The Natural History of Model Organisms: The big potential of the small frog Eleutherodactylus coqui
Abstract
The Puerto Rican coquí frog Eleutherodactylus coqui is both a cultural icon and a species with an unusual natural history that has attracted attention from researchers in a number of different fields within biology. Unlike most frogs, the coquí frog skips the tadpole stage, which makes it of interest to developmental biologists. The frog is best known in Puerto Rico for its notoriously loud mating call, which has allowed researchers to study aspects of social behavior such as vocal communication and courtship, while the ability of coquí to colonize new habitats has been used to explore the biology of invasive species. This article reviews existing studies on the natural history of E. coqui and discusses opportunities for future research.
Introduction
On the Caribbean island of Puerto Rico the night is filled with high-pitched calls of “¡Ko-kee!” as the coquí común – the common or Puerto Rican coquí (Eleutherodactylus coqui) – sings its song across the island. This small but boisterous frog is a national symbol of Puerto Rico and has featured prominently in the island’s culture for thousands of years (Joglar, 1998). The indigenous Taíno people believe a goddess created the frog to forever call out the name of her lost love, Coquí (pronounced ko-kee or co-qui), who was taken from her by the god of chaos and disorder. The coquí frog remains as celebrated today as it was by the ancient Taíno people, and the Taíno symbol for the frog (Figure 1) appears in everything from artwork and pottery to the marketing and branding many of Puerto Rican companies. Indeed, the coquí frog is so important to the identity of Puerto Ricans that they often express their nationality by saying “Soy de aquí como el coquí” (“I’m from here, like the coquí”).

The indigenous Taíno symbol for coquí, which is ubiquitous in Puerto Rico.
The frog-like hands seen in Taíno imagery are associated with ‘femaleness’ and the calls of coquí are associated with female fertility and children (Ostapkowicz, 2015).
However, in addition to being an important cultural symbol in Puerto Rico, E. coqui has also become an important species for scientific inquiry, and researchers from a number of fields have been drawn to the coquí frog as a result of its natural history, conspicuous behavior, and sheer abundance. This combination of traits has made the coquí frog an excellent model for the study of developmental biology, neuroethology (notably in the areas of mate attraction, communication, and auditory processing) and invasion biology. Here, we give an overview of the life history of the coquí frog, discuss its unique role in three major fields of biology, outline research with coquí in other areas (Box 1), and highlight open questions about the natural history of the coquí frog and its relatives.
Other notable coquí research
Fossil evidence
As one of the most speciose vertebrate genera, Eleutherodactylids have a long evolutionary history. In fact, a distal humerus of an Eleutherodactylid found in Puerto Rico appears to be the earliest known fossil frog from any Caribbean island, with an estimated age of ~29 Mya (Oligocene; Blackburn et al., 2020). Molecular phylogenies estimate the genus of Eleutherodactylus diverged ~57 Mya (Heinicke et al., 2007; Pyron, 2014) and were present in the islands of the Greater Antilles by the mid-Cenozoic (~30 Mya; Heinicke et al., 2007). E. coqui, specifically, are estimated to have diverged from their nearest common ancestor ~7.4-12.8 Mya (Pyron, 2014; Hedges et al., 2015).
Extra tympanic hearing
Vocal communication is central to the lives of most frogs that – like coquí – use sound as their central mode of communication. Effective communication, therefore, requires effective detection and localization of (conspecific) sounds. Sound localization in many species relies on neural comparisons of detection timing between ears, making two observations in diverse frogs very surprising. First, even species with very tiny heads, including E. coqui, are capable of accurate sound localization. Second, some frogs and toads perform sound localization in the complete absence of external ears (i.e. eardrums or tympanic membranes). These perplexing observations led to the hypothesis that there must be accessory, extra tympanic pathways for hearings. Studies in E. coqui were some of the first to solve this puzzle by confirming the existence of accessory hearing via the body wall and lungs (Ehret et al., 1990; Narins et al., 1988).
Coquí in bioengineering
To make their impressive vocalizations, coquí frogs expand and contract their gular skin with remarkably high extensibility, elongating up to 400% for males (337% for females) with an ultimate tensile strength of 1.7 MPa, meaning the tissue is easily extensible. In comparison, the gular tissue of Xenopus laevis, which do not have vocal sacs, elongates up to 104% and requires much more force to extend with an ultimate tensile strength of ~6.3 MPa. This tissue has inspired research on the structure and molecular mechanisms of the coquí gular skin tissue compliance with implications for using biomimicry in development of more compliant biomaterials in regenerative medicine, such as 3D printed bladder tissues (Hui et al., 2020).
Life history of the coquí
Common coquí are small, nocturnal, terrestrial frogs (Thomas, 1965). The snout-vent length of a coquí is up to 63 mm for adult females and 50 mm for adult males. Coquí are gray brown, with natural variation in color and striping among populations (Figure 2A–C; Beard et al., 2009; Woolbright and Stewart, 2008). This polymorphic variation is driven by local habitat matching and reduces predation risk by apostatic selection, where predators have a search image of the common morph, thus favoring survival of the rarer morphs (Woolbright and Stewart, 2008). Coquí have clutches of 10–40 eggs that are fertilized internally and brooded on land (Elinson et al., 1990). Males guard eggs as they develop directly from egg to froglet, skipping the free-swimming tadpole stage observed in most frogs (Townsend, 1986; Townsend and Stewart, 1985). The small size of coquí froglets makes them vulnerable to predation by many invertebrates such as the giant crab spider (Olios spp.; Formanowicz et al., 1981).
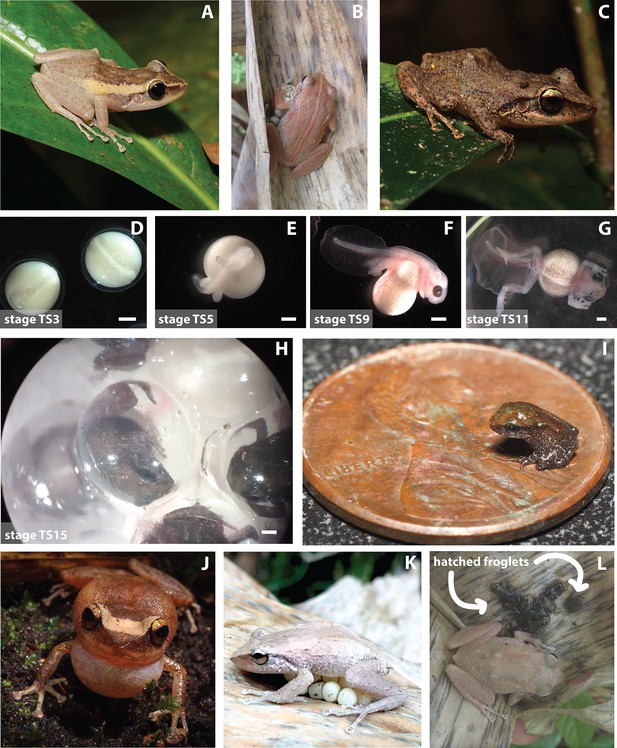
Different aspects of coquí natural history.
(A–C) Coquí vary in color and pattern across different localities. (D–H) Coquí are direct developers, skipping the tadpole stage in their early development from an egg to a froglet. Townsend-Stewart (TS) described coquí development in 15 stages. Scale bars are 1 mm. (I) Froglets are quite small when they hatch and are at risk of predation by invertebrates. (J) Coquí are particularly known for their noisy calls made with their elastic vocal sacs. (K–L) Male coquí sit on their terrestrial eggs to hydrate them and will guard their newly hatched froglets.
Image credits: (A, C) S Van Belleghem (B, K–L) K Harmon (D–H) M Laslo (I) C Brown, USGS (J) A Lopez.
Coquí are generalists occurring in a wide range of habitats, including forests, mountains, and urban areas in tropical regions of the Americas. Since E. coqui are terrestrial and lay eggs on land, they are not constrained by needing bodies of water for reproduction, provided sufficient humidity (Beard and Pitt, 2012). Coquí show a shift in microhabitat preference during development. While adults can be found across the whole vertical spectrum, from leaf litter to canopies, juveniles are more often found in the understory and avoid leaf litter (Beard et al., 2003). At night, adult coquí prefer large-leafed plants that can support their weight for calling and foraging and will use large fallen leaves for nesting and diurnal retreat sites (Beard et al., 2003; Townsend, 1989). Coquí are primarily insectivores and opportunistic feeders of abundant prey (Beard, 2007; Stewart and Woolbright, 1996; Woolbright and Stewart, 1987).
In their native Puerto Rico, coquí live at high densities. Estimates vary but can be as high as ~50,000 frogs/hectare (Beard et al., 2008; Stewart and Woolbright, 1996; Woolbright et al., 2006). Populations of coquí are estimated to consume up to ~690,000 invertebrates/hectare each night (Beard et al., 2008). Population densities are impacted by rainfall, the number of available territories, and disruptive weather events such as hurricanes (Stewart and Pough, 1983; ). As habitat generalists living closely with humans, and liberated from water for reproduction thanks to terrestrial breeding, coquí have readily invaded other Caribbean and Pacific islands through accidental introductions (Figure 3 and detailed discussion below; Beard and Pitt, 2012; Stewart and Woolbright, 1996). Indeed, a single male brooding a clutch of eggs in a potted plant may be sufficient to establish a new population (Figure 3 photo inset).
