Science Forum: Building a community to engineer synthetic cells and organelles from the bottom-up
Abstract
Employing concepts from physics, chemistry and bioengineering, 'learning-by-building' approaches are becoming increasingly popular in the life sciences, especially with researchers who are attempting to engineer cellular life from scratch. The SynCell2020/21 conference brought together researchers from different disciplines to highlight progress in this field, including areas where synthetic cells are having socioeconomic and technological impact. Conference participants also identified the challenges involved in designing, manipulating and creating synthetic cells with hierarchical organization and function. A key conclusion is the need to build an international and interdisciplinary research community through enhanced communication, resource-sharing, and educational initiatives.
Philosophy of the field and past achievements
Physicists and engineers traditionally focus on the non-living world and apply model systems with reduced complexity to capture the essentials of a living entity, and to gain mechanistic insights into higher-order processes on a micro-, meso- and macroscale. This approach is also becoming more popular in the life sciences, where a ‘learning-by-building’ strategy is used to design and construct synthetic cells and organelles of reduced but defined complexity (Jia and Schwille, 2019; Ausländer et al., 2017). Early national and transnational initiatives, such as the Max Planck School ‘Matter to Life’ (https://mattertolife.maxplanckschools.org), the Build-A-Cell research coordination network (https://www.buildacell.org/), the Building a Synthetic Cell (BaSyC) research program (https://www.basyc.nl/about-basyc/), and the International Genetically Engineered Machine competition (https://igem.org/Competition), have been established to advance training, research and collaboration in this exciting new field.
Cells are the basic units of life. But their intricate structure and the tightly orchestrated interplay of individual molecular components within cells are far from basic. Most cellular phenomena are not understandable through intuition but require complex analytical systems to provide a mechanistic description of the processes forming living matter. Yet, within the complexity of living cells hide the answers to some of the most fundamental questions in the life sciences, from the emergence of life and the transition from inanimate matter to life, to the development and cure of diseases.
These compelling and profoundly difficult questions reflect a vision for the future of the field as expressed by the SynCell2020/21 early-career panelists. The philosophical and ethical considerations underlying these questions, e.g., the misuse of synthetic cells for biological warfare, the impact of synthetic cells on natural environments, or the unpredictable nature of completely new life forms, are notable for their contrast with technological and engineering-focused objectives (Gallup et al., 2021).
Using principles from biology and engineering, interdisciplinary research teams have applied synthetic cells to construct materials and hierarchical structures with life-like properties that recreate essential features of living cells and reach beyond the capabilities of natural cells (Figure 1; Elani et al., 2018; Krinsky et al., 2018; Rampioni et al., 2018; Staufer et al., 2021a; Tian et al., 2019). Cellular functions entail a diverse spectrum of modular components that regulate cell behavior. These include the ability to process chemical and physical signals from extracellular stimuli (information processing and decision making), cellular motions and adaptation to the environment (molecular adaptivity), cellular replication through division (proliferation) and nutrient uptake and garbage disposal (energy homeostasis). These abilities have helped to guide the functional design of synthetic cells and their equipment with modules that allow them to interact with the extracellular environment and to discriminate extracellular signals. For instance, extracellular morphogen sensing systems have been implemented in protocells that are able to change their structure and to discriminate between uni- and counter-directional morphogen gradients (Tian et al., 2019). Tactile behavior in the form of chemo- and phototaxis has also been engineered into synthetic cell surrogates (Bartelt et al., 2018; Pan et al., 2019).
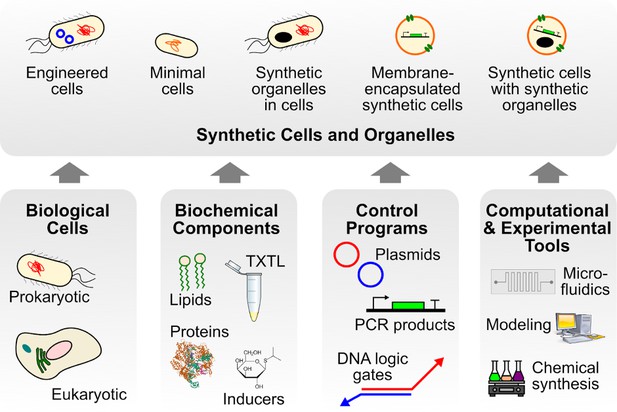
Research on engineering synthetic cells and organelles, as represented at SynCell2020/21, covers a wide range of experimental systems including engineered cells created using standard transformation techniques, minimal cells, membrane-encapsulated synthetic cells, and all of the above with the possible inclusion of engineered membraneless organelles that produce hierarchical structures.
The common objective of the field is to engineer synthetic structures with defined complexity to mimic biological systems on multiple length scales. The creation and characterization of these experimental systems draws on a wide variety of interdisciplinary inputs, including biological cells, biochemical components (such as cell-free TXTL extracts), and control programs that encode desired behavior in a variety of formats. In addition, a broad range of computational and experimental tools are required.
Broadly speaking, there are two primary approaches to construct low-complexity synthetic cells: top-down and bottom-up (Ausländer et al., 2017). Top-down approaches make use of existing living cells and sequentially remove individual components such as single genes (Lachance et al., 2019). This process can be iterated until reaching the absolute lowest point of complexity required for a cell to live. Analogous to synthetic lethality experiments in model organisms, top-down approaches provide descriptive insights into which parts of a cell are most crucial. However, it can be harder to obtain a systems-level understanding of how the parts work together.
