Host ecology regulates interspecies recombination in bacteria of the genus Campylobacter
Figures
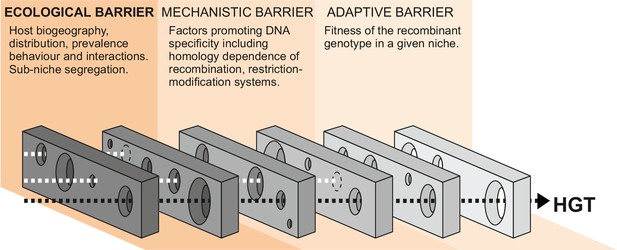
Barriers to horizontal gene transfer (HGT) in bacteria.
A series of barriers must be surmounted for DNA to transmit from one species to another. These are broadly defined in three categories. At a given time, alignment of holes in successive barriers is necessary for HGT to occur. Here, we focus on ecological barriers that are influenced by multiple factors that reflect the physical isolation of bacteria in separate niches.
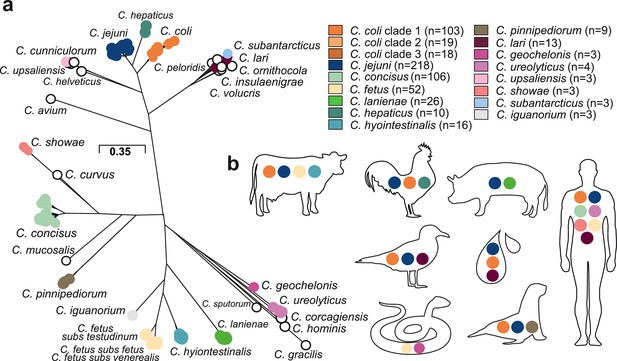
Population structure and host ecology in the genus Campylobacter.
(a) Phylogenetic tree of 631 Campylobacter isolates from 30 species reconstructed using a gene-by-gene concatenated alignment of 820 core genes (shared by >95% of isolates) and an approximation of the maximum-likelihood (ML) algorithm implemented in RAxML. The species name is indicated adjacent to the associated sequence cluster. The scale bar indicates the estimated number of substitutions per site. (b) Isolation source of Campylobacter species with n ≥ 3 isolates.
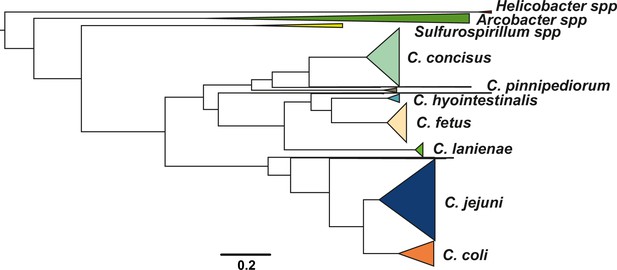
Population structure of the Campylobacteraceae family.
Phylogenetic tree of 506 isolates that belong to the Campylobacteraceae family with Helicobacter pylori used as an outgroup. Different colors correspond to main species with number of isolates greater than three. The tree was reconstructed using a gene-by-gene concatenated alignment of 799 core genes shared by >95% by all isolates and an approximation of the maximum-likelihood (ML) algorithm implemented in RAxML. The scale bar indicates the estimated number of substitutions per site.
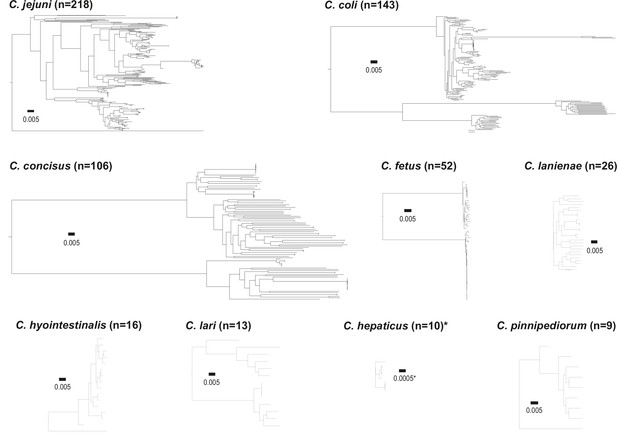
Core genome species trees.