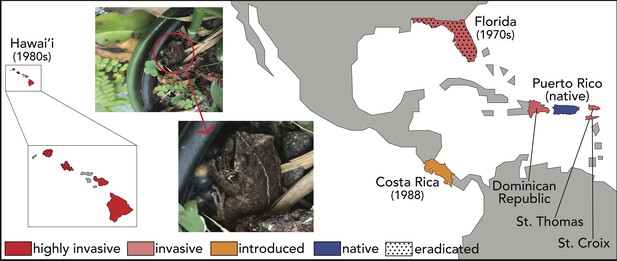
Distribution of the coquí.
Native to Puerto Rico, E. coqui have successfully invaded many tropical islands and countries. Close to home, coquí have been introduced to other Caribbean islands, including the Culebra and Vieques Islands of Puerto Rico (Rivero and Joglar, 1979), St. Thomas and St. Croix in the Virgin Islands (MacLean, 1982), and the Dominican Republic (Joglar, 1998). Farther afield, coquí were accidentally introduced to Florida in the 1970s (Austin and Schwartz, 1975; Wilson and Porras, 1983) and Hawai’i in the late 1980s (Velo-Antón et al., 2007), presumably as hitch-hikers on ornamental plants (photo inset). An intentional introduction of E. coqui was documented in Costa Rica, where six individuals were released in a private garden in 1998 and have since spread (Barrantes-Madriga et al., 2019). Floridian and some Hawaiian populations have been successfully eradicated due to inhospitable environmental conditions (Florida) and human effort (Hawai’i), but reintroductions remain a documented concern.
Image credit: RN Tischler.
Development
The direct-developing life cycle of coquí is dramatically different from the ancestral metamorphosing life cycle observed in most living frogs. Therefore, E. coqui is an important model for understanding the evolutionary origins and consequences of direct development. Direct-developing frogs skip the free-swimming tadpole stage characteristic of most frogs, including Xenopus laevis, the most common model of amphibian development. Instead, direct-developing frogs hatch as miniature adults (Figure 2D–H).
Direct development has evolved independently multiple times in frogs (Duellman and Trueb, 1994; Gomez-Mestre et al., 2012; Figure 4A), suggesting that this life history can be advantageous. This is likely because – especially when coupled with parental care as in coquí (see below) – direct-developing frogs are freed from the requirement of water for breeding.
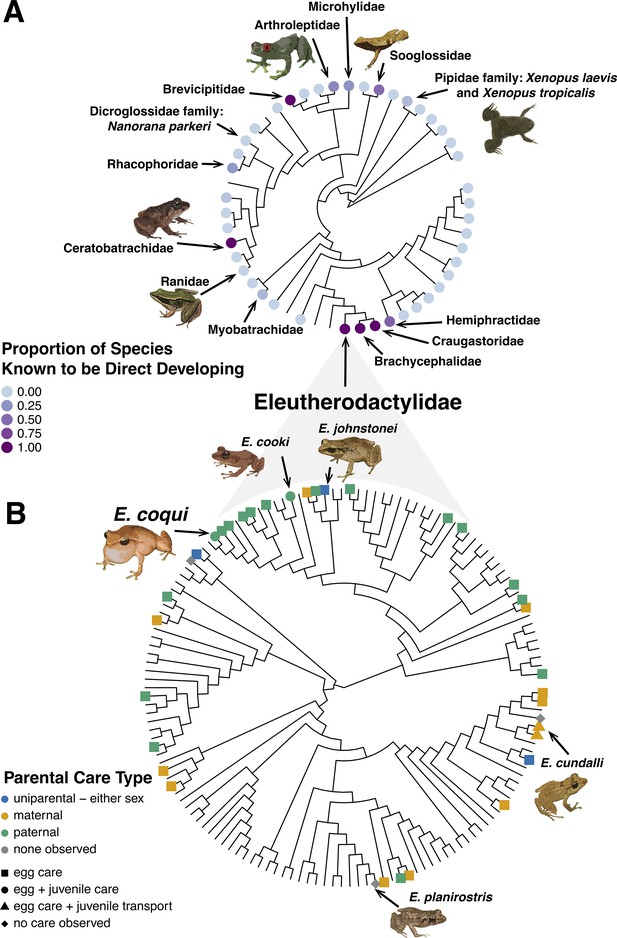
Evolution of direct development and parental care type.
(A) Phylogenetic tree showing that, across Anura, there have been 11 independent evolutionary occurrences of direct development. Evidence suggests that the clade that includes Eleutherodactylidae, Craugastoridae, and Hemiphractidae is one of the oldest direct developing lineages, having evolved ~71–108 MYA (see Heinicke et al., 2009 and Gomez-Mestre et al., 2012 more details on the evolution of direct development). Other instances of direct development appear to have emerged more recently. (B) Phylogenetic tree showing that, across the family Eleutherodactylidae, there is variation in parental care strategies, including maternal, paternal, and amphisexual. E. coqui sits within a larger clade with mostly paternal egg care; additionally, male coquí care for froglets. E. coqui is one of two Eleutherodactylid species known to show paternal egg and juvenile care (Furness and Capellini, 2019). Currently, data is too sparse and varied to determine what specific parental care strategy was used by the ancestor of Eleutherodactylids; however, parental care is generally associated with direct development and a terrestrial life history (Gomez-Mestre et al., 2012; Vági et al., 2019). Phylogenetic clade relationships created from Pyron, 2014 time tree data. Direct development and behavior traits mapped on phylogenies with data from Furness and Capellini, 2019.
Several immediate questions arise about this unique life history: Is early development and patterning (the process by which equivalent cells take on different identities) the same in direct-developing and metamorphosing frogs? To what extent do direct-developing frogs repeat tadpole development within the egg? In coquí, the answer seems to be that development is a mix of conserved and novel features. This unique combination of developmental features has made coquí an important model for the evolution of development, as well as a model for the evolution of the amniote egg, a key innovation in the evolutionary diversification of vertebrates.
Early development
While fertilization in most frogs is external, fertilization occurs internally in coquí (Townsend et al., 1981; Townsend and Stewart, 1985). Because there is no independently feeding tadpole stage, the egg must contain all the nutrients needed to get the embryo to the juvenile froglet stage. As a result, coquí eggs are large: 3.5 mm as compared to the 1.3 mm eggs of Xenopus laevis.
Frogs in general have holoblastic, or complete, cleavage of the egg. Coquí maintain this complete cleavage, but the large egg shifts the cleavage furrows towards the animal pole (top), resulting in a larger vegetal (bottom) section (Figure 5). This altered cleavage pattern in turn shifts other developmental events (such as germ layer formation and neurulation) towards the animal pole (Figure 5; Ninomiya et al., 2001) and impacts the distribution of several early patterning RNAs (Beckham et al., 2003; Fang et al., 2000; Fang and Elinson, 1999).
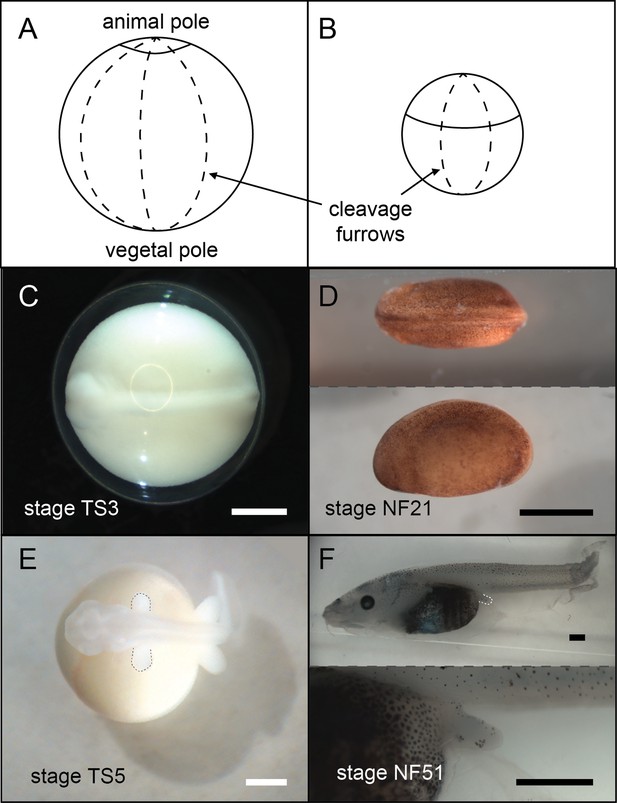
A comparison of E.coqui (A, C, E) and X. laevis (B, D, F) development.