In contrast, the bottom-up approach rationally combines non-living molecules in an understand-by-design approach to activate and exhibit the behaviors of living cells within artificial structures (Buddingh’ and van Hest, 2017). A common defining element of cellular life forms is the ability to replicate a compartmentalized information-storing and self-sustaining, out-of-equilibrium system that manifests itself in specific characteristics, which can be selected in an evolutionary process (Benner, 2010; Damiano and Stano, 2020; Porcar et al., 2011). This could be achieved by engineering a compartmentalized entity that exhibits a metabolism for reproduction purposes and environmental adaptation. For example, a lipid membrane vesicle that can grow and divide by catabolizing external substrates and harboring DNA-encoded genes that specify the enzymes required for catabolism and reproduction. The advantage of the bottom-up approach is that every component of the created system can be located and defined in a quantitative manner, together with specified interactions between molecules.
But regardless of the approach – top-down or bottom-up – the knowledge gained from building synthetic cells has the potential to provide fundamental insights into life and to shape technologies of global impact, such as new vaccination strategies (Dormitzer et al., 2013), routes to overcome antibiotic resistance (Wu et al., 2017), new manufacturing pipelines for synthetic materials (Le Feuvre and Scrutton, 2018), and alternatives to petrochemicals (Shih, 2018).
Within the last decade, the field of building synthetic cells and organelles has achieved several major technological breakthroughs. A minimal synthetic cell consisting of only 473 genes, capable of metabolizing and reproducing, has been constructed using a top-down approach (Hutchison et al., 2016). With the objective of engineering a minimal synthetic cell synthetic chromosomes have been designed to generate artificial genetic blueprints for operating synthetic cell systems (Greene et al., 2019). In addition, droplet-based synthetic cells with an artificial photosynthetic metabolism that can bind CO2 have been created, and synthetic cell systems for the scalable bio-production of natural plant products have also been built (Miller et al., 2020). Bioengineering concepts emerging from such studies have guided the implementation of new, application-focused technologies, e.g., cell-free expression systems. And most recently, the advanced synthetic genetic codes and technologies to rewire translational processes of protein production have provided the foundation for RNA-based SARS-CoV-2 vaccines (Karikó et al., 2008; Mulligan et al., 2020). Together with liposomal and lipid nanoparticle technologies, which program cellular uptake and processing of the RNA content, these vaccines have been a vital tool in the fight against COVID-19 (Park et al., 2021).
Exploring the fundamentals of life as illustrated in these brief examples requires diverse skill sets for designing and engineering experimental systems. It further needs an unbiased and creative mind with a strong interdisciplinary background to successfully integrate aspects of physics, chemistry, biology, and the information sciences. Similar to fields that explore artificial intelligence and neuromorphic computing (Valeri et al., 2020), students attracted to synthetic cell research often share enthusiasm and interests that go beyond their primary disciplines, incorporating aspects of philosophy and cognition within their research (e.g., the 'Synthetic Biology, Politics and Philosophy' workshop held at BrisSynBio Meacham and Prado Casanova, 2020). This cross-fertilization provides unique opportunities for attracting young researchers into the field of engineering synthetic cells and organelles (see Box 1 and Box 2 in Supplementary file 1 for selected quotations from young researchers who participated in the SynCell2021 Workshop).
Recent research directions and bottlenecks
The creation of compartmentalized, cell-mimicking structures and the integration of coupled transcription-translation (TXTL) systems has seen substantial progress (Garamella et al., 2019). However, considerable challenges, some seemingly paradoxical, remain. Many of these were highlighted at the recent SynCell2020/21 conference (see Figure 1—figure supplement 1 and Tables S1–S5 in Supplementary file 1 for listings of the conference program and links to recordings) and have been extensively reviewed recently (Gallup et al., 2021; Meng and Ellis, 2020).
One of the most demanding challenges remains the coupling of information-encoding systems with self-replicating cell-like entities (Walker et al., 2017), which can be framed in terms of von Neumann´s abstract generality about the logic of cell-like, self-replicating automata (von Neumann, 1966) Such entities require both a mechanism to copy the cellular architecture and functionalities that allow copying of genetic information specifying cellular structure and function. Such units need molecular systems that link the functional parts of a synthetic cell to a decoding mechanism that reads the genetic instructions required to autocatalytically build a new cell. They also require a molecular module that copies and reinserts a transcript of the (genetic) instruction into the synthetic daughter cell (Olivi et al., 2021). This is the logical basis of self-reproduction.
The first steps towards the synthetic construction of such systems were presented at the conference. DNA-encoded genetic systems represent just one implementation of self-reproduction, leaving ample room for designing alternatives to fulfil the basic conditions for a 'living', synthetic cell (Dreher et al., 2021; Otrin et al., 2021). In addition, synthetic cells will also require control programs to orchestrate the interconnected processes of sensing, response and metabolism necessary for replication and other processes relevant for life-like behavior (Lakin and Stefanovic, 2016; Li et al., 2021; Steinkühler et al., 2020).