Single-species trees for nine Campylobacter species with >4 isolates demonstrating the diversity for among species. The scale bars indicate the estimated number of substitutions per site. *The scale for the tree corresponding to C. hepaticus is 10 times smaller than the rest.
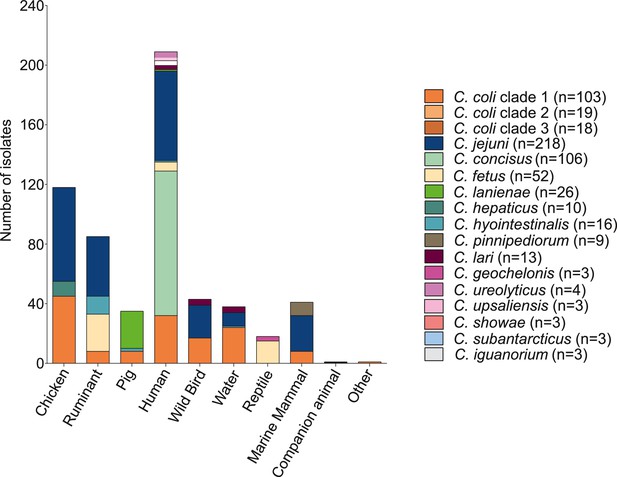
Overview of host associations of Campylobacter species.
Abundance and diversity of 631 Campylobacter isolates in each host and environment. Different colors correspond to main species with number of isolates ≥3. The number of isolates is shown on the y-axis and the various isolation sources are on the x-axis.
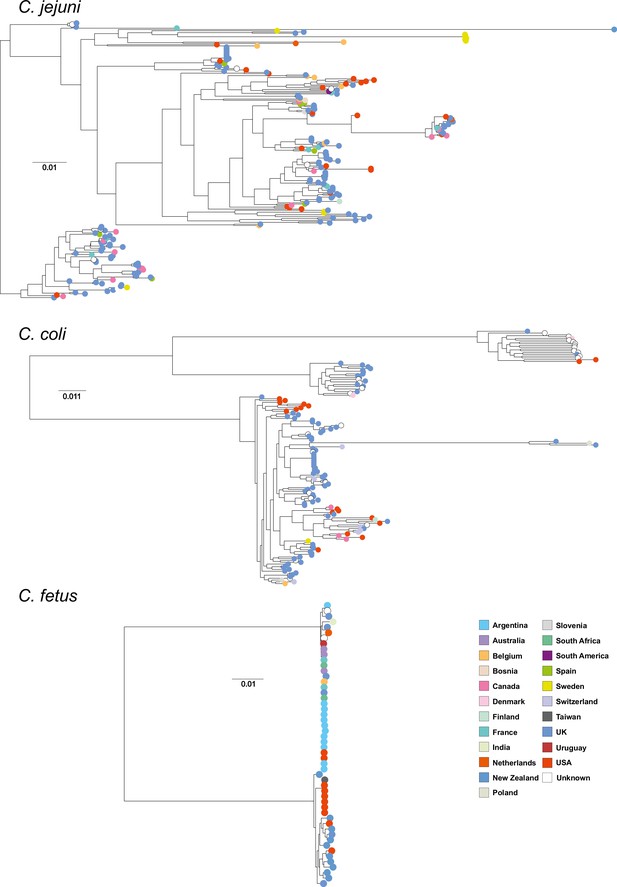
Core genome species trees.
Single-species trees for C. jejuni, C. coli, and C. fetus species that contain isolates from multiple hosts and countries. The scale bars indicate the estimated number of substitutions per site.