(A–B) Drawings of embryos after the first horizontal division. This horizontal division divides the embryo into animal (top) and vegetal (bottom) cells. The cap of E. coqui animal cells is much smaller than the animal cells in the early X. laevis embryo. (C) Dorsal view of the E. coqui neurula, which is shifted towards the animal pole compared to the X. laevis neurula (D; dorsal and lateral views shown). (E–F) Morphologically equivalent limb bud stages of the coquí embryo (E) and the X. laevis embryo (F). The coquí embryo is atop a large yolk mass, while limb buds emerge from a free-swimming X. laevis tadpole. Scale bars are 1 mm.
Image credit: M Laslo.
Despite these differences, some early developmental features are conserved with metamorphosing frogs; germ cells are still located at the vegetal pole (Figure 5; Elinson et al., 2011) and neural crest cell migration is similar between E. coqui and metamorphosing frogs (Moury and Hanken, 1995). Xenopus is one of four model organisms (chick, mouse, zebrafish) that together have painted a picture of early vertebrate development in the past sixty years. Thus, coquí offer an important comparison for understanding the evolution of early vertebrate, and especially amphibian, development. Coquí also represents a natural ‘experiment’ in understanding how alterations of early molecular patterning impact later development.
The changes in early development and molecular organization of the coquí egg have prompted comparisons to the evolution of the amniote egg. The expansion of the vegetal region and shifting of the germ layers towards the animal pole is thought to also have occurred at the evolutionary transition from a membranous egg to the large amniote egg, a hypothesis supported by molecular data (Arendt and Nübler-Jung, 1999; Buchholz et al., 2007). Additionally, the covering of the yolk in E. coqui by dorsal structures, including muscles and skin, is a characteristic that may have been important in the evolution of amniotes (Elinson and Fang, 1998).
A final parallel between E. coqui and amniote development involves the nutritional endoderm. In the typical 1–2 mm amphibian egg, the whole egg becomes an embryo. In contrast, the nutritional endoderm is a novel coquí tissue made of cells that do not contribute to the embryonic intestine (as does regular endoderm) but do provide nutrition (Buchholz et al., 2007). This tissue has been proposed as an intermediate step in the evolution of the large amniote egg, as nutritional endoderm is like extraembryonic tissues in amniotes in that some oocyte material is used purely for nutrition and does not contribute to the embryo (Elinson, 2009). Therefore, coquí acts as a “missing link” or “transitional” model system to understand a possible evolutionary path between membranous eggs and the amniote egg.
Late development
Post-embryonic development (metamorphosis) is shifted prior to hatching in coquí (into the period we call embryogenesis in coquí). Because coquí skip the tadpole stage, there are two potential trajectories of embryonic development: (1) larval structures never form, and adult features form directly, or (2) larval features develop and are remodeled into the adult morphology prior to hatching. Both of these cases are found for different structures in E. coqui.
Limbs are perhaps the most dramatic adult feature to form directly and early in coquí relative to metamorphosing frogs. Although the basic sequence and pattern of limb bone formation is conserved between E. coqui and metamorphosing frogs (Hanken et al., 2001), expression patterns of limb development genes differ, or are completely absent (Gross et al., 2011; Kerney and Hanken, 2008). Another major difference in E. coqui limb development is the absence of physical ridge of ectoderm (the AER, or apical ectodermal ridge; Richardson et al., 1998) that promotes and maintains growth of the limb in all frogs and all other vertebrate models examined, with the exception of salamanders (Hanken, 1986; Saunders, 1998; Saunders, 1948; Tarin and Sturdee, 1971). Although the physical structure is lacking in E. coqui, gene expression and transplantation studies suggest that major signaling centers are still present and function in coquí limbs as they do in other vertebrates (Gross et al., 2011; Hanken et al., 2001).
Several other adult features appear directly in the adult configuration. During metamorphosis, adult muscle must be recruited from satellite cell populations, whereas in E. coqui the adult muscle fibers appear directly (Hanken et al., 1997). Adult nervous system features important for terrestrial life also form directly, including the olfactory system (Jermakowicz et al., 2004), the retina, and the optic tectum (Schlosser and Roth, 1997). In tadpoles, neurogenesis and proliferation of the spinal cord increase dramatically after hatching. These developmental events occur in the coquí spinal cord earlier relative to other parts of the central nervous system, likely because they are linked to early limb development (Schlosser, 2003).
Some larval features form briefly, and then are remodeled in the final third of development, which has therefore been nicknamed “cryptic” metamorphosis (Callery and Elinson, 2000). Many tadpole-specific cranial cartilages and muscles are patterned, or outlined, by gene expression, but the tissues never actually form (Kerney et al., 2010). The lower jaw and parts of the skeleton that would support the gills appear in the mid-metamorphic arrangement (Hanken et al., 1992; Ziermann and Diogo, 2014). Interestingly, some ancestral muscles important for aquatic feeding of the tadpole form appear briefly, although these muscles have no function in E. coqui (Hanken et al., 1997).
Finally, it is worth noting features of E. coqui development that are entirely novel and the typical tadpole-specific features that never form. The E. coqui tail is highly vascularized and may be used as a respiratory organ, a hypothesis supported by the observation that embryos undergoing accelerated development, including accelerated tail resorption, die if they cannot access air (ML, personal observation). The cement gland, which secretes a sticky mucus and allows tadpoles to secure themselves to substrate, and the lateral line, sensory cells that detect water movement and vibrations, never form (Schlosser et al., 1999). Altogether these observations inform our understanding of developmental modularity and constraint.
Endocrine regulation of development
Given their unique life history, E. coqui are also useful for understanding the evolution of endocrine regulation of development. Thyroid hormone (TH) controls the timing of post-embryonic metamorphosis. Events normally under TH control in metamorphosing frogs appear to be a mixture of TH-independent and TH-dependent in E. coqui. For example, tail resorption and aspects of metabolism are regulated by TH as in metamorphing frogs (Callery and Elinson, 2000; Elinson, 1994), but limb development and growth, dependent on TH in metamorphosing frogs, seems to have both an early TH-independent and a late TH-dependent period in coquí (Callery and Elinson, 2000; Elinson, 1994). The hypothesis that maternally derived TH (Elinson, 2013; Laslo et al., 2019) could influence the early TH-independent period has not yet been directly tested. Other aspects of endocrine regulation of development appear to be conserved in E. coqui and metamorphosing frogs, including interactions of TH and glucocorticoids, and the secretion of pituitary hormones by corticotropin-releasing hormone (Jennings et al., 2015; Kulkarni et al., 2010).
E. coqui’s direct-developing life history continues to teach us about the evolution of development. Coquí have taught us how egg size impacts early development, how the amniote egg may have evolved, how the vertebrate limb development module evolves, and how endocrine regulation of development evolves. These frogs also represent an exciting model for hormonal contributions to development. Thyroid hormone is necessary for neural development in vertebrates; however, this is difficult to study in mammals because thyroid hormones cross the placenta.
Coquí share many of the traits that make Xenopus species good models for translational research, including large and easily manipulated eggs; however, because coquí lack the extended free-swimming larval period of Xenopus, coquí’s life cycle is more similar to the human development than Xenopus’. Additionally, evolutionary life cycle transitions across the animal tree of life (in fish, echinoderms, and insects) and within frogs can benefit from understanding the mechanism of direct development in coquí. Specifically, coquí is a valuable starting point for studies on the convergent evolution of direct development in frogs. Finally, previous studies have relied on a candidate gene approach. In the future, our understanding of development in E. coqui stands to benefit from modern sequencing technologies that allow an unbiased, exploratory approach to developmental questions. Because direct development is an evolutionary shift in developmental timing, and metamorphosis is relatively well understood, coquí represents an ideal model to investigate the proximate mechanisms, including the role of maternal hormones, in these shifts in developmental timing (Box 2).
Outstanding questions about the natural history of Eleutherodactylus coqui
Developmental biology
How does maternal nutritional and hormonal contribution impact early development and developmental timing (e.g., through yolk hormones)?
How does heterochrony evolve and what are its genomic mechanisms (e.g., evolution of regulatory sequences or chromatin structure)?
Do mechanisms mediating developmental plasticity in metamorphosing frogs also influence (plasticity in) developmental timing in coquí (e.g., glucocorticoids, thyroid hormone)?
How does the mechanism of direct development in E. coqui compare to other direct-developing frogs?
Neuroethology and social behavior
What behavioral, neural, and molecular mechanisms govern (switching between) distinct call types (aggressive vs courtship vs defense) in males (e.g., are distinct neural activity and/or hormonal patterns associated with distinct call types)?
How are trade-offs between alternative behavioral states (territorial vs parental) negotiated at behavioral, physiological, and neural levels (e.g., do expression changes in shared or distinct gene sets mediate behavioral switching)?
What are the neural and genomic molecular mechanisms mediating female mate choice of choice (e.g., what neural and hormonal changes are triggered by attractive male calls)?
Conservation/invasion biology
How have invasive populations adapted to new environments despite low genetic divergence (e.g., in antipredator behavior or physiology)?
How can ongoing introductions and new populations be used to track rapid adaptation to novel environments (e.g., expansions to high elevation sites in Hawai’i)?
