Endogenous protein tagging in medaka using a simplified CRISPR/Cas9 knock-in approach
Figures
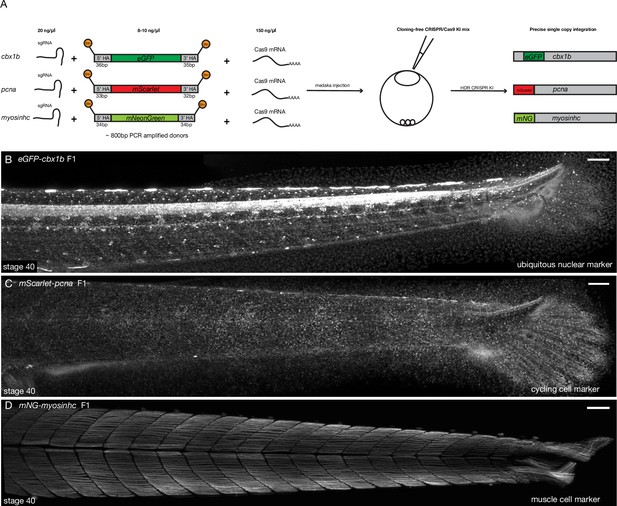
Cloning-free single-copy CRISPR/Cas9-mediated knock-in (KI) lines in medaka.
(A) Schematic diagram of cloning-free CRISPR knock-in strategy. The injection mix consists of three components, a single-guide RNA (sgRNA) targeting the gene of interest, Cas9-mSA mRNA, and the PCR-amplified donor fragment containing short homology arms on both ends (30–40 bp) and the fluorescent protein of interest with no ATG and no stop codon. Note that the 5′ ends of the PCR donor fragment are biotinylated (Btn). The mix is injected in one-cell staged medaka embryos and the injected fishes are screened for potential in-frame integrations mediated by homology-directed repair (HDR). (B) eGFP-cbx1b F1 CRISPR KI line stage 40 medaka embryos. eGFP-Cbx1b labels all nuclei and is thus an ubiquitous nuclear marker. n > 10 embryos. Scale bar = 100 µm (C) mScarlet-pcna F1 CRISPR KI line stage 40 medaka embryos. mScarlet-Pcna labels exclusively cycling cells. n > 10 embryos. Scale bar = 100 µm. (D) mNG-myosinhc F1 CRISPR KI line stage 40 medaka embryos. mNG-Myosinhc labels exclusively muscle cells located in the myotome tissue of medaka embryos. n > 10 embryos. Scale bar = 100 µm.
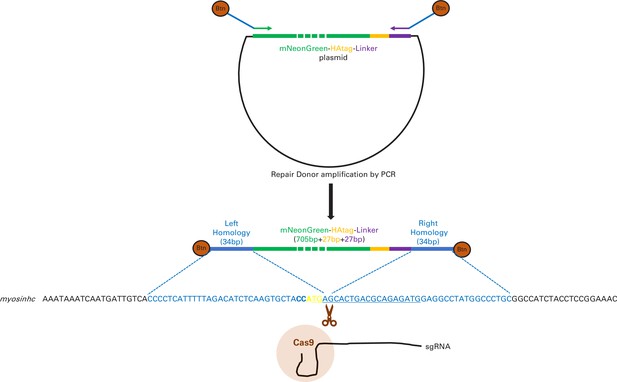
Schematic representation of mNeonGreen-myosinhc tagging strategy.
PCR amplification of mNeonGreen-HAtag-Linker (green, orange, and purple) using primers homologous to the extremity of the insert and containing flanking sequence corresponding to the homology arms (blue) for insertion just downstream the ATG of myosinhc gene (yellow) following Cas9/sgRNA DNA-induced cut. The PCR primers contain 5′ end Biotins (Btn). Bold letters denote the single-guide RNA (sgRNA) PAM, underlined the sequence upstream the PAM recognized by the sgRNA, blue letters the sequence homologous between the myosinhc locus and the PCR donor, dashed green for mNeonGreen indicates it is not to scale.