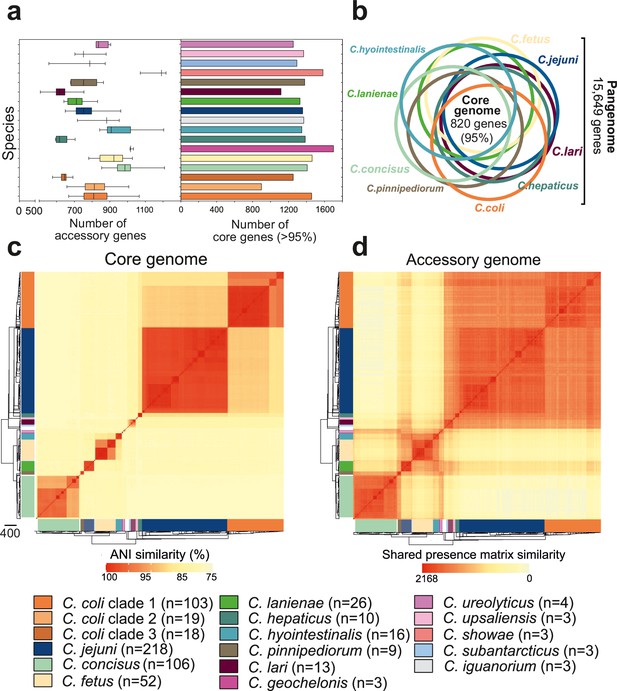
Core and accessory genome variation in the genus Campylobacter.
(a) Overall distribution of the total number of accessory genes (left) and core genes (right) per isolate for each Campylobacter species (where n ≥ 3 isolates). The number of accessory genes is shown as boxplots (min to max). (b) Venn diagram of pangenomes among different Campylobacter species (n ≥ 9). The number of core genes shared by all species is illustrated in the center. (c) Pairwise average nucleotide identity (ANI) comparison calculated for all 631 Campylobacter isolates based upon 820 core genes shared by >95% of isolates. ANI values < 75% are not calculated by FastANI (Jain et al., 2018). (d) Pairwise accessory genome similarity based upon gene presence or absence at 2,168 non-core loci. The heatmap coloring ranges from yellow (minimum) to red (maximum). The matrices are ordered according to the phylogenetic tree presented in Figure 2a. Different colors correspond to Campylobacter species with ≥3 isolates.
-
Figure 3—source data 1
This file contains the numerical values on which the graphs in Figure 3 are based.
- https://cdn.elifesciences.org/articles/73552/elife-73552-fig3-data1-v2.xlsx
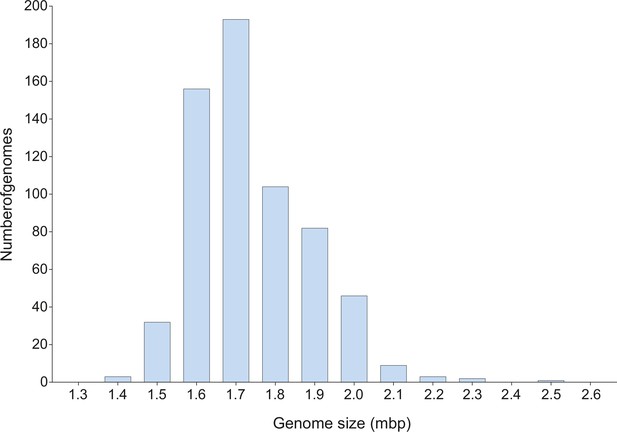
Genome size variation of the Campylobacter genus.
The frequency distribution of the genome size of all Campylobacter genomes used in this study is shown as a histogram. The number of genomes is shown on the y-axis while the genome size (in bp) on the x-axis.