Have coquí adapted physiologically and/or behaviorally following eradication efforts (e.g., increased resistance to citric acid)?
Integrative questions
Proximate mechanisms of invasion
Are there genomic signatures of invasiveness? How does local adaptation take place in the face of low genetic diversity?
How do natural history traits like developmental mode and communication play facilitate invasion and in turn evolve in response to invasion?
How does the behavior of an invasive species contribute to ecosystem remodeling?
What are the genetic and molecular mechanisms of Bd resistance in coquí and are they advantageous in the face of novel pathogens?
Parent-offspring interactions
What are the impacts of early life development and parental hormonal and nutritional contributions on later behavior in E. coqui?
What are the maternal contributions to offspring in absence of direct parental care by females?
What is the role of signaling (chemical or hormonal) between adults and embryos/juveniles?
Comparative studies across Eleutherodactylids
What can comparative genomic studies tell us about the development of alternative life history strategies?
How do neural mechanisms controlling behavior differ in species with different parental care strategies?
How does plasticity in development and behavior shape differences between species?
Social behavior
Vocal communication
Vocal communication is central to the lives of most frogs. Among many possible choices, researchers were attracted to E. coqui due to their remarkably loud calls and sheer abundance. This initial choice was fortuitous, and studies of vocal communication in E. coqui have provided key insights.
E. coqui have a rich vocal repertoire, adjusting their namesake call for distinct social and environmental contexts. In the context of territory defense, males increase call rate and volume and vary the number of syllables in their call, sometimes using single ‘co’ notes and sometimes appending additional ‘co’ and ‘qui’ syllables (e.g., ‘co-co-qui-qui’, ‘co-qui-qui-qui’, etc.; Narins and Capranica, 1976; Stewart and Rand, 1991; see Video 1). Vocal escalation progresses to physical attack if an intruder comes within less 50cm (Reyes-Campos, 1971). By contrast, during courtship males reduce call volume and occasionally drop the ‘qui’ syllable (Townsend and Stewart, 1986; see below for additional details of courtship behavior).
Coquí are notorious in Puerto Rico for their loud vocalizations at night.
Video credit: K Harmon.
Unlike in most frogs, female E. coqui also call. Female calls consist of one long note followed by a series of shorter notes that are of a distinct quality and quieter than male calls (Stewart and Rand, 1991). Females call to defend their daytime retreats and will physically attack intruders of both sexes (Stewart and Rand, 1991). In the wild coquí of any sex are found together in retreats only during courtship, and aggressive calls in both sexes likely allow frogs to appropriately space themselves and avoid costly physical encounters (Stewart and Bishop, 1994).
In addition to varying call volume, rate, and syllable number, the ‘co’ and ‘qui’ syllables themselves have distinct functions: the ‘co’ syllable is specialized for aggressive interactions while the ‘qui’ syllable is important for mate attraction (Narins and Capranica, 1976; Narins and Capranica, 1978). These functional specializations are associated with differences in auditory sensitivity between sexes (Narins and Capranica, 1976), a finding that was the first demonstration of sex differences in peripheral auditory tuning in a vertebrate.
Taken together these observations are exciting as they provide a mechanism by which males may simultaneously communicate specialized information to male competitors and female mates, even in a noisy, complex sound environment. More recent studies further highlight coquí as an intriguing example of how selection may fine-tune multi-functional communication systems by demonstrating behavioral and auditory tuning along an altitudinal gradient in E. coqui (Meenderink et al., 2017; Meenderink et al., 2010; O’Neill and Beard, 2011).
In brief, the complex interplay of note identity, loudness, and sequence alongside auditory tuning and behavioral adaptations remain fertile ground for investigation of complex, multi-functional vocal communication systems in E. coqui. Excitingly, genomic technologies for quantification and manipulation can now be brought to bear on open questions concerning the mechanisms of both signal production and reception.
Courtship
Courtship in E. coqui is lengthy and involves a unique amplexus posture. Once a prospective mate arrives, the male leads the female to a nest site in his territory, moving 10-30cm ahead of the female and calling to encourage her to follow (Townsend and Stewart, 1986). This lead-and-follow continues until the pair reaches and enters a nest site. Once inside, the female inspects the site and – if she accepts – backs herself underneath the male (Townsend, 1989; Townsend and Stewart, 1986).
Unlike many amphibians, males do not clasp females. Instead, a female will lay her legs over the top of the male’s legs in a “reverse hind leg clasp” (Townsend and Stewart, 1986). This unique posture is thought to facilitate internal fertilization, another coquí trait rare among frogs, with only 10 anuran species (0.13%) known to have internal fertilization (Sever et al., 2003).
Over the course of oviposition, the female moves out from under the male, such that he comes to rest on the newly laid clutch. While courtship generally commences in the early evening (peak calling in males is between dusk and midnight; Woolbright, 1985), oviposition does not occur until the next morning (Townsend and Stewart, 1986). Both the male and female generally remain in the nest until dusk of the following day (i.e. nearly 24 hours after courtship is initiated), at which time the male becomes aggressive, calling and biting to chase the female out of the nest. Data suggest that extended courtship is required for females to complete ovulation prior to oviposition (Townsend and Stewart, 1986). This research establishes great potential for studying connections between behavior and physiology in coquí.
Parental care
Parental care is exhibited by only ~10% of anurans (Wells, 2007), making both the parental care and the direct development from embryo to froglet in E. coqui notable. Indeed, these unique aspects of coquí’s life history may be linked because they eliminate the need for embryos and tadpoles to be in water. Reduced reliance on water for reproduction is favored because it reduces predation risk to embryos and juveniles and allows frogs to take advantage of more varied habitats. Male coquí provide care to their offspring for 17–26 days until embryos hatch, often remaining with hatched froglets for an additional 1–6 days (Townsend et al., 1984). In the wild, males typically provide care to one clutch at a time and seldomly leave the nest during the brooding period (Townsend, 1989; Townsend et al., 1984). Care is critical for offspring survival and clutch failure is ~80% when males are removed due to desiccation, predation, and cannibalism (Townsend et al., 1984).
Parental care is likely energetically costly, both directly and indirectly, due to reduced opportunities for foraging, territory defense, and mating (Townsend, 1989). The role of cannibalism highlights these trade-offs. Coquí males cannibalize the eggs of conspecifics (simultaneous resource acquisition and aggression/competition) who vigorously defend their brood (an additional cost of care; Townsend et al., 1984). However, fathers are also known to cannibalize their own eggs, presumably to recover energetic resources when brood failure is likely due to high egg mortality from extrinsic factors (e.g., fungal infection), depredation, or cannibalism by another coquí (Townsend et al., 1984). As mechanistic links between parental behavior and infanticide have been demonstrated in mice (Wu et al., 2014) and suggested in other species (Fischer and O’Connell, 2017), these observations provide a particularly intriguing starting point for work exploring mechanisms of behavioral trade-offs.
Based on the mating and parental behavior detailed above, E. coqui males can be classified as being in a territorial versus a parental state. These behavioral states are largely mutually exclusive, begging the question of how alternative states are regulated. An early study showed that, as in many vertebrates, testosterone decreases during parental effort in E. coqui (Townsend and Moger, 1987).
More recent work has uncovered additional mechanisms mediating aggressive behavior, including a role for the nonapeptides arginine vasotocin and mesotocin (the amphibian homologs of mammalian vasopressin and oxytocin, respectively), as well as serotonin. Increased vasotocin or oxytocin increased aggressive calling behavior and the propensity for non-territorial satellite males to establish a new territory (Ten Eyck and Ten Eyck, 2020; Ten Eyck and Ten Eyck, 2017; Ten Eyck and ul Haq, 2012), while increased serotonin signaling decreased calling in male territory holders (Ten Eyck, 2008; Ten Eyck and Ten Eyck, 2020).
Interestingly, vasotocin treatment did not increase calling in parental (non-calling, non-territorial) males, suggesting that the effects of vasotocin treatment are modulated by parental state (Ten Eyck and ul Haq, 2012). Nonapeptides are broadly implicated in vertebrate sociality including parental care and aggression (Goodson, 2013; Goodson, 2008), and E. coqui provide opportunities to understand how these molecules mediate social behavioral trade-offs not only among species but also at the individual level. Given early indications that parental state influences the effects of neuromodulators on calling behavior, it will be interesting to explore the effects of these molecules on the different call types discussed above, which are produced for distinct purposes in different behavioral contexts.
In sum, coquí social behavior provides opportunities for exploring sensory processing, behavioral trade-offs, and how and why unique life histories evolve. Existing studies address various aspects of these questions and set the stage for integration across behavioral contexts and levels of analysis. For example, previous studies have characterized differences in auditory tuning in males versus females (Narins and Capranica, 1976) and the molecular correlates of calling in males (Ten Eyck, 2008; Ten Eyck and ul Haq, 2012), and it would now be fruitful to disentangle neural mechanisms of distinct call types, to explore neural and molecular mechanisms of vocalization in females, and to ask whether the same neuromodulators that influence calling behavior also modulate auditory sensitivity within and between sexes (see Box 2).