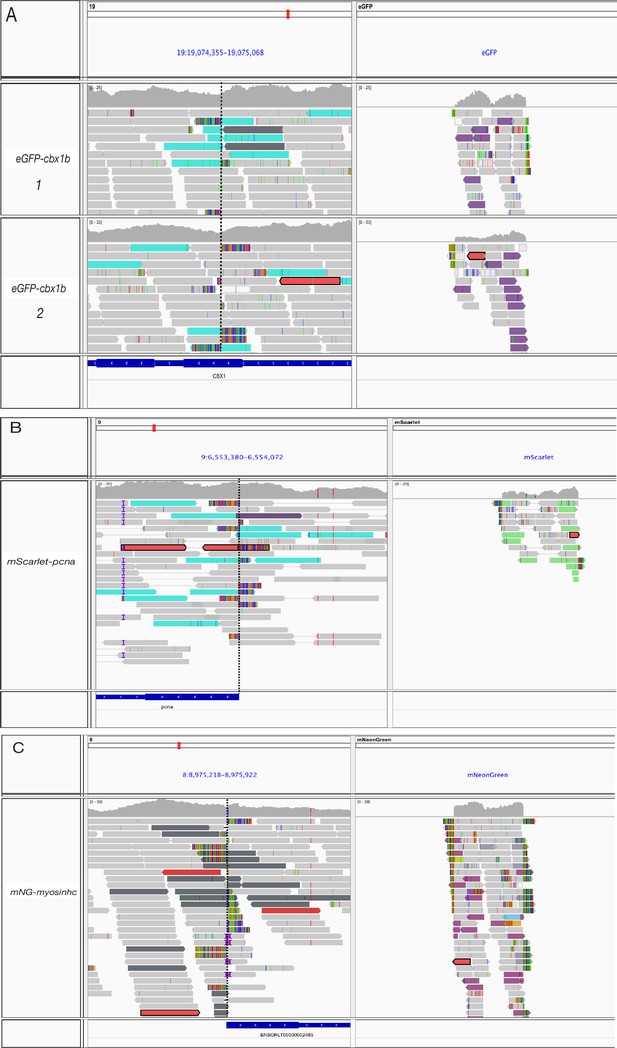
Alignment of whole genome sequencing (WGS) reads to fluorescent protein sequences.
(A) eGFP integration in cbx1b locus. Paired-end sequenced reads from eGFP-cbx1b F1 embryos, two biological replicates (gDNA1 and gDNA2) from the same F0 founder are shown in the upper and lower panels, coloured in grey for concordant mappings and coloured in turquoise/purple for inter-chromosomal paired-ends with one mate mapped to chr19 (left panel) and one mate mapped to eGFP (right panel). The predicted integration site is shown as a vertical dashed line with soft-clipped reads (shown as coloured bases) to the left and right of the integration site. Above the sequenced reads are the basepair-level coverage histogram. The results indicate that eGFP integrated only at the cbx1b locus genome wide as we could not detect eGFP reads anchored anywhere else in the genome. Purple (right)/turquoise (left) bars are of particular importance as they represent reads that span both the eGFP and the endogenous cbx1b locus. The two dark grey bars represent unpaired mate, mate not mapped, or otherwise unknown status. The mapping quality of these two reads is 0 and therefore they most likely represent false mappings. The red bars are anomalous mappings that either deviate from the expected insert size or paired-end mapping orientation. Coverage of eGFP-cbx1b_gDNA1 is 20.4× and for eGFP-cbx1b_gDNA2 is 23.6×. (B) mScarlet integration in pcna locus. Paired-end sequenced reads from mScarlet-pcna F1 embryos, coloured in grey for concordant mappings and coloured in turquoise/green for inter-chromosomal paired-ends with one mate mapped to chr9 (left panel) and one mate mapped to mScarlet (right panel). The predicted integration site is shown as a vertical dashed line with soft-clipped reads (shown as coloured bases) to the left and right of the integration site. Above the sequenced reads are the basepair-level coverage histogram. The results indicate that mScarlet integrated only at the pcna locus genome wide as we could not detect mScarlet reads anchored anywhere else in the genome. Green (right)/turquoise (left) bars are of particular importance as they represent reads that span both mScarlet and the endogenous pcna locus. The red bars are anomalous mappings that either deviate from the expected insert size or paired-end mapping orientation. The one purple bar is flagged as a possible inter-chromosomal read between chromosomes 9 and 17. The mapping quality of this read is 0 which most likely means it is a false mapping. Coverage of mScarlet-pcna is 14.4×. (C) mNeonGreen integration in myosinhc locus: paired-end sequenced reads from mNG-myosinhc F1 embryos, coloured in grey for concordant mappings and coloured in dark grey/purple for inter-chromosomal paired-ends with one mate mapped to chr8 (left panel) and one mate mapped to mNeonGreen (right panel). The predicted integration site is shown as a vertical dashed line with soft-clipped reads (shown as coloured bases) to the left and right of the integration site. Above the sequenced reads are the basepair-level coverage histogram. Paired-end analysis yielded a second, weakly supported partial insertion of mNeonGreen at chr12:9083923 (not shown) which could not be confirmed by subsequent PCR, suggesting that this is a false-positive prediction or a rare insertion of very low frequency. The results argue that mNeonGreen integrated only at the myosinhc locus genome wide. Purple (right)/dark grey (left) bars are of particular importance as they represent reads that span both mNeonGreen and the endogenous myosinhc locus. The red bars are anomalous mappings that either deviate from the expected insert size or paired-end mapping orientation. Coverage of mNG-myosinhc is 14.5×.