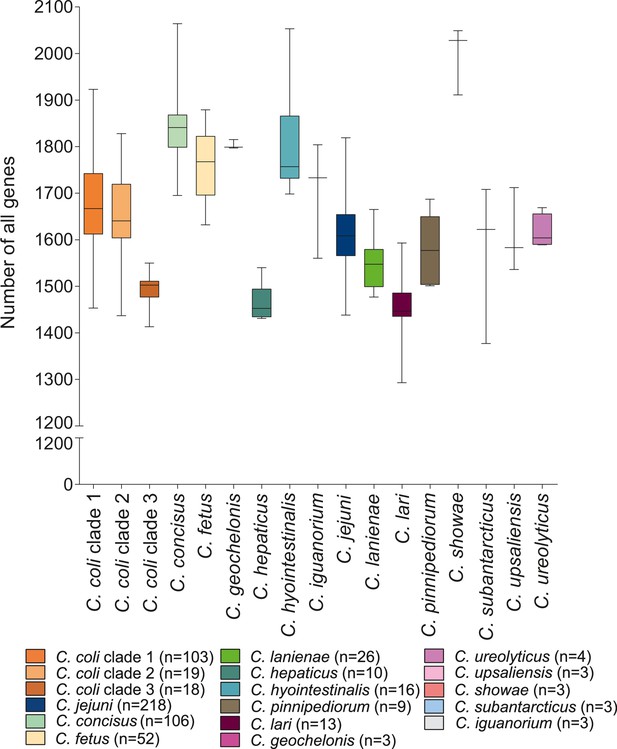
Gene variation in the genus Campylobacter.
Overall distribution of the total number of genes per isolate for each Campylobacter species (where n ≥ 3 isolates). The number of genes is shown as boxplots (min to max).
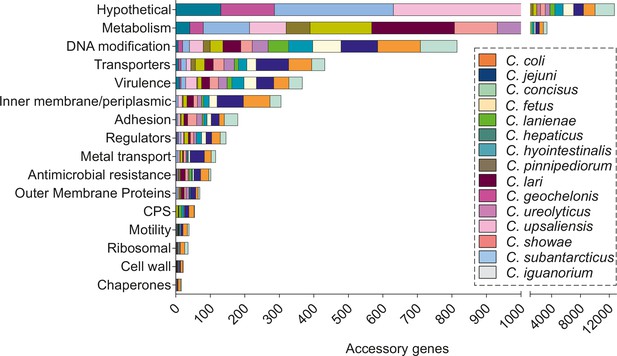
Accessory gene function in all main Campylobacter species.
The different gene functions are depicted on the y-axis, while the number of shared accessory genes on the x-axis. Different colors correspond to different Campylobacter species.
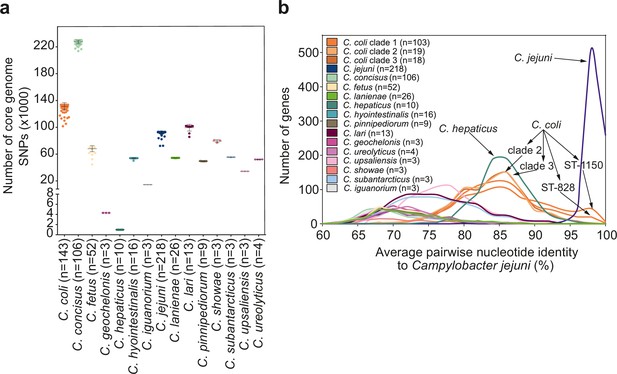
Core genome allelic variation and the effect of recombination.
(a) Number of SNPs per genome of the main Campylobacter species (where n ≥ 3 isolates) in the core genome alignment. The horizontal line in each plot represents the mean value while the upper and lower lines the standard deviation. (b) Average nucleotide identity for pairwise comparisons of 820 core genes for 605 genomes of 15 main Campylobacter species. Different colors correspond to different Campylobacter species.