Biological invasion
While coquí are a beloved cultural symbol in their native Puerto Rico, they are much less celebrated – and even despised – where they have invaded in Central America, North America, and the Caribbean (Figure 3). With the notable exception of cane toads, Rhinella marina, in Australia (see Shine, 2010 for review), invasive amphibians are relatively rare. The generalist lifestyle of E. coqui and their independence from standing water for reproduction have contributed to coquí’s rapid dispersal and proliferation, as have both accidental and intentional human transport (Everman et al., 2013; Kraus and Campbell III, 2002).
E. coqui are considered among the 100 worst invasive species in the world (Simberloff and Rejmanek, 2019), bringing with them a host of biological and economic problems. The Hawaiian invasion is both the most extensive and the best studied. Hawaiian populations reach densities of up to 91,000 frogs/hectare (Beard et al., 2009), two to three times higher than estimates for native populations in Puerto Rico (Woolbright et al., 2006), and among the highest known for any amphibian worldwide. From a biological perspective, the invasion of coquí presents several concerns, most notably competition with and predation on endemic species (Beard, 2007; Choi and Beard, 2011). From an economic perspective, these small frogs are responsible for millions of dollars of damage each year (Kaiser and Burnett, 2006), decreasing property values (chorusing coquí produce sound pressures of up to 95 dB – similar to a motorcycle engine) and impacting multi-million-dollar floriculture industry (Beard and Pitt, 2005). Nonetheless, having coquí on their property decreases residents’ negative perceptions (Kalnicky et al., 2014) suggesting attitudes toward these noisy invaders may shift over time.
In view of biological and economic concerns, substantial resources have been invested in eradicating E. coqui in Hawai’i (reviewed Beard and Pitt, 2012). While eradication is likely no longer possible on the Big Island, smaller populations on O’ahu and Kauai were successfully eradicated (Beachy et al., 2011), although reintroductions remain a constant concern. Hot water treatment is effective in greenhouse settings and treatment with citric acid is approved by the USDA. The chytrid fungus Batrachochytrium dendrobatidis (Bd) was proposed as a biological control agent, but this idea has been largely abandoned as coquí are somewhat resistant to the fungus (Langhammer et al., 2014; Rollins-Smith et al., 2015) and due to concerns that Bd could spread to vulnerable amphibians outside Hawai’i (Beard and O’Neill, 2005). Notably, the above methods are possible in Hawai’i because the archipelago does not have any native amphibians, but are unlikely to translate well to other coquí invasions – for example in Costa Rica – where protection of endemic species is of central concern.
While the invasion of coquí presents serious biological and economic concerns, it also presents opportunities for basic and applied research (see Hanson et al., 2020 for a similar example in house sparrows, and the excellent body of work on cane toad invasion in Australia; e.g., Brown et al., 2015; DeVore et al., 2021; Rollins et al., 2015; Selechnik et al., 2017). Conservation-centered questions abound and excellent research is being conducted in this area (Beard et al., 2009; Beard and Pitt, 2005; Joglar, 1998). Additionally, comparisons between invasive and native Puerto Rican populations provide a largely untapped opportunity for exploration of local adaptation and examination of genomic and phenotypic traits that facilitate invasion. This research could shed light on why E. coqui have been so successful – both in their native and introduced ranges – while some closely related species are imperiled. E. coqui’s relative resistance to Bd (Langhammer et al., 2014; Rollins-Smith et al., 2015), which is driving many amphibian species to extinction, may provide particularly important insights. Finally, large, invasive populations allow for developmental and neurobiological studies that often require destructive sampling and would therefore not be ethical in most wild populations. Alongside explicit consideration of their natural history, study of model organisms outside the lab has the potential to provide new insights through the incorporation of critical environmental and ecological context.
Conclusion
Thanks to their unique natural history, coquí have attracted the interest of diverse research fields, including some outside the scope of this paper (Box 1).
Direct development from egg to froglet has made coquí an excellent model system for evolutionary developmental biologists to examine how changes in maternal provisioning influence development and speculate on the evolution of the amniotic egg. Studies of coquí development have uncovered new evolutionary features and probed how coquí have altered conserved developmental processes in the transition from a biphasic life history to direct development. In turn, direct development has facilitated the coquí’s invasion potential, making these frogs a focus of conservation research. Through direct development and parental care, coquí have reduced reliance on water which opens many ecological niches in comparison to water-bound amphibians and facilitated the invasion of coquí on many tropical islands. Coquí’s impressively loud nocturnal choruses, in and outside their native range, have also attracted researchers in behavioral neuroscience, yielding work that contributes to our understanding of auditory communication and adaptations for sensory processing.
Despite this rich history of research, there are still unexplored aspects of coquí natural history that are driving novel research. For instance, coquí have recently shown promise in advancing bioengineering due to incredibly extensibile gular skin tissue that allows them to produce their characteristic vocalizations (Hui et al., 2020; Box 1).
Despite great interest in the natural history of coquí across diverse fields, there is little work bridging disparate disciplines. The time is ripe to leverage previous studies and emerging technologies to build a holistic understanding of this culturally, developmentally, behaviorally, and evolutionarily interesting species. In addition to discipline-spanning work, coquí are poised for integrative research spanning levels of biological organization, from molecular mechanisms to ecosystem impacts. Recent and foundational studies provide the necessary framework for impactful application of genomic, transcriptomic, and genetic manipulation techniques: transcriptomic approaches have already been successfully applied (Laslo, 2019), a genome is currently under construction (Vert Genome Project, https://vertebrategenomesproject.org/), and tools for genomic manipulation are particularly amenable in amphibians, which have large, externally developing embryos.
The intersection of traditionally distinct conservation, developmental, and neuroethological work provides fertile ground for impactful integrative studies; for example, linking developmental manipulations with adult behavioral outcomes, understanding the evolution and development of alternative life history strategies, understanding the impacts of maternal hormonal and nutritional contributions to offspring health and developmental timing in the absence of direct care, and exploring the role of developmental mode and communication in invasion potential, to name just a few (see Box 2). Moreover, diversity across closely related Eleutherodactylids provides opportunities to expand these questions into a powerful comparative framework (Jourjine and Hoekstra, 2021; see Box 3).
Natural diversity in Eleutherodactylids
Eleutherodactylus is a highly speciose genus ubiquitous across Neotropical regions (Crawford and Smith, 2005). Estimates for the number of species included in the genus Eleutherodactylus vary widely, from 185 to over 700; at the time of writing an AmphibiaWeb search for “Eleutherodactylus” returned 202 hits (AmphibiaWeb, 2021; Duellman, 1993; Hedges et al., 2008). While there was a high rate of newly described or resurrected taxa of Eleutherodactylids in the late 1990s and early 2000s (e.g., Campbell and Savage, 2000; Duellman and Pramuk, 1999; Lynch, 2001; Savage and Myers, 2002), further phylogenetic studies are needed to evaluate taxonomic relationships and biogeography of this diverse genus (Crawford and Smith, 2005; Hedges et al., 2008).
The life history diversity of Eleutherodactylids makes this genus a prime candidate for comparative studies and advancement as a powerful ‘model clade' for integrative research (Jourjine and Hoekstra, 2021). Such work is embodied by research on Peromyscus mice (see Bedford and Hoekstra, 2015) and has potential in models like coquí and ricefish (see Hilgers and Schwarzer, 2019). For example, among Eleutherodactylus species there is broad variation in parental care strategies with species exhibiting uniparental care of eggs and/or froglets or no care (Townsend, 1996; Figure 4B). However, the sex providing uniparental care differs across species (Townsend, 1996; Figure 4B). E. cooki and E. coqui both show paternal care of eggs, whereas E. cundalli shows maternal care, and E. johnstonei shows amphisexual parental care of eggs (either paternal or maternal but not biparental; Bourne, 1998; Burrowes, 2000, p. 200; Townsend, 1996). Historically, E. planirostris was classified as exhibiting no parental care (Townsend, 1996) but recent observations suggest this species possibly has paternal care (Iturriaga and Dugo-Cota, 2018). Notably, E. cundalli mothers transport hatched froglets ‘piggy-back’ style (Diesel et al., 1995), as compared to the more common transportation of tadpoles (Weygoldt, 2009). Natural variation in Eleutherodactylidae provides an opportunity to understand the similarities and differences of these unique but analogous forms of parental care. In addition, most Eleutherodactylids are oviparous, but one species (E. jasperi) is ovoviviparous (Drewry and Jones, 1976), which opens the potential to investigate questions of development and maternal investment between closely related species. Finally, there is remarkable diversity in auditory tuning in this group (Lewis et al., 1992) providing fertile ground for comparative studies of multi-modal communication. The broad foundational knowledge about E. coqui provides an excellent starting point for integrative, comparative work.