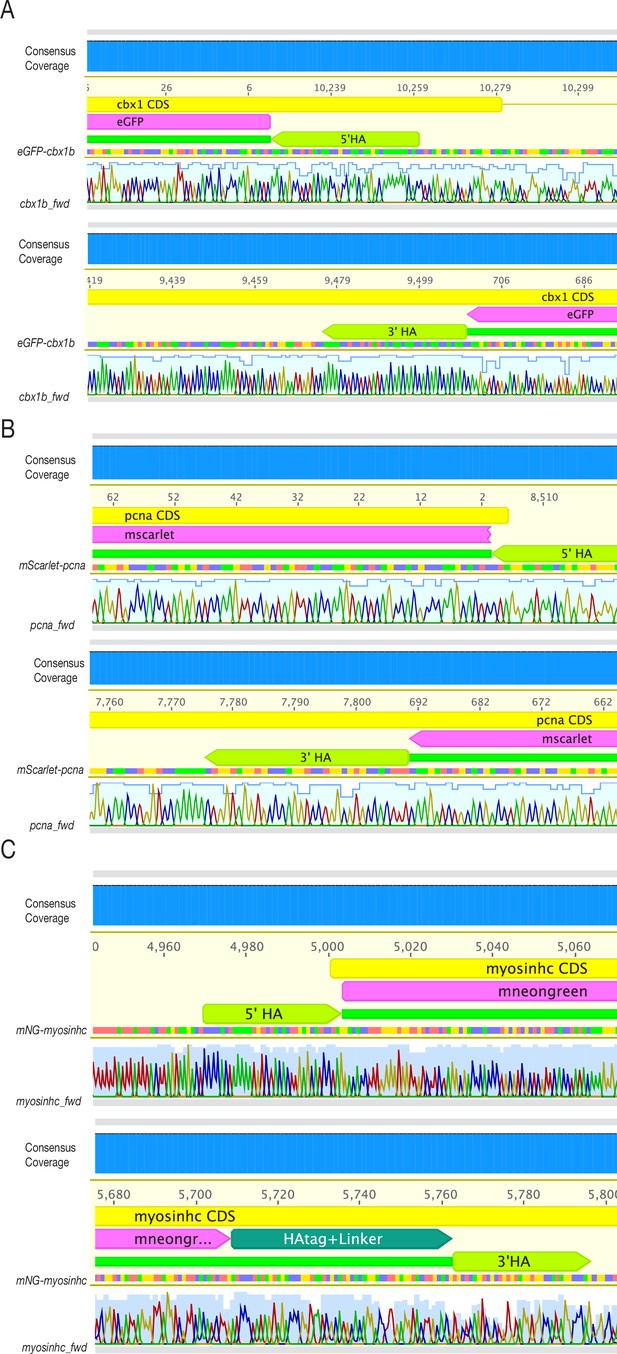
Sanger sequencing of F1 lines confirms single-copy in-frame fusion proteins.
(A) Sanger sequencing read from eGFP-cbx1b F1 fin-clipped adult shows scarless integration of eGFP into the cbx1b locus in both 5′ and 3′ junctions. Consensus coverage sequence is located at the top most panel in blue and grey. Any mismatch will be flagged by a black colour. There are no mismatches present. Blue line on top of the chromatograph peaks (A = green, T = red, C = blue, G = black) represent the quality of sequenced bases. (B) Sanger sequencing reads from mScarlet-pcna F1 fin-clipped adult show scarless integration of mScarlet into the pcna locus at the 3′ junction. While the 5′ junction shows correct in-frame fusion of endogenous pcna to mScarlet, we were able to detect a partial duplication of the 5′ homology arm with a small insertion that occurred 22 bp upstream of the start codon of endogenous pcna (for details see Materials and methods). Consensus coverage sequence is located at the top most panel in blue and grey. Any mismatch will be flagged by a black colour. There are no mismatches present. Blue line on top of the chromatograph peaks (A = green, T = red, C = blue, G = black) represents the quality of sequenced bases. (C) Sanger sequencing reads from mNG-myosinhc F1 fin-clipped adult show scarless integration of mNeonGreen into the myosinhc locus in both 5′ and 3′ junctions. Consensus coverage sequence is located at the top most panel in blue and grey. Any mismatch will be flagged by a black colour. There are no mismatches present. Blue line on top of the chromatograph peaks (A = green, T = red, C = blue, G = black) represents the quality of sequenced bases.