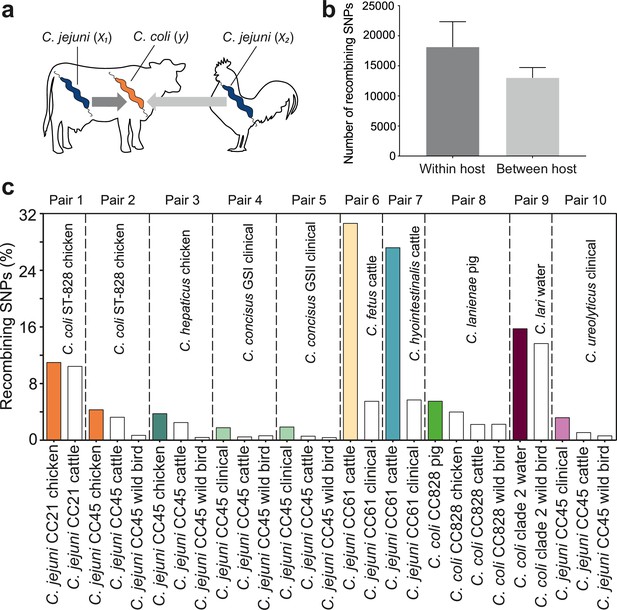
Elevated within-host interspecies recombination and donor–recipient comparisons.
(a) A hypothesis depicting the relationships between Campylobacter species, C. jejuni (x1, x2) and C. coli (y), when found in the same or in different hosts. (b) Number of recombining SNPs within and between host as inferred by chromosome painting analysis for all donor–recipient species comparisons. The error bar represents the standard error of the mean (SEM). (c) The figure shows the number of donated SNPs in 10 donor–recipient pair species comparisons. The proportion (%) of recombining SNPs with >90% probability of copying from a donor to a recipient genome is illustrated on the y-axis. All donor groups are shows in the x-axis. All colored boxes correspond to comparison where donors and recipients are found in the same host.
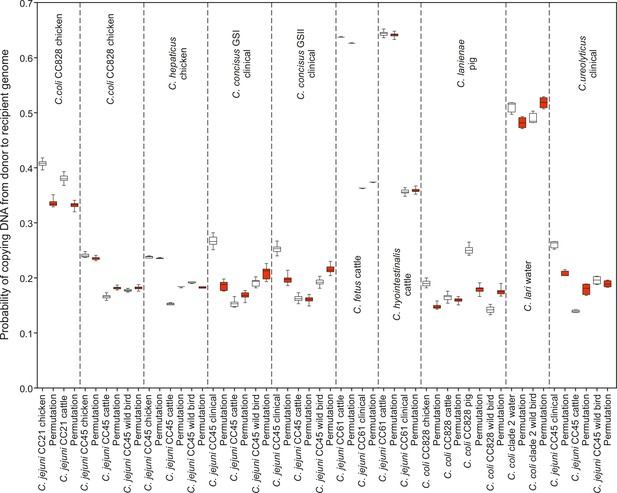
Probability of the recipient genomes sharing DNA with each donor groups is illustrated as box whiskers (white) for every donor–recipient comparison for all 10 pairs that supported our hypothesis.
The analysis where the host data were randomized across all isolates is illustrated as box whiskers (red). The probability of copying DNA from a donor to a recipient genome is shown on the y-axis. The midline in the box whiskers indicates the mean and the error bars the standard deviation.