Across Eleutherodactylidae, species vary in their International Union for Conservation of Nature (IUCN) Red List status. Unfortunately, like many amphibian genera (Alford and Richards, 1999), threats such as Bd and habitat destruction have decreased the population of most Eleutherodactylid species and many are considered endangered or critically endangered (IUCN, 2021). In contrast, E. coqui is one of only three known Eleutherodactylids that are increasing in population size (IUCN, 2021). The success of coquí as an invasive species may provide insights into why some amphibian species are thriving while others are struggling to maintain reproductive populations. Scientists across the Caribbean are working hard to conserve the biodiversity of this unique clade of frogs. For example, the non-profit organization El Proyecto Coquí (http://www.proyectocoqui.com/), led by Dr. Rafael L. Joglar at University of Puerto Rico at Ponce, works on conservation of amphibians and reptiles in Puerto Rico, with a particular focus on Eleutherodactylids, which, including the Puerto Rican coquí, are all often commonly referred to as coquí. Anyone interested in contributing to the conservation efforts of Puerto Rico can ‘adopt’ one of these beloved frogs through Conservación ConCiencia’s ‘Adopt-A-Coquí’ program (https://www.conservacionconciencia.org/adopt-a-coqui).
Beyond the science, E. coqui are valuable and unique in their cultural importance. The cultural interest in this small, vocal frog provides an opportunity to engage diverse communities in STEM educational opportunities and citizen science efforts. Coquí are also well-positioned to welcome and include diverse stakeholders in scientific practice.
Given the geographic range of coquí, research involving these frogs can work towards the decolonization of scientific practice by partnering with local researchers and community members, particularly in Puerto Rico and Hawai’i. These partnerships are mutually beneficial as local scientific and lay communities have a unique knowledge of and relationship with their local flora and fauna but often lack the infrastructure and training opportunities that promote certain subdisciplines (for example, ready access to expensive, rapidly evolving genomic technologies).
Meaningful collaboration can improve the science by increasing diversity of experience and thought, and help increase opportunities where they are lacking. Collaborating with local scientists on coquí research in its native and invasive range will help increase the representation of Puerto Rican and other Latinx countries in ecology, neuroscience, and conservation. Coquí provide an opportunity for Puerto Rican scientists to do high-quality work close to home and in fields historically lacking Latinx representation (Lewis et al., 2009; O’Brien et al., 2020; Quirk, 2017; Rincón and Rodriguez, 2020). It is difficult to quantify the representation of Native Hawaiian and Pacific Islanders in STEM because Pacific Islanders are traditionally grouped together with Asian Americans, despite being a group with multiple racial and ethnic identities (Maramba, 2013). Participation is likely lower than Latinx (Levine, 2013; Maramba, 2013; NSF and NCSES, 2021), and the invasion of coquí in Hawai’i provides an opportunity for community engagement and for training Hawaiian scientists in their homelands on research questions with direct local application.
We hope that the broad base of scientific and cultural knowledge about the unique life history of E. coqui will inspire researchers to capitalize on the big potential of these small frogs to do integrative work in the lab, the field, and the community.
Data availability
No new data were generated for this article. Data from Furness and Capellini (2019) and from Pyron (2014; deposited in Dryad: https://doi.org/10.5061/dryad.jm453) were used.
-
Dryad Digital RepositoryData from: Biogeographic analysis reveals ancient continental vicariance and recent oceanic dispersal in amphibians.https://doi.org/10.5061/dryad.jm453
References
-
Global amphibian declines: a problem in applied ecologyAnnual Review of Ecology and Systematics 30:133–165.https://doi.org/10.1146/annurev.ecolsys.30.1.133
-
BookSuccessful control of an incipient invasive amphibian: Eleutherodactylus coqui on O’ahu, Hawai’iIn: Veitch CR, Clout MN, Towns DR, editors. Island Invasives: Eradication and Management. IUCN. pp. 140–147.
-
Potential consequences of the coqui frog invasion in HawaiiDiversity and Distributions 11:427–433.https://doi.org/10.1111/j.1366-9516.2005.00178.x
-
Population density estimates and growth rates of Eleutherodactylus coqui in HawaiiJournal of Herpetology 42:626.https://doi.org/10.1670/07-314R1.1
-
BookEleutherodactylus coqui Thomas (Caribbean tree frog)In: Francis RA, editors. Handbook of Global Freshwater Invasive Species. London: Earthscan. pp. 1–10.
-
Stress and immunity at the invasion front: a comparison across cane toad (Rhinella marina) populationsBiological Journal of the Linnean Society 116:748–760.https://doi.org/10.1111/bij.12623
-
Nutritional endoderm in a direct developing frog: a potential parallel to the evolution of the amniote eggDevelopmental Dynamics 236:1259–1272.https://doi.org/10.1002/dvdy.21153
-
Parental care and sexual selection in the Puerto Rican cave-dwelling frog, Eleutherodactylus cookiHerpetologica pp. 375–386.
-
Coqui frog invasions change invertebrate communities in HawaiiBiological Invasions 14:939–948.https://doi.org/10.1007/s10530-011-0127-3
-
Frogs of the genus Eleutherodactylus (Anura: Leptodactylidae) in the Andes of northern PeruScientific Papers, Natural History Museum, University of Kansas 13:1–78.https://doi.org/10.5962/bhl.title.16169
-
Hearing through the lungs: lung-eardrum transmission of sound in the frog Eleutherodactylus coquiDie Naturwissenschaften 77:192–194.https://doi.org/10.1007/BF01131168
-
A practical guide to the developmental biology of terrestrial-breeding frogsThe Biological Bulletin 179:163–177.https://doi.org/10.2307/1541765
-
Leg development in a frog without a tadpole (Eleutherodactylus coqui)The Journal of Experimental Zoology 270:202–210.https://doi.org/10.1002/jez.1402700209
-
Nutritional endoderm: a way to breach the holoblastic-meroblastic barrier in tetrapodsJournal of Experimental Zoology. Part B, Molecular and Developmental Evolution 312:526–532.https://doi.org/10.1002/jez.b.21218
-
Metamorphosis in a frog that does not have a tadpoleCurrent Topics in Developmental Biology 103:259–276.https://doi.org/10.1016/B978-0-12-385979-2.00009-5
-
Human-facilitated jump dispersal of a non-native frog species on Hawai’i IslandJournal of Biogeography 40:1961–1970.https://doi.org/10.1111/jbi.12146
-
Modification of feeding circuits in the evolution of social behaviorThe Journal of Experimental Biology 220:92–102.https://doi.org/10.1242/jeb.143859
-
Predation by Giant Crab Spiders on the Puerto Rican frog Eleutherodactylus coquiHerpetologica 37:125–129.
-
The evolution of parental care diversity in amphibiansNature Communications 10:4709.https://doi.org/10.1038/s41467-019-12608-5
-
Phylogenetic analyses reveal unexpected patterns in the evolution of reproductive modes in frogsEvolution; International Journal of Organic Evolution 66:3687–3700.https://doi.org/10.1111/j.1558-5646.2012.01715.x
-
Nonapeptides and the evolutionary patterning of socialityProgress in Brain Research 170:3–15.https://doi.org/10.1016/S0079-6123(08)00401-9
-
Deconstructing sociality, social evolution and relevant nonapeptide functionsPsychoneuroendocrinology 38:465–478.https://doi.org/10.1016/j.psyneuen.2012.12.005
-
Molecular anatomy of the developing limb in the coquí frog, Eleutherodactylus coquiEvolution & Development 13:415–426.https://doi.org/10.1111/j.1525-142X.2011.00500.x
-
BookDevelopmental Evidence for Amphibian OriginsIn: Hecht MK, Wallace B, Prance GT, editors. Evolutionary Biology. Boston, MA: Springer. pp. 389–417.https://doi.org/10.1007/978-1-4615-6983-1_7
-
Jaw muscle development as evidence for embryonic repatterning in direct-developing frogsProceedings. Biological Sciences 264:1349–1354.https://doi.org/10.1098/rspb.1997.0187
-
Limb development in a “nonmodel” vertebrate, the direct-developing frog Eleutherodactylus coquiThe Journal of Experimental Zoology 291:375–388.https://doi.org/10.1002/jez.1136
-
Tree of life reveals clock-like speciation and diversificationMolecular Biology and Evolution 32:835–845.https://doi.org/10.1093/molbev/msv037
-
The specific molecular composition and structural arrangement of Eleutherodactylus coqui gular skin tissue provide its high mechanical complianceInternational Journal of Molecular Sciences 21:E5593.https://doi.org/10.3390/ijms21165593
-
Parental care in the Greenhouse frog Eleutherodactylus planirostris (Cope, 1862) from CubaHerpetol Notes 11:857–861.
-
BookLos Coquíes de Puerto Rico: Su Historia Natural y ConservaciónSan Juan, Puerto Rico: Editorial de la Universidad de Puerto Rico.
-
Economic impacts of E. coqui frogs in HawaiiInterdisciplinary Environmental Review 8:1.https://doi.org/10.1504/IER.2006.053951
-
Corticotropin-releasing factor regulates the development in the direct developing frog, Eleutherodactylus coquiGeneral and Comparative Endocrinology 169:225–230.https://doi.org/10.1016/j.ygcen.2010.09.009
-
BookEducation in Pacific Island States: Reflections on the Failure of “Grand RemediesHonolulu, HI: East-West Center.