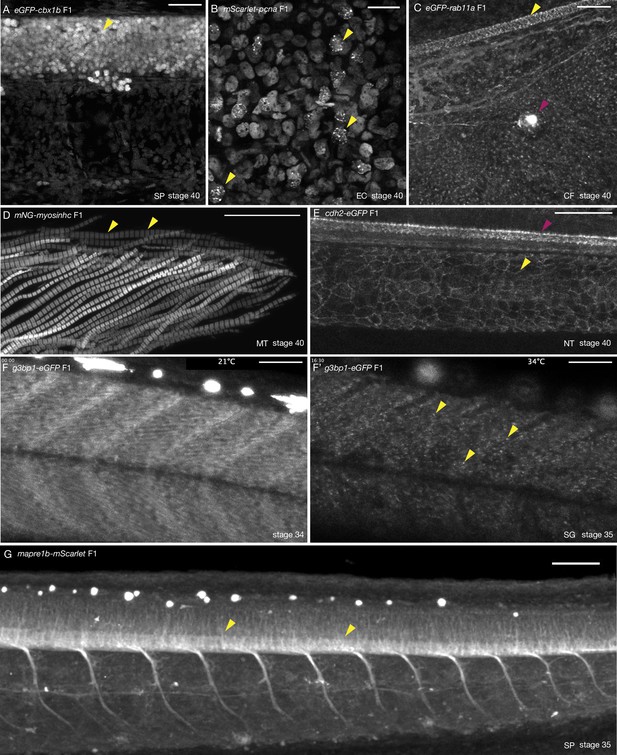
Tissue- and organelle-specific expression of seven CRISPR/Cas9 knock-in (KI) lines in medaka.
(A) eGFP-cbx1b F1 stage 40 medaka embryo. eGFP-Cbx1b labels all nuclei. Nuclei in the spinal cord of medaka are highlighted (yellow arrowhead). n > 10 embryos. SP = spinal cord. Scale bar = 30 µm. (B) mScarlet-pcna F1 stage 40 medaka embryo. mScarlet-Pcna is localized in the nuclei of cycling cells. mScarlet-Pcna is visible in skin epithelial cell nuclei located in the mid-trunk region of a medaka embryo. The localization of Pcna as speckles within the nucleus indicates cells in S phase of the cell cycle (yellow arrowheads). n = 10 embryos. EC = epithelial cells. Scale bar = 20 µm. (C) eGFP-rab11a F1 stage 40 medaka embryo. Expression of the membrane trafficking marker eGFP-Rab11a is evident in the caudal fin region. eGFP-Rab11a is strongly expressed in the spinal cord (yellow arrowhead) and lateral line neuromasts (magenta arrowhead). n = 6 embryos. CF = caudal fin. Scale bar = 30 µm. (D) mNG-myosinhc F1 stage 40 medaka embryo. mNG-Myosinhc is expressed solely in muscle cells. Myofibrils containing chains of individual sarcomere can be seen (mNG-Myosinhc labels the Myosin A band inside each sarcomere, yellow arrowheads). n > 10 embryos. MT = myotome. Scale bar = 30 µm. (E) cdh2-eGFP F1 stage 40 medaka embryo. Cdh2-eGFP is localized at cell membranes in several tissues, including the spinal cord (magenta arrowhead) and the notochord (yellow arrowhead). n = 5 embryos. NT = notochord. Scale bar = 50 µm. (F–F’) g3bp1-eGFP F1 stage 34–35 medaka embryo. Time-lapse imaging of G3bp1-eGFP dynamics under normal and stress conditions. (F) G3bp1-eGFP localizes to the cytoplasm under physiological conditions. (F’) Under stress conditions (temperature shock), G3bp1-eGFP localizes to stress granules (yellow arrowheads). Time in hours. n = 8 embryos. SG = stress granules. Scale bar = 50 µm. (G) mapre1b-mScarlet F1 stage 35 medaka embryo. Mapre1-mScarlet is expressed in a number of tissues and cell types including epithelial cells, muscle cells, the notochord, neuromasts and is highly expressed in the spinal cord. n = 5 embryos. SP = spinal cord. Scale bar = 50 µm.
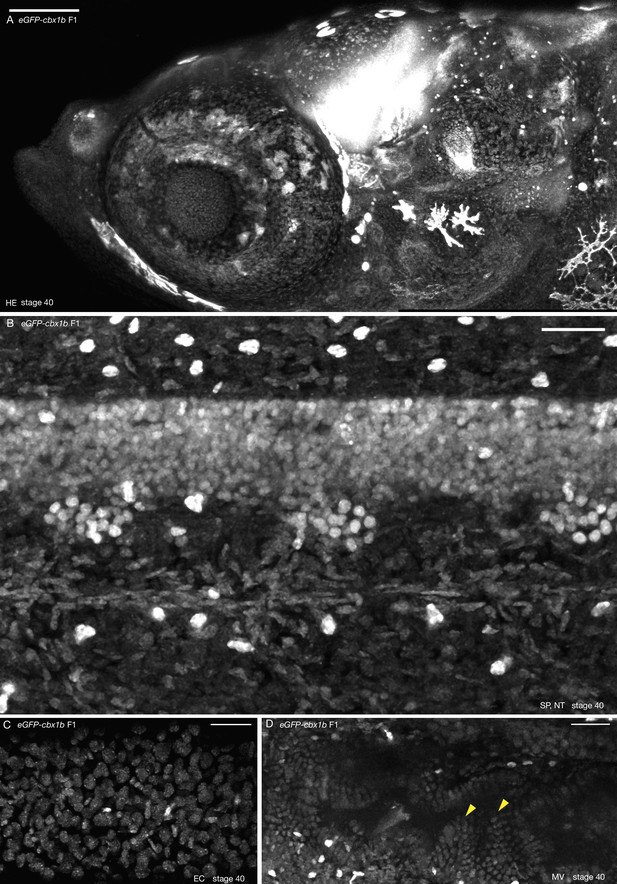
Ubiquitous nuclear expression of eGFP-cbx1b.