Important progress reported at the meeting also included the engineering of synthetic structures with hierarchical organization inspired by eukaryotic life forms. Several implementations of such systems, such as hierarchical intrinsically disordered protein and nucleic acid droplets generated within synthetic cell-like compartments, were presented (Simon et al., 2017; Pérez et al., 2021). These efforts are aimed at deconvolving the organizational principles of life, including the highly dynamic cross-scale architecture of eukaryotic and multicellular organisms, most apparent during embryogenic development and tissue regeneration.
How the structural organization of subcellular, cellular and tissue components is hard-wired and how degrees of plasticity in respective structures are regulated, are problems of such immense complexity for which approaches including multi-centered global screening efforts have not been able to resolve the underlying principles. New methods based on in vitro synthetic model systems of lower complexity may provide new insights into these processes.
For example, a pivotal driving force behind tissue organization consists of genetic feedback loops based on reaction-diffusion processes and hysteresis, as first proposed by Alan Turing in his work on the chemical basis of morphogenesis in the mid-twentieth century (Turing, 1997). This is a prime example of how reductionist approaches in the form of precisely defined models can be applied to the study of complex behaviors in biological systems. Researchers in synthetic biology have recently recreated Turing patterns from protein-based systems and used these to study decision-making during cellular organization and symmetry breaking (Glock et al., 2019). This underscores both the fundamental impact of the questions asked in the field and their longstanding relevance that argues for the need to pursue novel theoretical, computational and experimental approaches by unbiased young scientists working in integrative research communities.
Other approaches have contributed insights into the spatio-temporal dynamics and organization principles of membrane-less organelles (Pérez et al., 2021). Until recently, studying such dynamic structures in living cells has mostly been limited by a lack of perturbation capabilities and the undefined chemical environment within the cytosol. However, through in vitro reconstitution of intrinsically disordered protein/nucleic acid systems in isolated low-complexity environments, quantitative insights into the molecular and thermodynamic principles needed for assembly and homeostasis of phase-separated organelles has been achieved (Linsenmeier et al., 2019). Understanding the hierarchical organization principles of life will ultimately enable the formulation of the principal laws of decision-making within living matter, and the basis of information processing and signal integration within cell collectives (Staufer et al., 2018; Staufer et al., 2019).
Engineering synthetic cells and organelles is not solely directed towards investigating biological principles, but also holds promise for practical applications. This offers the opportunity to explore an extensive technical repertoire. For instance, microfluidic approaches have been developed to assemble synthetic cells with adjustable and tunable composition. Specifically, cell-sized compartments in the form of water-in-oil droplets that contain proteins, lipids or nucleic acids, provide means of engineering systems capable of genetic information processing and artificial genotype-to-phenotype coupling, where selection is exerted at the level of the synthetic cells´ phenotype but propagation of a selected trait depends on the relevant genetic information being carried forward (Miller et al., 2020; Staufer et al., 2021b; Staufer et al., 2020; van Vliet et al., 2015; Weiss et al., 2018). Such droplet-based approaches have also been adopted for lipid membrane engineering (Lussier et al., 2021; Steinkühler et al., 2020). Similarly, DNA nanotechnology has allowed to combine programmable molecular architectures with extrinsically controlled functions (Bazrafshan et al., 2020; Jahnke et al., 2020). In a combinatorial approach, integration of DNA nano-architectures with synthetic cells has synergized top-down and bottom-up strategies (Jahnke et al., 2021). These examples demonstrate the potential for technology innovation originating from the field.
Although the reductionist approach pursued in the field of engineering synthetic cells and organelles has proven to be powerful for several lines of research, it is also confronted with systematic limitations. Foremost, as an engineering approach that iteratively reduces the complexity of living systems, the construction of synthetic cells will always be subjected to the problem of ‘hidden variables’ (Garcia et al., 2016). Unobservable or unidentified molecular components of importance to natural systems might be underrepresented or absent in the recreated in vitro system. Such unknown variables could contribute to the stochastic behavior of a system, and biological phenomena could potentially be masked behind noise effects in synthetic cells and organelles.
Moreover, living cells cannot only be considered as the simple sum of their parts. Emergence in living systems is poorly defined and understood, and the possibility to capture this aspect by a modular approach remains to be evaluated. A second systematic limitation of the reductionist approach lies in the fact that reduced complexity does not directly imply increased understanding of a biological phenomenon. This is well illustrated by the creation of minimal bacterial genomes with only 473 genes (Hutchison et al., 2016). Despite the remarkably reduced complexity and size of this genome, 149 of the genes are still of unknown function. Addressing these limitations provides opportunities for ongoing research and collaborative efforts, even as progress continues in advancing understanding and functional applications of synthetic cells and organelles.
Future perspectives and community
Engineering approaches set the stage for implementing synthetic functional modules capable of performing specific functions in synthetic cells. The successful combination of all individual elements within a single entity will be key to assemble synthetic living cells. This, in turn, requires integrated inter-laboratory solutions that allow for off-the-shelf unification of individual modules. Exchanging expertise between laboratories and universal module interfaces will be essential and will enable broad participation in the field.
Discussions during the SynCell2020/21 revealed several fundamental strategic frameworks and infrastructure that are needed to achieve such a successful integration of the global community. Firstly, in the interest of effective paywall-free knowledge transfer among researchers, open-access data repositories are needed. This will facilitate transfer of experimental protocols and sharing of data and blueprints for synthetic cell modules, effectively boosting access of interested students to the field. Moreover, standardization efforts that strive to provide universal norms for the design and assembly of synthetic cell modules and interfaces need to be developed. Specific implementations of such platforms could be arranged, inspired by the collaborative software development and version control platform GitHub, which has experienced community-wide acceptance within computer science and engineering fields. The Build-A-Cell network has embraced this approach and has begun to assemble such open-access repositories.
