM), PBS, or PMA (100 nM) was added and incubation at 37℃ for 4 hr. Neutrophils were stained with DAPI (Invitrogen, USA) or anti-Cit-H3 (Abcam, USA), respectively. Nuclei and NETs were observed under a confocal microscope ( × 60, Nikon, Japan) (He et al., 2019).
Statistical analysis
Request a detailed protocolData were represented as mean ± SD. Statistical significance was determined by an unpaired two-tailed Student’s t tests for two-group comparison, and was determined by ANOVA followed by Bonferroni post hoc analysis f or multiple-group comparison. All statistical analysis was performed using GraphPad Prism software version 5.0. A p value less than 0.05 was considered as statistically significant.
Data availability
Sequencing data have been deposited in GenBank (accession number: KY391886). All data generated or analysed during this study are included in the manuscript, supporting files, and source data.
-
NCBI GenBankID KY391886. Rhacophorus puerensis cathelicidin precursor, mRNA, complete cds.
References
-
Cod cathelicidin: isolation of the mature peptide, cleavage site characterisation and developmental expressionDevelopmental and Comparative Immunology 35:296–303.https://doi.org/10.1016/j.dci.2010.10.002
-
LL-37, the neutrophil granule- and epithelial cell-derived cathelicidin, utilizes formyl peptide receptor-like 1 (FPRL1) as a receptor to chemoattract human peripheral blood neutrophils, monocytes, and T cellsThe Journal of Experimental Medicine 192:1069–1074.https://doi.org/10.1084/jem.192.7.1069
-
Characteristics of cathelicidin-Bg, a novel gene expressed in the ear-side gland of Bufo gargarizansGenetics and Molecular Research 15:15038481.https://doi.org/10.4238/gmr.15038481
-
Anti-infection peptidomics of amphibian skinMolecular & Cellular Proteomics 6:882–894.https://doi.org/10.1074/mcp.M600334-MCP200
-
Naturally occurring antimicrobial peptide OH-CATH30 selectively regulates the innate immune response to protect against sepsisJournal of Medicinal Chemistry 56:9136–9145.https://doi.org/10.1021/jm401134n
-
Modulation of the TLR-mediated inflammatory response by the endogenous human host defense peptide LL-37Journal of Immunology (Baltimore, Md) 176:2455–2464.https://doi.org/10.4049/jimmunol.176.4.2455
-
A potential wound-healing-promoting peptide from salamander skinFASEB Journal 28:3919–3929.https://doi.org/10.1096/fj.13-248476
-
Neutrophils and immunity: challenges and opportunitiesNature Reviews. Immunology 6:173–182.https://doi.org/10.1038/nri1785
-
The antimicrobial peptide LL-37 facilitates the formation of neutrophil extracellular trapsThe Biochemical Journal 464:3–11.https://doi.org/10.1042/BJ20140778
-
Synthetic cationic peptide IDR-1002 provides protection against bacterial infections through chemokine induction and enhanced leukocyte recruitmentJournal of Immunology (Baltimore, Md 184:2539–2550.https://doi.org/10.4049/jimmunol.0901813
-
The innate defense regulator peptides IDR-HH2, IDR-1002, and IDR-1018 modulate human neutrophil functionsJournal of Leukocyte Biology 94:159–170.https://doi.org/10.1189/jlb.1012497
-
Novel peptide therapeutics for treatment of infectionsJournal of Medical Microbiology 58:977–987.https://doi.org/10.1099/jmm.0.011122-0
-
Antimicrobial peptides: natural effectors of the innate immune systemSeminars in Immunopathology 29:27–43.https://doi.org/10.1007/s00281-007-0064-5
-
An anti-infective peptide that selectively modulates the innate immune responseNature Biotechnology 25:465–472.https://doi.org/10.1038/nbt1288
-
Defensins: antifungal lessons from eukaryotesFrontiers in Microbiology 5:97.https://doi.org/10.3389/fmicb.2014.00097
-
Structure and Function of a Potent Lipopolysaccharide-Binding Antimicrobial and Anti-inflammatory PeptideJournal of Medicinal Chemistry 56:3546–3556.https://doi.org/10.1021/jm4004158
-
The first anionic defensin from amphibiansAmino Acids 47:1301–1308.https://doi.org/10.1007/s00726-015-1963-8
-
The Chemistry and Biological Activities of Peptides from Amphibian Skin SecretionsChemical Reviews 115:1760–1846.https://doi.org/10.1021/cr4006704
-
Identification of the first cathelicidin gene from skin of Chinese giant salamanders Andrias davidianus with its potent antimicrobial activityDevelopmental and Comparative Immunology 77:141–149.https://doi.org/10.1016/j.dci.2017.08.002
-
EGCG Induces Pro-inflammatory Response in Macrophages to Prevent Bacterial Infection through the 67LR/p38/JNK Signaling PathwayJournal of Agricultural and Food Chemistry 69:5638–5651.https://doi.org/10.1021/acs.jafc.1c01353
-
Structure and biology of cathelicidinsAdvances in Experimental Medicine and Biology 479:203–218.https://doi.org/10.1007/0-306-46831-X_17
-
Cathelicidins, multifunctional peptides of the innate immunityJournal of Leukocyte Biology 75:39–48.https://doi.org/10.1189/jlb.0403147
Article and author information
Author details
Funding
National Natural Science Foundation of China (31870868)
- Lin Wei
National Natural Science Foundation of China (31970418)
- Hailong Yang
National Natural Science Foundation of China (81373380)
- Hailong Yang
National Natural Science Foundation of China (81802023)
- Jing Wu
Key Research & Development Plan in Social Development of Jiangsu Province (BE2020652)
- Lin Wei
Priority Academic Program Development of Jiangsu Higher Education Institutions
- Lin Wei
The funders had no role in study design, data collection and interpretation, or the decision to submit the work for publication.
Ethics
Animal experiments were performed in accordance with the Institutional Animal Care and Use Committee of Soochow University, and all research protocols were approved by the Animal Ethical Committee of Soochow University. All surgery of mice was performed under pentobarbital sodium anesthesia with minimum fear, anxiety and pain.
Copyright
© 2022, Yang et al.
This article is distributed under the terms of the Creative Commons Attribution License, which permits unrestricted use and redistribution provided that the original author and source are credited.
Metrics
-
- 746
- views
-
- 105
- downloads
-
- 21
- citations
Views, downloads and citations are aggregated across all versions of this paper published by eLife.
Citations by DOI
-
- 21
- citations for umbrella DOI https://doi.org/10.7554/eLife.72849