(A) Anterior head region of eGFP-cbx1b F1 stage 40 medaka embryo. eGFP-Cbx1b is expressed ubiquitously with a nuclear localization pattern. n > 10 embryos. HE = head. Scale bar = 100 µm. (B) Maximum projection of spinal cord and notochord in the mid-trunk region of eGFP-cbx1b stage 40 medaka embryo. All nuclei are labelled by eGFP-Cbx1b. n > 10 embryos. SP = spinal cord, NT = notochord. Scale bar = 30 µm. (C) A subset of epithelial cell nuclei positive labelled by eGFP-Cbx1b. n > 10 embryos. EC = epithelial cells. Scale bar = 30 µm. (D) Partial view of the gut of eGFP-cbx1b KI stage 40 medaka embryo. Gut microvilli are positive for eGFP-Cbx1b (yellow arrowheads). MV = microvilli. n > 10 embryos. Scale bar = 30 µm.
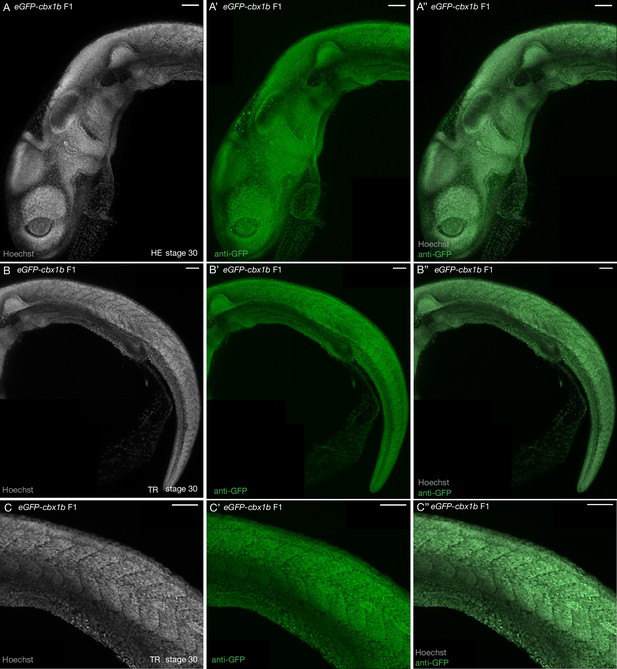
Immunofluorescence of eGFP-cbx1b line confirms ubiquitous nuclear expression.
(A) Maximum intensity projection of Hoechst stained stage 30 medaka embryo focusing on the anterior head region. (A’) Maximum intensity projection of anti-GFP staining in the anterior head region of the eGFP-cbx1b KI line. (A’’) Merged image from (A) and (A’). Note the overlap of Hoechst and the anti-eGFP staining in the eGFP-cbx1b KI line. n = 5 embryos. HE = head. Scale bar = 40 µm. (B) Maximum intensity projection of Hoechst stained stage 30 medaka embryo focusing on the posterior trunk region. (B’) Maximum intensity projection of anti-GFP staining in the posterior trunk region of the eGFP-cbx1b KI line. (B’’) Merged image from (B) and (B’). Note the overlap of Hoechst and the anti-eGFP staining in the eGFP-cbx1b KI line. n = 5 embryos. TR = trunk. Scale bar = 50 µm. (C–C’’) (C) Maximum intensity projection of Hoechst stained stage 30 medaka embryo, a zoomed-in view on a section of the trunk from (B–B’’) shows individual nuclei. (C’) Maximum intensity projection of anti-GFP staining on zoomed-in view of the trunk in the eGFP-cbx1b KI line. (C’’) Merged image from (C) and (C’’). Note the overlap of Hoechst and the anti-eGFP staining in nuclei. n = 5 embryos. TR = trunk. Scale bar = 50 µm.