Secondly, engineering synthetic cells and organelles will be a model for new transcontinental educational modalities. SynCell2020/21 was organized by the National Science Foundation (USA) and the Max Planck Society (Germany). It also received support from national research programs, e.g., the Build-A-Cell network (USA-based) and the BaSyC program (Netherlands; Figure 1—figure supplement 1). Presentations by leading researchers in student-centered tutorials were a focus of the conference framework. Community-driven education programs for specialized training in relevant domains (biology, physics, chemistry, microbiology, molecular biology, biophysics, computer science or ethics) will be key for equipping new generations with the necessary skills to successfully engineer living synthetic cells and organelles. International workshops and research summer schools will be important to develop a coherent, long-lasting community that fosters cross-generational collaborations among scholars.
At present, only a limited number of training and graduate programs focused on the engineering of synthetic cells and organelles have been established, such as the Max Planck School ‘Matter to Life’, the Cold Spring Harbor Laboratory Summer School on Synthetic Biology, and research programs supported by the US National Science Foundation ‘Rules of Life’ initiative. Their successful implementation will not only nurture the next generation of scientists but will also train a cohort of researchers to enable industrial applications. If possible, future events should be organized between all major research and teaching initiatives (Figure 1—figure supplement 1) to bring together the global expertise and emerging talent, and to promote a broad distribution of thought leadership across institutions as the field continues to grow and develop.
Lastly, following the learning-by-building approach, the field awaits a steadily growing demand for an integrated research infrastructure that provides computational power and specialized courses in molecular and genetic design. This includes molecular modelling of large-scale, whole-cell models to predict the interactions of engineered components with host cells. Access to advanced computational facilities and enhanced algorithms for simulations based on machine learning and optimization techniques, will greatly expand the scope for designing and constructing synthetic cells and organelles. Dedicated research centers, such as the Max Planck–Bristol Center for Minimal Biology, could provide such facilities, as well as technical support for the increasingly important administrative aspects to the field, including technology transfer procedures, handling of intellectual property issues, and curation of specialized genetic parts and molecular module libraries specified for the field (inspired by biobanks such as Addgene and large-scale gene and genome synthesis 'bio foundries', such as those funded by the Department of Energy in the US).
For all the proposed measures, commitment and support from funding bodies, political and regulatory authorities, and universities with established teaching infrastructure, will be essential. Especially to successfully install a strategic, open-source platform for synthetic biology and student exchange programs, like the ones between the University of New Mexico and the Max Planck Society.
We also observe that connections to and inspiration drawn from other research communities will be important. For example, research advances addressing origin-of-life questions, the basic principles of life, and the exploration of eukaryogenesis, connect many scientific themes that arise in the study of synthetic cells and organelles. This was highlighted by the observation that many SynCell2020/21 participants are also active in these related communities. Furthermore, expanding the research community in synthetic cells to connect to these and other related scientific communities opens additional opportunities for research support, including that available from private and philanthropic foundations. Recent examples of such initiatives include the 'Life? – A Fresh Scientific Approach to the Basic Principles of Life' program, supported by the Volkswagen Foundation, and the 'Project on the Origin of the Eukaryotic Cell', sponsored jointly by the Gordon and Betty Moore Foundation and the Simons Foundation.
Specific measures will include a joint program between the research initiatives mentioned above, aimed towards continued organization of the SynCell conference as a think-tank for community building and research exchange. Moreover, the Build-a-Cell initiative has initiated several focused working groups, e.g., working towards collection and annotation of synthetic cell subtype components or towards establishing in silico modeling frameworks of synthetic cells with predictable behavior. These groups provide an optimal platform to develop future cross-scale-organized infrastructure that will be able to manage between different stakeholders from academia, industry and political authorities, while also serving as an advisory council representing the field’s interest. Furthermore, concentrated efforts will be made to raise awareness in academic faculties and scientific societies towards the importance of establishing relevant teaching schemes in graduate and undergraduate programs.
A compelling model for developing and sharing modular tools across the diverse synthetic biology community can be found in the design of the original Unix multi-user operating system and subsequent community-driven, evolutionary development of Linux (Raymond and Young, 2001). Unix’s 'graceful facilities' enabled users to create complex programs by using software 'pipes' to compose simple modules together: at the same time, the operating system was designed to facilitate communication among programmers as 'the essence of communal computing’ (as seen in the video ‘The Unix System: Making Computers Easier to Use’). Linux emerged from an unprecedented, worldwide open-source effort by volunteer programmers. These core values of streamlined, modular design and enthusiastic, open, collaborative development can similarly inform and shape progress in the synthetic cell community.
Conclusions
SynCell2020/21 demonstrated remarkable engagement of a large and geographically diverse community, and potential for global collaboration and transcontinental knowledge-sharing as the foundation for future success in the field. Importantly, a collaborative and well-trained community, including a new generation of young scholars, will be able to communicate the societal impacts of engineering synthetic cells and organelles responsibly and effectively to the public. Particularly with respect to questions of how to share intellectual property to benefit humanity while continuing to reward innovation, biosafety, biosecurity and other unique ethical and philosophical considerations, including the most fundamental question of all: 'what is life?'.