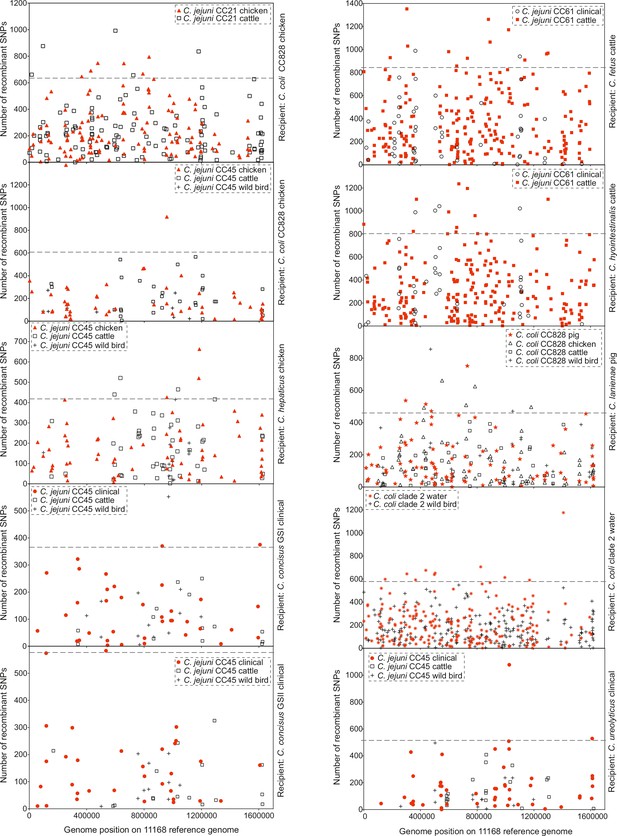
Genome position of genes containing recombining SNPs.
Genes and their corresponding number of recombining SNPs, inferred by chromosome painting analysis for all 10 species comparisons, and mapped to the NCTC11168 reference genome. Genes from within-host (red) and between-host (white) pair comparisons are shown for each comparison. Donors are isolates from chicken (triangle), cattle (square), wild bird (cross), pig (star), clinical (circle), and water (snowflake) samples. The dashed line indicates the 95th percentile for every individual group comparison.
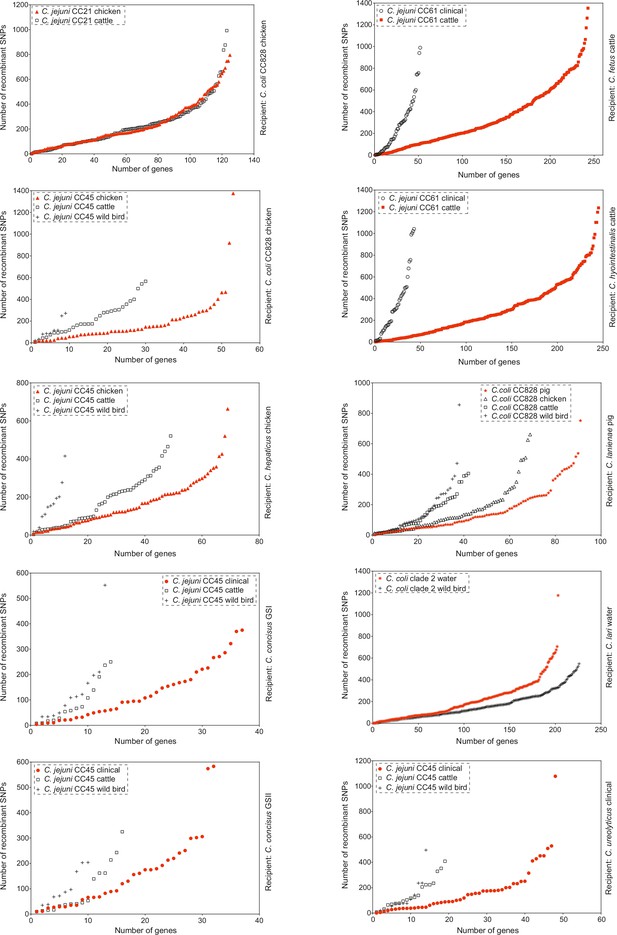
Genes ranked in ascending order of the number of recombining SNPs they contain as inferred by chromosome painting analysis for all 10 species comparisons.
Genes from within-host (red) and between-host (white) are shown for each comparison. Donors are isolates from chicken (triangle), cattle (square), wild bird (cross), pig (star), clinical (circle), and water (snowflake) samples.