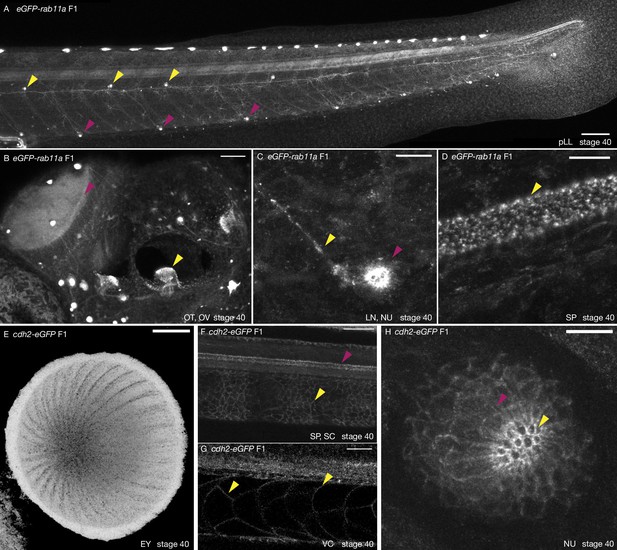
Tissue-specific expression of eGFP-rab11a and cdh2-eGFP KI lines.
(A) Neuromasts of eGFP-rab11a F1 stage 40 medaka embryo. eGFP-rab11a is strongly expressed in the posterior lateral line neuromasts and posterior lateral line nerve. Ventral primary neuromasts strongly express eGFP-Rab11a (magenta arrowheads), as well as secondary neuromasts located at the horizontal myoseptum (yellow arrowheads). n = 10 embryos. pLL = posterior lateral line. Scale bar = 100 µm. (B) Anterior region of eGFP-rab11a F1 stage 40 medaka embryo. eGFP-Rab11a is strongly expressed in the optic tectum (magenta arrowhead) and in the otic vesicle sensory organs (yellow arrowhead). n = 10 embryos. OT = optic tectum, OV = otic vesicle. Scale bar = 50 µm. (C) Close-up on a ventral primary neuromast of eGFP-rab11a F1 stage 40 medaka embryo. Strong expression of eGFP-Rab11a in neuromast cells (magenta arrowhead) and the lateral line nerve (yellow arrowhead). n = 10 embryos. LN = lateral line nerve, NU = neuromast. Scale bar = 10 µm. (D) Spinal cord of eGFP-rab11a F1 stage 40 medaka embryo. eGFP-Rab11a is strongly expressed in the spinal cord. n = 10 embryos. SP = spinal cord. Scale bar = 10 µm. (E) Eye of cdh2-eGFP F1 stage 40 medaka embryo. Cdh2-eGFP is strongly expressed in elongated cells covering the retina. n = 10 embryos. EY = eye. Scale bar = 30 µm. (F) Expression of cdh2-eGFP F1 in stage 40 medaka embryo. Cdh2-eGFP is expressed in the spinal cord (magenta arrowhead) and notochord sheath cells (yellow arrowhead). n = 10 embryos. SP = spinal cord, SC = sheath cells. Scale bar = 50 µm. (G) Notochord of cdh2-eGFP F1 stage 40 medaka embryo. Cdh2-eGFP is weakly expressed in notochord vacuolated cells (yellow arrowhead). The contrast has been enhanced in panel (G) compared to (F) to highlight better the expression in VCs. n = 10 embryos. VC = vacuolated cells. Scale bar = 30 µm. (H) Neuromast support and hair cells of cdh2-eGFP F1 stage 40 medaka embryo. Neuromast support and hair cells within a mature primary neuromast organ express high levels of Cdh2-eGFP. n = 10 embryos. NU = neuromast. Scale bar = 10 µm.
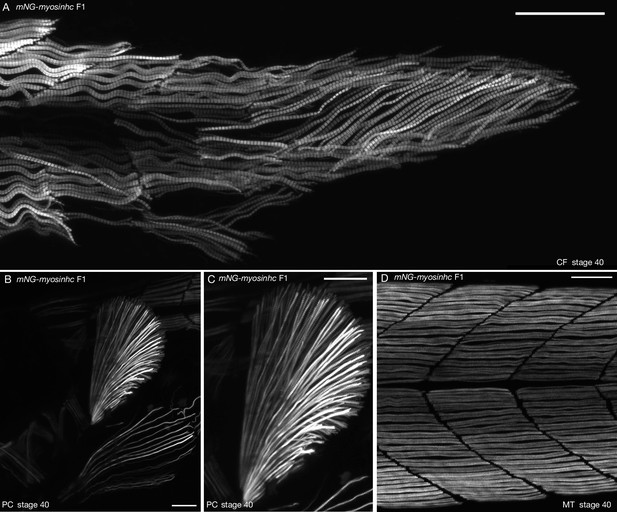
Muscle-specific expression of mNG-myosinhc.