-
Diversity of form in the amphibian papilla of Puerto Rican frogsJournal of Comparative Physiology. A, Sensory, Neural, and Behavioral Physiology 171:421–435.https://doi.org/10.1007/BF00194575
-
Rethinking trends in minority participation in the sciencesScience Education 93:961–977.https://doi.org/10.1002/sce.20338
-
Three new rainfrogs of the Eleutherodactylus diastema group from Colombia and PanamaRevista de La Academia Colombiana de Ciencias Exactas, Físicas y Naturales 25:287–299.
-
Community Colleges and STEMCreating Successful Pathways for Asian Americans and Pacific Islander Community College Students (AAPIs) in STEM, Community Colleges and STEM, Routledge.
-
Communicative significance of the two-note call of the treefrog Eleutherodactylus coquiJournal of Comparative Physiology A 127:1–9.https://doi.org/10.1007/BF00611921
-
Mesoderm formation in Eleutherodactylus coqui: body patterning in a frog with a large eggDevelopmental Biology 236:109–123.https://doi.org/10.1006/dbio.2001.0310
-
WebsiteWomen, Minorities, and Persons with Disabilities in Science and EngineeringAccessed April 29, 2021.
-
Observaciones sobre la conducta del coquí, Eleutherodactylus coqui caribbJ Sci 11:209–210.
-
Limb development and evolution: a frog embryo with no apical ectodermal ridge (AER)Journal of Anatomy 192 ( Pt 3):379–390.https://doi.org/10.1046/j.1469-7580.1998.19230379.x
-
Latinx students charting their own stem pathways: how community cultural wealth informs their stem identitiesJournal of Hispanic Higher Education 20:149–163.https://doi.org/10.1177/1538192720968276
-
A genetic perspective on rapid evolution in cane toads (Rhinella marina)Molecular Ecology 24:2264–2276.https://doi.org/10.1111/mec.13184
-
Coqui frogs persist with the deadly chytrid fungus despite a lack of defensive antimicrobial peptidesDiseases of Aquatic Organisms 113:81–83.https://doi.org/10.3354/dao02823
-
The proximo-distal sequence of origin of the parts of the chick wing and the role of the ectodermThe Journal of Experimental Zoology 108:363–403.https://doi.org/10.1002/jez.1401080304
-
Apical ectodermal ridge in retrospectThe Journal of Experimental Zoology 282:669–676.https://doi.org/10.1002/(sici)1097-010x(19981215)282:6<669::aid-jez3>3.0.co;2-d
-
The things they carried: the pathogenic effects of old and new parasites following the intercontinental invasion of the Australian cane toad (Rhinella marina)International Journal for Parasitology. Parasites and Wildlife 6:375–385.https://doi.org/10.1016/j.ijppaw.2016.12.001
-
BookInternal Fertilization in the Anura with Special Reference to Mating and Female Sperm Storage in AscaphusIn: Jamieson BGM, editors. Reproductive Biology and Phylogeny of Anura. Reproductive Biology and Phylogeny. Science Publishers, Inc. pp. 319–341.
-
The ecological impact of invasive cane toads (Bufo marinus) in AustraliaThe Quarterly Review of Biology 85:253–291.https://doi.org/10.1086/655116
-
The Food Web of a Tropical Rain Forest8. Amphibians, The Food Web of a Tropical Rain Forest, University of Chicago Press.
-
Early limb development of Xenopus laevisJournal of Embryology and Experimental Morphology 26:169–179.
-
Serotonin modulates vocalizations and territorial behavior in an amphibianBehavioural Brain Research 193:144–147.https://doi.org/10.1016/j.bbr.2008.05.001
-
Mammalian nonapeptides activate territorial behavior in an amphibianPhysiology & Behavior 179:220–225.https://doi.org/10.1016/j.physbeh.2017.06.020
-
Serotonin and vasotocin function in territorialityPharmacology, Biochemistry, and Behavior 199:173068.https://doi.org/10.1016/j.pbb.2020.173068
-
The costs of male parental care and its evolution in a neotropical frogBehav Ecol Sociobiol 19:187–195.
-
Courtship and mating behavior of a Puerto Rican frog, Eleutherodactylus coquiHerpetologica 42:165–170.
-
The consequences of microhabitat choice for male reproductive success in a tropical frog. The consequences of microhabitat choice for male reproductive success in a tropical frog (Eleutherodactylus coqui)Herpetologica 45:451–458.
-
BookPatterns of Parental Care in Frogs of the Genus Eleutherodactylus.In: Powell R, Henderson RW, editors. Contributions to West Indian Herpetology: A Tribute to Albert Schwartz. Society for the Study of Amphibians and Reptiles. pp. 229–239.
-
Parental care and the evolution of terrestriality in frogsProceedings. Biological Sciences 286:20182737.https://doi.org/10.1098/rspb.2018.2737
-
BookThe Ecology and Behavior of AmphibiansChicago: The University of Chicago Press.https://doi.org/10.7208/chicago/9780226893334.001.0001
-
Evolution of parental care in dart poison frogs (Amphibia: Anura: Dendrobatidae)Journal of Zoological Systematics and Evolutionary Research 25:51–67.https://doi.org/10.1111/j.1439-0469.1987.tb00913.x
-
BookThe ecological impact of man on the south Florida herpetofaunaLawrence, Kansas: University of Kansas.
-
Patterns of nocturnal movement and calling by the tropical frog Eleutherodactylus coquiHerpetologica 41:1–9.
Decision letter
-
Helena Pérez ValleReviewing Editor; eLife, United Kingdom
-
Peter RodgersSenior Editor; eLife, United Kingdom
-
Ryan KerneyReviewer; Gettysburg College, Gettysburg, United States
In the interests of transparency, eLife publishes the most substantive revision requests and the accompanying author responses.
Decision letter after peer review:
Thank you for submitting your article "The big potential of the small frog Eleutherodactylus coqui " to eLife for consideration as a Feature Article. Your article has been reviewed by 3 peer reviewers, and the evaluation has been overseen by a member of the eLife Features Team (Helena Pérez Valle). The following individual involved in review of your submission has agreed to reveal their identity: Ryan Kerney.
The reviewers and editors have discussed the reviews and we have drafted this decision letter to help you prepare a revised submission.
Summary:
This is a useful synthesis from multiple perspectives on the research surrounding the frog Eleutherodactylus coqui. Like so many focal species in biological research, there are non-overlapping research programs from a range of disciplines that are converging on this fascinating frog. This review will be of interest to a wide range of specialists, since the article provides not only a very broad overview of the current knowledge about the biology and life history of E. coqui, but also points out potential major directions for future research.
Essential revisions:
1. Please update Figure 4 to include more information about the convergence of direct development in amphibians and parental care in Eleutherodactylids. Some families with direct development are missing from the tree labels (e.g. Rhacophoridae) and other clades with direct development on the tree are not labelled (e.g. within the clade that includes Arthroleptidae). See Kentwood Wells (Wells K. 2007. The Ecology and Behavior of Amphibians. Chicago: The University of Chicago Press) to help clarify 4A. Please include (if possible) when direct development or parental types evolved, especially in relation to coquí frogs: e.g. are coquís among the oldest lineages to have direct development and parental care? Or are there other related species that developed it first? Please also provide more information about the paternal/maternal care species named in 4B. Please also point out that coqui offers a basis of comparison for studies on the convergence of direct development, which evolved independently in multiple amphibian lineages. The work by Rick Shine on cane toad physiology and evolution in Australia may provide another interesting basis of comparison to the coqui invasions. See:
– Rollins LA, Richardson MF, Shine R. A genetic perspective on rapid evolution in cane toads (Rhinella marina). Mol Ecol. 2015 May;24(9):2264-76. doi: 10.1111/mec.13184. Epub 2015 Apr 20. PMID: 25894012
– Selechnik D, Rollins LA, Brown GP, Kelehear C, Shine R. The things they carried: The pathogenic effects of old and new parasites following the intercontinental invasion of the Australian cane toad (Rhinella marina). Int J Parasitol Parasites Wildl. 2016 Dec 29;6(3):375-385. doi: 10.1016/j.ijppaw.2016.12.001. PMID: 30951567; PMCID: PMC5715224
– Shine R. The ecological impact of invasive cane toads (Bufo marinus) in Australia. Q Rev Biol. 2010 Sep;85(3):253-91. doi: 10.1086/655116. PMID: 20919631
2. In Figure 3, please confirm that coquís are currently present in Florida, given that they were thought to disappear during a cold winter around the year 2,000. They are also no longer on Kauai and Oahu since they were eradicated, so please remove those from the map.
3. In box 2 please ensure that the questions are specific and testable. For example:
– The question about developmental plasticity in juveniles is vague, please add more details as to what developmental plasticity means in the context of the coquí, and what traits would be studied.
– The question regarding the role of female mate choice seems quite broad, but it is listed under neuroethology and social behaviour. Would it be possible to rephrase the question to frame it more specifically within this heading?