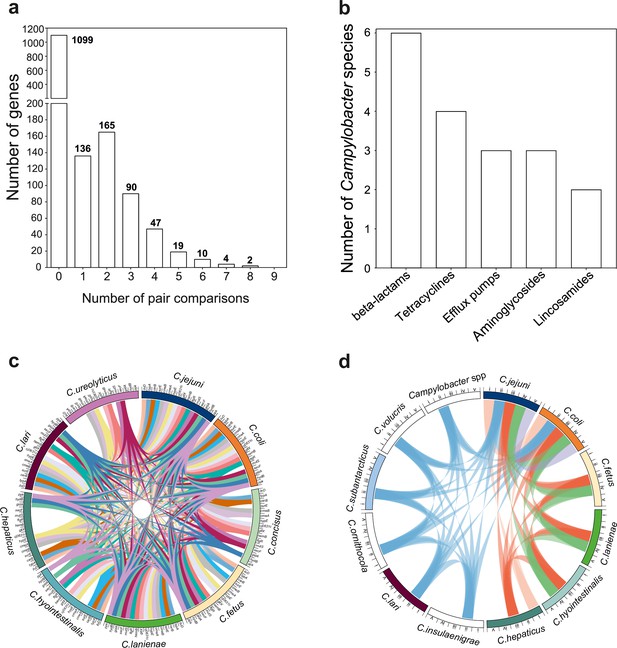
The mobilome of the Campylobacter genus.
(a) The graph illustrates the proportion of recombining genes in 10 different species comparisons. The number of species pairs in which the gene was found to recombine is shown on the x-axis, and the number of genes in each category is given on the y-axis. The exact number of genes found in each group comparison is shown on the top of each box. (b) Number of Campylobacter species harboring antimicrobial resistance (AMR) genes that belong to efflux pumps and four different antibiotic classes that are shown on the x-axis. (c) The circos plot indicates the 16 genes involved in recombination in >5 donor–recipient pair species comparisons. Gene matches are indicated by joining lines, colored differently for each gene. Gene names are shown around the perimeter for each Campylobacter species. (d) The circos plot indicates the sharing of AMR genes associated with efflux pumps and four antibiotic classes among Campylobacter species. Presence of at least one gene (not necessarily the same gene) conferring resistance to a specific antibiotic class is indicated by joining lines, colored differently for each drug class. Efflux pumps (i), β-lactams (ii), tetracyclines (iii), aminoglycosides (iv), and lincosamides (v) are shown around the perimeter for each Campylobacter species.
-
Figure 5—source data 1
This file contains the numerical values on which the graphs in Figure 5b–d are based.
- https://cdn.elifesciences.org/articles/73552/elife-73552-fig5-data1-v2.xlsx
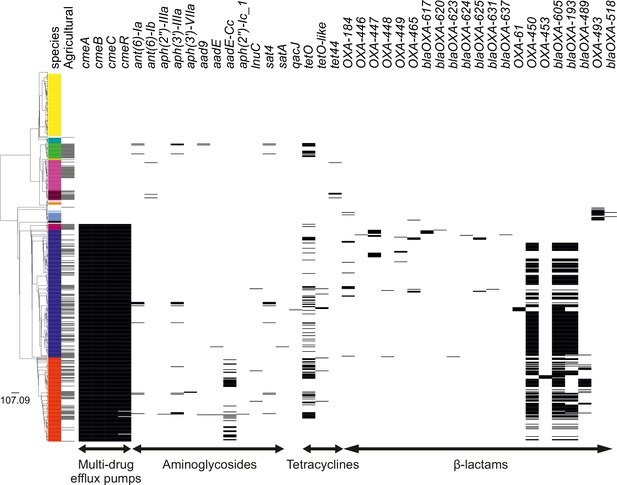
Presence of antimicrobial resistance (AMR) genes in the Campylobacter genus.
The phylogenetic tree was reconstructed using a gene-by-gene concatenated alignment of 820 core and soft-core genes and an approximation of the maximum-likelihood (ML) algorithm implemented in RAxML. The designated color scheme was used for each species in the first column. The second column indicates whether the strain is isolated from an agricultural animal (gray). Remaining columns indicate the presence of AMR genes (black). The scale represents the number of substitutions per site.