(A) Muscle cells of mNG-myosinhc F1 stage 40 medaka embryo. mNG-Myosinhc is expressed in muscle cells of the posterior tail region and forms myofibrils, view of myofibrils from Figure 2D. n = 10 embryos. CF = caudal fin. Scale bar = 50 µm. Pectoral fin of mNG-myosinhc F1 stage 40 medaka embryo. mNG-Myosinhc is expressed in muscles of the pectoral fin, and myofibrils radiate from the base of the pectoral fin. (C) is a close-up of (B). n = 10 embryos. PC = pectoral fin. Scale bar = 50 µm. (D) Body trunk region of mNG-myosinhc F1 stage 40 medaka embryo. mNG-Myosinhc is expressed in muscle cells in the body trunk. Close-up of muscle cells from Figure 1D. n = 10 embryos. MT = myotome of trunk. Scale bar = 50 µm.
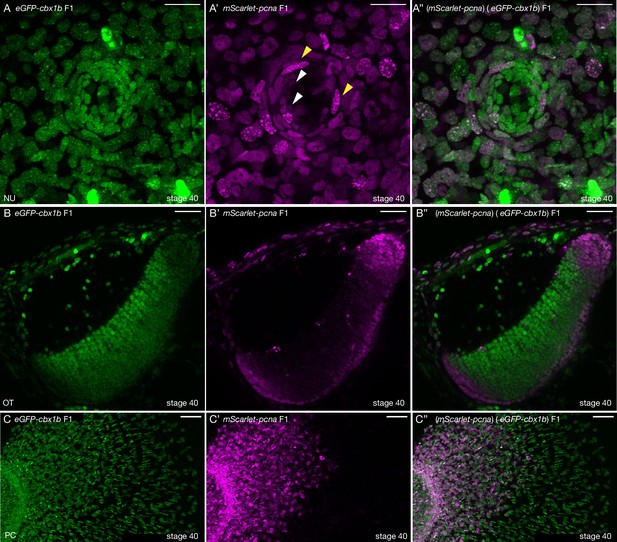
mScarlet-pcna line acts as an organismal-wide marker for proliferative zones.
(A–A’’) (eGFP-cbx1b) (mScarlet-pcna) double positive stage 40 medaka embryo. Maximum projection of a mature secondary neuromast (centre of image) within the lateral line system surrounded by epithelial cells, labelled by: endogenous eGFP-Cbx1b in (A) and endogenous mScarlet-Pcna in (A’). The merge is shown in (A’’). (A) eGFP-Cbx1b is a ubiquitous nuclear marker and labels all cell types within a mature neuromast. Those are: hair cells (HCs) in the centre of a neuromast, surrounded by support cells (SCs), and an outer ring of mantle cells (MCs) surrounded by the elongated neuromast border cells (nBCs). (A’) mScarlet-Pcna labels cycling cells, which are located at the very edge of the mature neuromast organ, a proportion of MCs express mScarlet-Pcna (white arrowheads). nBCs also express mScarlet-Pcna. Speckles can be seen in several mScarlet-Pcna-positive nBC nuclei (yellow arrowheads), indicating cells in late S phase of the cell cycle. (A’’) Merged image. n = 10 neuromast organs. NU = neuromast. Scale bar = 20 µm. (B–B’’) (eGFP-cbx1b) (mScarlet-pcna) stage 40 medaka embryo. Single Z-slice showing the medaka optic tectum. (B) eGFP-Cbx1b is a ubiquitous nuclear marker, (B’) whereas mScarlet-Pcna labels a subset of cells at the outer periphery of the optic tectum, indicating the position of proliferative cells in this tissue. A graded expression of mScarlet-Pcna is observed, with more central cells in the optic tectum losing the expression of mScarlet-Pcna. (B’’) Merged image. n = 4 embryos. OT = optic tectum. Scale bar = 30 µm. (C–C’’) Maximum projection of the pectoral fin of stage 40 medaka embryos. (C) eGFP-Cbx1b is a ubiquitous nuclear marker, (C’) while a subset of cells is labelled by mScarlet-Pcna indicating the position of proliferative cells. Note the proximal to distal gradient of mScarlet-Pcna expression, with proliferative cells at the base of the fin (left) and differentiated cells at the outer edges of the fin (right) (C’’) Merged image. n = 4 embryos. PC = pectoral fin. Scale bar = 50 µm.
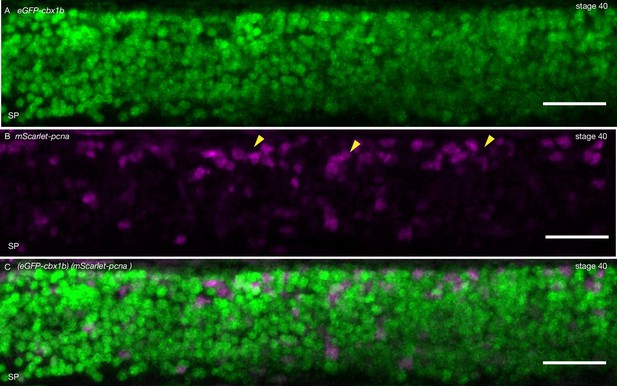
Proliferative cells in the anterior spinal cord.