– There is already some research into how native and invasive populations have diverged, as well as some investigation into rapid adaptation in Hawaii. See work by Eric O'Neill: O'Neill, EM, KH Beard, CW Fox. 2018. Body size and life history traits in native and introduced population of Coqui frogs. Copeia; O'Neill, EM, KH Beard, CW Fox. 2012. Cast adrift on an island: introduced populations experience an altered balance between selection and drift. Biology Letters.
– How do the mechanisms of Bd resistance relate to invasion per se?
https://doi.org/10.7554/eLife.73401.sa1Author response
Essential revisions:
1. Please update Figure 4 to include more information about the convergence of direct development in amphibians and parental care in Eleutherodactylids. Some families with direct development are missing from the tree labels (e.g. Rhacophoridae) and other clades with direct development on the tree are not labelled (e.g. within the clade that includes Arthroleptidae). See Kentwood Wells (Wells K. 2007. The Ecology and Behavior of Amphibians. Chicago: The University of Chicago Press) to help clarify 4A.
We have labeled the remaining clades containing direct developing species using data from Furness and Capellini (2019). We also corrected an error in the original figure in the location of Pipidae and have corrected it in the revision.
Please include (if possible) when direct development or parental types evolved, especially in relation to coquí frogs: e.g. are coquís among the oldest lineages to have direct development and parental care? Or are there other related species that developed it first?
We have updated the figure caption for Figure 4 to include details on when direct development in Eleutherodactylids is likely to have evolved relative to other independent occurrences of direct development. Because data are sparse for Eleutherodactylids, we do not know what the ancestral parental care type for Eleutherodactylids is but it is likely to have exhibited some type of parental care given the terrestrial nature and direct development of Eleutherodactylids.
Please also provide more information about the paternal/maternal care species named in 4B.
We have added more details about the labeled Eleutherodactylus species in Box 3. We chose these species to showcase the diversity in parental care strategies in this genus.
Please also point out that coqui offers a basis of comparison for studies on the convergence of direct development, which evolved independently in multiple amphibian lineages.
We have added a sentence describing this point at the end of “Development” section, as well as a question in Box 2.
The work by Rick Shine on cane toad physiology and evolution in Australia may provide another interesting basis of comparison to the coqui invasions. See:
– Rollins LA, Richardson MF, Shine R. A genetic perspective on rapid evolution in cane toads (Rhinella marina). Mol Ecol. 2015 May;24(9):2264-76. doi: 10.1111/mec.13184. Epub 2015 Apr 20. PMID: 25894012
– Selechnik D, Rollins LA, Brown GP, Kelehear C, Shine R. The things they carried: The pathogenic effects of old and new parasites following the intercontinental invasion of the Australian cane toad (Rhinella marina). Int J Parasitol Parasites Wildl. 2016 Dec 29;6(3):375-385. doi: 10.1016/j.ijppaw.2016.12.001. PMID: 30951567; PMCID: PMC5715224
– Shine R. The ecological impact of invasive cane toads (Bufo marinus) in Australia. Q Rev Biol. 2010 Sep;85(3):253-91. doi: 10.1086/655116. PMID: 20919631
We agree and have modified the next to include references to the excellent body of work on the invasion of cane toads in Australia.
2. In Figure 3, please confirm that coquís are currently present in Florida, given that they were thought to disappear during a cold winter around the year 2,000. They are also no longer on Kauai and Oahu since they were eradicated, so please remove those from the map.
We have modified the figure and figure legend to indicate invasive populations in Florida and Hawai’i that have been eradicated.
3. In box 2 please ensure that the questions are specific and testable. For example:
As in other articles in the series, we have intentionally left these questions quite broad as there are many possible avenues for exciting future research. Nonetheless, we have refined our language and included more detailed examples.
– The question about developmental plasticity in juveniles is vague, please add more details as to what developmental plasticity means in the context of the coquí, and what traits would be studied.
Done
– The question regarding the role of female mate choice seems quite broad, but it is listed under neuroethology and social behaviour. Would it be possible to rephrase the question to frame it more specifically within this heading?
As work on call processing has been done we have modified this point to suggest that examining neural and hormonal shifts in response to an attractive call is a promising starting point. As little is known about female choice in this system a wealth of exciting experiments are possible.
– There is already some research into how native and invasive populations have diverged, as well as some investigation into rapid adaptation in Hawaii. See work by Eric O'Neill: O'Neill, EM, KH Beard, CW Fox. 2018. Body size and life history traits in native and introduced population of Coqui frogs. Copeia; O'Neill, EM, KH Beard, CW Fox. 2012. Cast adrift on an island: introduced populations experience an altered balance between selection and drift. Biology Letters.
We agree these studies are interesting and have revised our wording to clarify that we therefore advocate for additional studies in this area.
– How do the mechanisms of Bd resistance relate to invasion per se?
We have moved this point to the ‘Integrative Questions’ as we feel it fits better there.
https://doi.org/10.7554/eLife.73401.sa2Article and author information
Author details
Funding
National Science Foundation (Postdoctoral Fellowship in Biology)
- Sarah E Westrick
Hanse-Wissenschaftskolleg Institute for Advanced Study
- Eva K Fischer
The funders had no role in study design, data collection and interpretation, or the decision to submit the work for publication.
Acknowledgements
We thank members of the Fischer Lab and Mark Hauber for their helpful and encouraging feedback. We also thank Ryan Kerney and two anonymous reviewers for their insights and constructive feedback.
Publication history
- Received:
- Accepted:
- Accepted Manuscript published:
- Version of Record published:
Copyright
© 2022, Westrick et al.
This article is distributed under the terms of the Creative Commons Attribution License, which permits unrestricted use and redistribution provided that the original author and source are credited.
Metrics
-
- 7,075
- views
-
- 340
- downloads
-
- 6
- citations
Views, downloads and citations are aggregated across all versions of this paper published by eLife.
Download links
Downloads (link to download the article as PDF)
Open citations (links to open the citations from this article in various online reference manager services)
Cite this article (links to download the citations from this article in formats compatible with various reference manager tools)
Further reading
-
- Developmental Biology
- Genetics and Genomics
Paternal obesity has been implicated in adult-onset metabolic disease in offspring. However, the molecular mechanisms driving these paternal effects and the developmental processes involved remain poorly understood. One underexplored possibility is the role of paternally induced effects on placenta development and function. To address this, we investigated paternal high-fat diet-induced obesity in relation to sperm histone H3 lysine 4 tri-methylation signatures, the placenta transcriptome, and cellular composition. C57BL6/J male mice were fed either a control or high-fat diet for 10 weeks beginning at 6 weeks of age. Males were timed-mated with control-fed C57BL6/J females to generate pregnancies, followed by collection of sperm, and placentas at embryonic day (E)14.5. Chromatin immunoprecipitation targeting histone H3 lysine 4 tri-methylation (H3K4me3) followed by sequencing (ChIP-seq) was performed on sperm to define obesity-associated changes in enrichment. Paternal obesity corresponded with altered sperm H3K4me3 at promoters of genes involved in metabolism and development. Notably, altered sperm H3K4me3 was also localized at placental enhancers. Bulk RNA-sequencing on placentas revealed paternal obesity-associated sex-specific changes in expression of genes involved in hypoxic processes such as angiogenesis, nutrient transport, and imprinted genes, with a subset of de-regulated genes showing changes in H3K4me3 in sperm at corresponding promoters. Paternal obesity was also linked to impaired placenta development; specifically, a deconvolution analysis revealed altered trophoblast cell lineage specification. These findings implicate paternal obesity effects on placenta development and function as one potential developmental route to offspring metabolic disease.
-
- Developmental Biology
Although the impact of gender-affirming hormone therapy (GAHT) on spermatogenesis in trans women has already been studied, data on its precise effects on the testicular environment is poor. Therefore, this study aimed to characterize, through histological and transcriptomic analysis, the spermatogonial stem cell niche of 106 trans women who underwent standardized GAHT, comprising estrogens and cyproterone acetate. A partial dedifferentiation of Sertoli cells was observed, marked by the co-expression of androgen receptor and anti-Müllerian hormone which mirrors the situation in peripubertal boys. The Leydig cells also exhibited a distribution analogous to peripubertal tissue, accompanied by a reduced insulin-like factor 3 expression. Although most peritubular myoid cells expressed alpha-smooth muscle actin 2, the expression pattern was disturbed. Besides this, fibrosis was particularly evident in the tubular wall and the lumen was collapsing in most participants. A spermatogenic arrest was also observed in all participants. The transcriptomic profile of transgender tissue confirmed a loss of mature characteristics - a partial rejuvenation - of the spermatogonial stem cell niche and, in addition, detected inflammation processes occurring in the samples. The present study shows that GAHT changes the spermatogonial stem cell niche by partially rejuvenating the somatic cells and inducing fibrotic processes. These findings are important to further understand how estrogens and testosterone suppression affect the testis environment, and in the case of orchidectomized testes as medical waste material, their potential use in research.