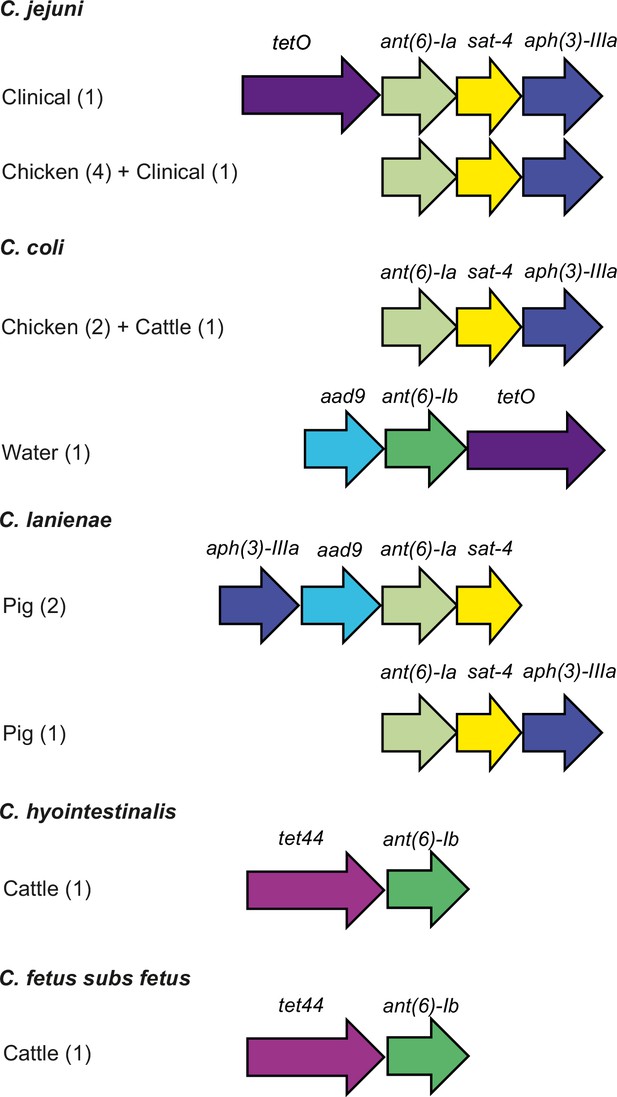
Genetic organization of antimicrobial resistance (AMR) genes in Campylobacter.
The presence of each AMR gene, highlighted in different colors, is shown for representative genomes from C. jejuni, C. coli, C. lanienae, C. hyointestinalis, and C. fetus subsp. fetus sampled from different agricultural animals. The number of isolate genomes containing each genomic arrangement is indicated in parenthesis.
Additional files
-
Supplementary file 1
Isolate information about the genomes used in this study.
- https://cdn.elifesciences.org/articles/73552/elife-73552-supp1-v2.xlsx
-
Supplementary file 2
Within-host highly (>95th percentile) recombining genes.
- https://cdn.elifesciences.org/articles/73552/elife-73552-supp2-v2.xlsx
-
Supplementary file 3
Recombination parameters as calculated by ClonalFrameML.
- https://cdn.elifesciences.org/articles/73552/elife-73552-supp3-v2.xlsx
-
Supplementary file 4
Quantifying recombination between cohabiting species using ChromoPainter.
- https://cdn.elifesciences.org/articles/73552/elife-73552-supp4-v2.xlsx
-
Supplementary file 5
Genes involved in interspecies recombination in 10 species comparisons.
- https://cdn.elifesciences.org/articles/73552/elife-73552-supp5-v2.xlsx
-
Transparent reporting form
- https://cdn.elifesciences.org/articles/73552/elife-73552-transrepform1-v2.docx