Data availability
This Feature Article does not contain any primary data.
References
-
Synthetic biology – the synthesis of biologyAngewandte Chemie International Edition 56:6396–6419.https://doi.org/10.1002/anie.201609229
-
Light-guided motility of a minimal synthetic cellNano Letters 18:7268–7274.https://doi.org/10.1021/acs.nanolett.8b03469
-
Artificial cells: synthetic compartments with life-like functionality and adaptivityAccounts of Chemical Research 50:769–777.https://doi.org/10.1021/acs.accounts.6b00512
-
On the “life-likeness” of synthetic cellsFrontiers in Bioengineering and Biotechnology 8:953.https://doi.org/10.3389/fbioe.2020.00953
-
Synthetic generation of influenza vaccine viruses for rapid response to pandemicsScience Translational Medicine 5:185ra68.https://doi.org/10.1126/scitranslmed.3006368
-
Division and regrowth of phase-separated giant unilamellar vesicles*Angewandte Chemie 60:10661–10669.https://doi.org/10.1002/anie.202014174
-
Ten future challenges for synthetic biologyEngineering Biology 5:51–59.https://doi.org/10.1049/enb2.12011
-
TXTL-based approach to synthetic cellsMethods in Enzymology 617:217–239.https://doi.org/10.1016/bs.mie.2018.12.015
-
Using synthetic biology to make cells tomorrow’s test tubesIntegrative Biology 8:431–450.https://doi.org/10.1039/c6ib00006a
-
Stationary patterns in a two-protein reaction-diffusion systemACS Synthetic Biology 8:148–157.https://doi.org/10.1021/acssynbio.8b00415
-
Engineering synthetic chromosomes by sequential loading of multiple genomic payloads over 100 kilobase pairs in sizeMolecular Therapy. Methods & Clinical Development 13:463–473.https://doi.org/10.1016/j.omtm.2019.04.006
-
Bottom-up synthetic biology: reconstitution in space and timeCurrent Opinion in Biotechnology 60:179–187.https://doi.org/10.1016/j.copbio.2019.05.008
-
Synthetic cells synthesize therapeutic proteins inside tumorsAdvanced Healthcare Materials 7:e1701163.https://doi.org/10.1002/adhm.201701163
-
Supervised learning in adaptive DNA strand displacement networksACS Synthetic Biology 5:885–897.https://doi.org/10.1021/acssynbio.6b00009
-
A living foundry for synthetic biological materials: a synthetic biology roadmap to new advanced materialsSynthetic and Systems Biotechnology 3:105–112.https://doi.org/10.1016/j.synbio.2018.04.002
-
Dynamics of Synthetic Membraneless Organelles in Microfluidic DropletsAngewandte Chemie 58:14489–14494.https://doi.org/10.1002/anie.201907278
-
Can Bottom-Up Synthetic Biology Generate Advanced Drug-Delivery Systems?Trends in Biotechnology 39:445–459.https://doi.org/10.1016/j.tibtech.2020.08.002
-
The second decade of synthetic biology: 2010-2020Nature Communications 11:5174.https://doi.org/10.1038/s41467-020-19092-2
-
Towards a synthetic cell cycleNature Communications 12:4531.https://doi.org/10.1038/s41467-021-24772-8
-
Non-viral COVID-19 vaccine delivery systemsAdvanced Drug Delivery Reviews 169:137–151.https://doi.org/10.1016/j.addr.2020.12.008
-
DNA binding by an intrinsically disordered elastin-like polypeptide for assembly of phase separated nucleoprotein coacervatesIndustrial & Engineering Chemistry Research 60:17408–17416.https://doi.org/10.1021/acs.iecr.1c02823
-
The ten grand challenges of synthetic lifeSystems and Synthetic Biology 5:1–9.https://doi.org/10.1007/s11693-011-9084-5
-
Synthetic cells produce a quorum sensing chemical signal perceived by Pseudomonas aeruginosaChemical Communications 54:2090–2093.https://doi.org/10.1039/c7cc09678j
-
BookThe Cathedral and the Bazaar: Musings on Linux and Open Source by an Accidental RevolutionaryO’Reilly & Associates, Inc.
-
Protease-resistant cell meshworks: an indication of membrane nanotube-based syncytia formationExperimental Cell Research 372:85–91.https://doi.org/10.1016/j.yexcr.2018.09.012
-
Artificial morphogen-mediated differentiation in synthetic protocellsNature Communications 10:3321.https://doi.org/10.1038/s41467-019-11316-4
-
The chemical basis of morphogenesisPhilosophical Transactions of the Royal Society of London. Series B, Biological Sciences 237:37–72.https://doi.org/10.1098/rstb.1952.0012
-
BookFrom Matter to Life: Information and CausalityCambridge University Press.https://doi.org/10.1017/9781316584200
-
Quantitative and synthetic biology approaches to combat bacterial pathogensCurrent Opinion in Biomedical Engineering 4:116–126.https://doi.org/10.1016/j.cobme.2017.10.007
Decision letter
-
Helga GrollReviewing Editor; eLife, United Kingdom
-
Peter RodgersSenior Editor; eLife, United Kingdom
-
Atul ParikhReviewer
-
Erdinc SezginReviewer
In the interests of transparency, eLife publishes the most substantive revision requests and the accompanying author responses.
Decision letter after peer review:
Thank you for submitting your article "Building a community to engineer synthetic cells and organelles from the bottom-up" to eLife for consideration as a Feature Article. Your article has been reviewed by 3 peer reviewers, and the evaluation has been overseen by 2 members of the eLife Features Team (Helga Groll and Peter Rodgers). The following individuals involved in review of your submission have agreed to reveal their identity: Atul Parikh, Erdinc Sezgin
The reviewers and editors have discussed the reviews and we have drafted this decision letter to help you prepare a revised submission.
Summary:
This is a nicely written article that summarizes the proceedings of the SynCell 2020/2021 conference, which brought together a diverse group of early-stage and senior researchers focused on designing, manipulating and creating cell-like synthetic activities.
The report highlights the importance of building an international and multidisciplinary community dedicated to developing the fundamental understanding and technical know-how to create synthetic cells from the bottom up. It discusses achievements within the emerging field, projects broader impacts, including socioeconomic and technological ones, and identifies challenges and opportunities. It concludes with a call for building an integrated research community through resource-sharing, enhanced communication, and cross-institutional and transnational educational initiatives.
The fascinating convergence of scientific and technological knowledge with philosophical and ethical considerations will inspire early-career scholars and bring transformative innovations to society.
Essential revisions:
1. To an engaged and informed readership, the article, would serve as a valuable resource and a roadmap if the conference proceedings (a) identified specific milestones, (b) highlighted major accomplishments, and (c) tabulated specific challenges and opportunities that must be addressed to meet the aspirations of the field.
A graphic or a table highlighting the timeline/milestones/opportunities and projecting the future would prove useful.
2. The description of the perceived limitations of the approach needs elaborating. Many features of complex systems are often lost when complexities are reduced and systems dissected to their presumed essential ingredients. But which emergent aspects are important? What does minimal complexity entail? How does minimal complexity change with the increased functionality, adaptability and survivability?
3. The discussion in the role of function in guiding the cellular design appears to be conspicuously missing. What ingredients must be incorporated to recapitulate some of the most basic abilities of the living cell, such as surviving in harsh environments, processing physical and chemical signals from their local environment, making decisions, i.e., selecting a specific phenotype (e.g., gene expression, morphology, organization) from a range of possibilities in response to an extracellular change, or identifying signals from noisy backgrounds?
4. The discussion on hierarchical organization is particularly interesting. I recommend considering insights from the growing body of literature on eukaryogenesis, including possibly a description of the initiative from the Simons-Moore Foundation.
5. It would be important to contemplate on the inclusivity aspect of the field while providing a perspective on the future of this discipline. Almost all research areas are dominated by a few established people or family-like consortiums – mostly from established countries, which makes it difficult for the young researchers to be part of the community. I cannot say this is the case for this effort authors are presenting here, but it needs to be kept in mind. Clearly, a few programs (such as Max Planck programs) have taken the initiative for this endeavour, but future steps would be stronger if more parties are involved regardless of their geographical whereabouts, career stage, or previous involvement in the established network.
https://doi.org/10.7554/eLife.73556.sa1Author response
Essential revisions:
1. To an engaged and informed readership, the article, would serve as a valuable resource and a roadmap if the conference proceedings (a) identified specific milestones, (b) highlighted major accomplishments, and (c) tabulated specific challenges and opportunities that must be addressed to meet the aspirations of the field.
A graphic or a table highlighting the timeline/milestones/opportunities and projecting the future would prove useful.
We thank the reviewers for bringing this point to our attention and we agree that these are important aspects to be considered in the community. Several very complete reviews have been published on these matters recently. For instance, Gallup et al., tabulate in an up-to-date review the 10 future challenges in the field and Meng et al., have extensively reviewed and illustrated the achievements of the field in the last decade. However, the major focus of our Feature Article lies inidentifying the infrastructural, educational and collaborative bottlenecks and opportunities in the field. In the view that the aspects raised by the reviewers are of importance for an informed readership, we now direct the reader to the reviews mentioned above in the revised version of our manuscript, and write (page 8, line 6):
“Many of these were highlighted at the recent SynCell2020/21 conference (see Supplementary Box 2 and Supplementary Information for listings of the conference program and links to recordings) and have been extensively reviewed recently by Gallup et al., and Meng et al.,7,32.”
Moreover, we now provide an additional supplementary figure in the revised version of our manuscript that summarizes quotes collected from early career panelists of the SynCell2021 workshop on specific challenges in the field.
2. The description of the perceived limitations of the approach needs elaborating. Many features of complex systems are often lost when complexities are reduced and systems dissected to their presumed essential ingredients. But which emergent aspects are important? What does minimal complexity entail? How does minimal complexity change with the increased functionality, adaptability and survivability?
We agree with the reviewers that our article would benefit from a more balanced description of the systematic limitations of the reductionist approach. In order to draw the reader’s attention to such issues, we have now added one paragraph to our revised manuscript that details two systematic limitations underlying the field and the constraints they entail (page 11, line 4):
“Although the reductionist approach pursued in the field of engineering synthetic cells and organelles has proven to be powerful for several lines of research, it is also confronted with systematic limitations. Foremost, as an engineering approach that iteratively reduces the complexity of living systems, the construction of synthetic cells will always be subjected to the problem of “hidden variables''55. Unobservable or unidentified molecular components of importance to natural systems might be underrepresented or absent in the recreated in vitro system. As such variables are by definition unknown and can contribute to the stochastic behavior of a system, biological phenomena could potentially be masked behind noise effects in synthetic cells and organelles. Moreover, living cells can not only be considered as the simple sum of their parts. Emergence in living systems is poorly defined and understood, and the possibility to capture this aspect by a modular approach remains to be evaluated. A second systematic limitation of the reductionist approach lies in the fact that reduced complexity does not directly imply increased understanding of a biological phenomenon. This is well illustrated by the creation of minimal bacterial genomes with only 473 genes23. Despite the remarkably reduced complexity and size of this genome, 149 of the genes are still of unknown function. Addressing these limitations provides opportunities for ongoing research and collaborative efforts, even as progress continues in advancing understanding and functional applications of synthetic cells and organelles.”
3. The discussion in the role of function in guiding the cellular design appears to be conspicuously missing. What ingredients must be incorporated to recapitulate some of the most basic abilities of the living cell, such as surviving in harsh environments, processing physical and chemical signals from their local environment, making decisions, i.e., selecting a specific phenotype (e.g., gene expression, morphology, organization) from a range of possibilities in response to an extracellular change, or identifying signals from noisy backgrounds?
We agree with the reviewers that providing a description of basic cellular functions, especially those associated with signal integration and sensing, would further highlight the challenges of synthetic cell engineering and modular aspects of the approach. We therefore now write on page 3, line 25 of our revised manuscript:
“Cellular functions entail a diverse spectrum of modular components that regulate cell behavior. Such cellular functions include but are not limited to the ability to process chemical and physical signals from extracellular stimuli (information processing and decision making), cellular motions and adaptation to the environment (molecular adaptivity), cellular replication through division (proliferation) and nutrient uptake and garbage disposal (energy homeostasis). These abilities have helped to guide the functional design of synthetic cells and their equipment with modules that allow for their interaction with the extracellular environment and discrimination of extracellular signals. For instance, extracellular morphogen sensing systems have been implemented in protocells that allow for structural adaptation in a reaction-diffusion model and discrimination between uni-directional and counter-directional morphogen gradients11. Moreover, tactile behaviour in the form of chemo- and phototaxis has been engineered into synthetic cell surrogates12,13.”
4. The discussion on hierarchical organization is particularly interesting. I recommend considering insights from the growing body of literature on eukaryogenesis, including possibly a description of the initiative from the Simons-Moore Foundation.
We appreciate the suggestion of the reviewer and agree that eukaryogenesis, as well as other related topics such as origin-of-life research, are closely related to questions addressed in the current submission. Indeed, many of the participants in SynCell2020/21 are active researchers in these and other related areas. Although an extensive discussion of recent advances in these areas extends beyond the scope or our submission, we have made revisions to highlight the existence of these connections and also the importance of foundation support. To that end, the third-to-last paragraph now reads:
“For all the proposed measures, commitment and support from funding bodies, political and regulatory authorities as well as universities with established teaching infrastructure will be essential. This is most important for the successful installation of a strategic open-source platform for synthetic biology and student exchange programs, such as now established between the University of New Mexico and the Max Planck Society. We also observe that connections to and inspiration drawn from other research communities will be important. For example, research advances addressing origin-of-life questions, investigation of basic principles of life, and exploration of eukaryogenesis connect to many scientific themes that arise in the study of synthetic cells and organelles. This connection is highlighted by the observation that many SynCell2020/21 participants are also active in these related communities. Furthermore, expansion of the research community in synthetic cells to connect to these and other related scientific communities opens additional opportunities for research support, including that available from private and philanthropic foundations. Recent examples of such initiatives include the "Life?" program championed by the Volkswagen Foundation57 and the "Project on the Origin of the Eukaryotic Cell," sponsored jointly by the Gordon and Betty Moore Foundation and the Simons Foundation58.”
5. It would be important to contemplate on the inclusivity aspect of the field while providing a perspective on the future of this discipline. Almost all research areas are dominated by a few established people or family-like consortiums – mostly from established countries, which makes it difficult for the young researchers to be part of the community. I cannot say this is the case for this effort authors are presenting here, but it needs to be kept in mind. Clearly, a few programs (such as Max Planck programs) have taken the initiative for this endeavour, but future steps would be stronger if more parties are involved regardless of their geographical whereabouts, career stage, or previous involvement in the established network.
While we agree that there are significant inequities in accessibility to the field of synthetic cells and organelles faced by aspiring researchers across the world (as there are in other emerging fields), we believe that the SynCell2020/21 symposium activities and this manuscript are precisely a significant effort to address these inequities. What initially was envisioned as a somewhat exclusive in-person conference (with 150 attendees) in a somewhat remote location (Santa Fe, NM), was, by necessity engendered by the pandemic, transformed into a widely-advertised, free, virtual conference with a huge number of participants (~750) from across 6 continents. As indicated in the manuscript, the symposium provided several activities specifically geared toward young participants including tutorials, poster sessions and, importantly, a post-conference workshop that formed the basis for the manuscript itself, for which one half of the 22 coauthors are young researchers (i.e., students or postdocs). A major component of the workshop was a session specifically designed to elicit creative new ideas for expanding the international reach of educational and research opportunities, while advancing the field and its technological capabilities in ways that resonate with a new generation. The symposium activities and this manuscript (including the index of available online recordings) raise awareness of global efforts and networks dedicated to synthetic cell research; these resources further highlight ongoing activities in which researchers outside these networks can participate. Notably, as a direct result of the pandemic, there are now numerous online synthetic cell colloquia and seminar series, highlighted by SynCell2020/21 speakers, that are readily accessed from anywhere in the world live or as YouTube recordings. We therefore feel strongly that our manuscript does indeed contemplate inclusivity as well as a vision of the future of the discipline by describing activities geared toward engaging young, ethnically diverse, and geographically diverse researchers. Indeed, this was a major motivating factor for our submission of this manuscript to eLife, an open access journal. We have made several additions to the manuscript text to emphasize our vision to enhance diversity, equity and inclusion in the emerging field of synthetic cells and organelles.
We thank the reviewers for sharing our mutual concern for inclusion as the field of synthetic cells and organelles matures; it is incumbent upon us (as the reviewers suggest) to continue to strive for accessibility of this field to researchers regardless of their institutional locale, professional stage or previous connections. We are dedicated to this task and several of us are already engaging in the organization of SynCell2022, for which we are advocating for the retention of the virtual component, as well as planning fundraising activities to enable widespread virtual attendance and in-person attendance of diverse, young researchers. In this manner, we hope to instill a culture of accessibility and inclusion in this important emerging field.
https://doi.org/10.7554/eLife.73556.sa2Article and author information
Author details
Funding
National Science Foundation (CBET-1841170)
- Gabriel P López
Max Planck Society (Max Planck School Matter to Life)
- Joachim P Spatz
New Mexico Consortium
- Gabriel P López
The funders had no role in study design, data collection and interpretation, or the decision to submit the work for publication.
Acknowledgements
The authors thank all the speakers and participants of the SynCell2020/21 and associated activities for their critical input, inspiring discussions and engaged participation. The National Science Foundation (CBET-1841170), the Max Planck Society, the New Mexico Consortium and the University of New Mexico are acknowledged for their financial support.
Publication history
- Received:
- Accepted:
- Accepted Manuscript published:
- Version of Record published:
Copyright
© 2021, Staufer et al.
This article is distributed under the terms of the Creative Commons Attribution License, which permits unrestricted use and redistribution provided that the original author and source are credited.
Metrics
-
- 3,068
- views
-
- 417
- downloads
-
- 35
- citations
Views, downloads and citations are aggregated across all versions of this paper published by eLife.
Download links
Downloads (link to download the article as PDF)
Open citations (links to open the citations from this article in various online reference manager services)
Cite this article (links to download the citations from this article in formats compatible with various reference manager tools)
Further reading
-
- Biochemistry and Chemical Biology
- Structural Biology and Molecular Biophysics
The two identical motor domains (heads) of dimeric kinesin-1 move in a hand-over-hand process along a microtubule, coordinating their ATPase cycles such that each ATP hydrolysis is tightly coupled to a step and enabling the motor to take many steps without dissociating. The neck linker, a structural element that connects the two heads, has been shown to be essential for head–head coordination; however, which kinetic step(s) in the chemomechanical cycle is ‘gated’ by the neck linker remains unresolved. Here, we employed pre-steady-state kinetics and single-molecule assays to investigate how the neck-linker conformation affects kinesin’s motility cycle. We show that the backward-pointing configuration of the neck linker in the front kinesin head confers higher affinity for microtubule, but does not change ATP binding and dissociation rates. In contrast, the forward-pointing configuration of the neck linker in the rear kinesin head decreases the ATP dissociation rate but has little effect on microtubule dissociation. In combination, these conformation-specific effects of the neck linker favor ATP hydrolysis and dissociation of the rear head prior to microtubule detachment of the front head, thereby providing a kinetic explanation for the coordinated walking mechanism of dimeric kinesin.
-
- Structural Biology and Molecular Biophysics
The canonical chemokine receptor CXCR4 and atypical receptor ACKR3 both respond to CXCL12 but induce different effector responses to regulate cell migration. While CXCR4 couples to G proteins and directly promotes cell migration, ACKR3 is G-protein-independent and scavenges CXCL12 to regulate extracellular chemokine levels and maintain CXCR4 responsiveness, thereby indirectly influencing migration. The receptors also have distinct activation requirements. CXCR4 only responds to wild-type CXCL12 and is sensitive to mutation of the chemokine. By contrast, ACKR3 recruits GPCR kinases (GRKs) and β-arrestins and promiscuously responds to CXCL12, CXCL12 variants, other peptides and proteins, and is relatively insensitive to mutation. To investigate the role of conformational dynamics in the distinct pharmacological behaviors of CXCR4 and ACKR3, we employed single-molecule FRET to track discrete conformational states of the receptors in real-time. The data revealed that apo-CXCR4 preferentially populates a high-FRET inactive state, while apo-ACKR3 shows little conformational preference and high transition probabilities among multiple inactive, intermediate and active conformations, consistent with its propensity for activation. Multiple active-like ACKR3 conformations are populated in response to agonists, compared to the single CXCR4 active-state. This and the markedly different conformational landscapes of the receptors suggest that activation of ACKR3 may be achieved by a broader distribution of conformational states than CXCR4. Much of the conformational heterogeneity of ACKR3 is linked to a single residue that differs between ACKR3 and CXCR4. The dynamic properties of ACKR3 may underly its inability to form productive interactions with G proteins that would drive canonical GPCR signaling.