(A) Maximum projection of the anterior spinal cord of an (eGFP-cbx1b) (mScarlet-pcna) double knock-in (KI) stage 40 medaka embryo. eGFP-Cbx1b (green) is expressed in all anterior spinal cord nuclei. n = 4 embryos. SP = spinal cord. Scale bar = 30 µm. (B) A subset of anterior spinal cord cells is positive for mScarlet-Pcna (magenta) indicating the existence of cycling cells. mScarlet-Pcna-positive cells (yellow arrowheads) are more clustered towards the dorsal side of the spinal cord. n = 4 embryos. SP = spinal cord. Scale bar = 30 µm. (C) Merged image of anterior spinal cord with eGFP-Cbx1b (green, A) and mScarlet-Pcna (magenta, B). n = 4 embryos. SP = spinal cord. Scale bar = 30 µm.
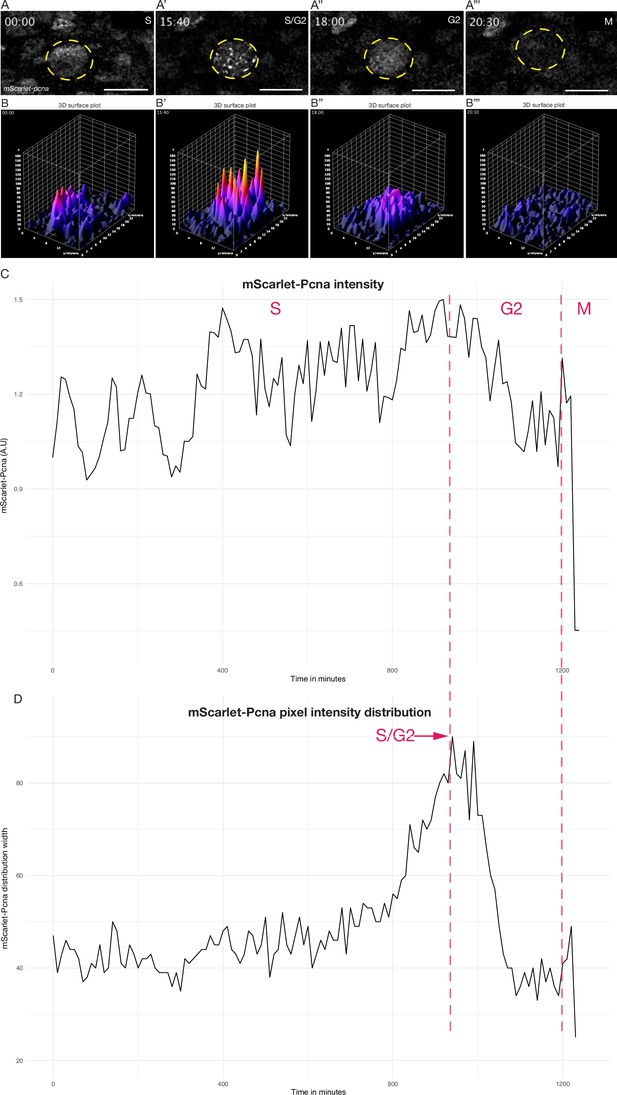
Quantitative live cell tracking of endogenous mScarlet-Pcna levels enables cell cycle phase classification.
(A–A’’’) Selected frames from a time-lapse imaging of a mScarlet-Pcna-positive skin epidermal cell nucleus (yellow circle) undergoing cell division. The different phases of the cell cycle are deduced from mScarlet-Pcna expression as highlighted within the panels. Late S phase can be distinguished by the presence of nuclear speckles that correspond to replication foci. n = 9 skin epithelial cells. Time in hours. (B–B’’’) 3D surface plots of cell from (A). The S/G2 transition is marked as the point of peak pixel intensity distribution within the nucleus, which is reached at 15:40 hr (B’) and is equivalent to the largest width of mScarlet-Pcna pixel intensity distribution shown in panel D (red arrow). n = 9 skin epidermal cells. (C) Normalized mScarlet-Pcna intensity level within the nucleus from cell in (A–A’’’) over the course of one cell division. Vertical dashed red lines demarcate the different cell cycle phases based on the intensity and distribution of mScarlet-Pcna within the nucleus. Initially, an increase of endogenous mScarlet-Pcna expression over time indicates cells in S phase of the cell cycle. M phase is characterized by a sharp drop in nuclear mScarlet-Pcna levels beginning at 1200 min. (D) Width of pixel intensity distribution over time on cell from (A–A’’’). The S/G2 transition is marked as the point of peak pixel intensity distribution within the nucleus, which is reached at 940 min and is equivalent to the largest width of mScarlet-Pcna pixel intensity distribution (red arrow). n = 9 skin epidermal cells.
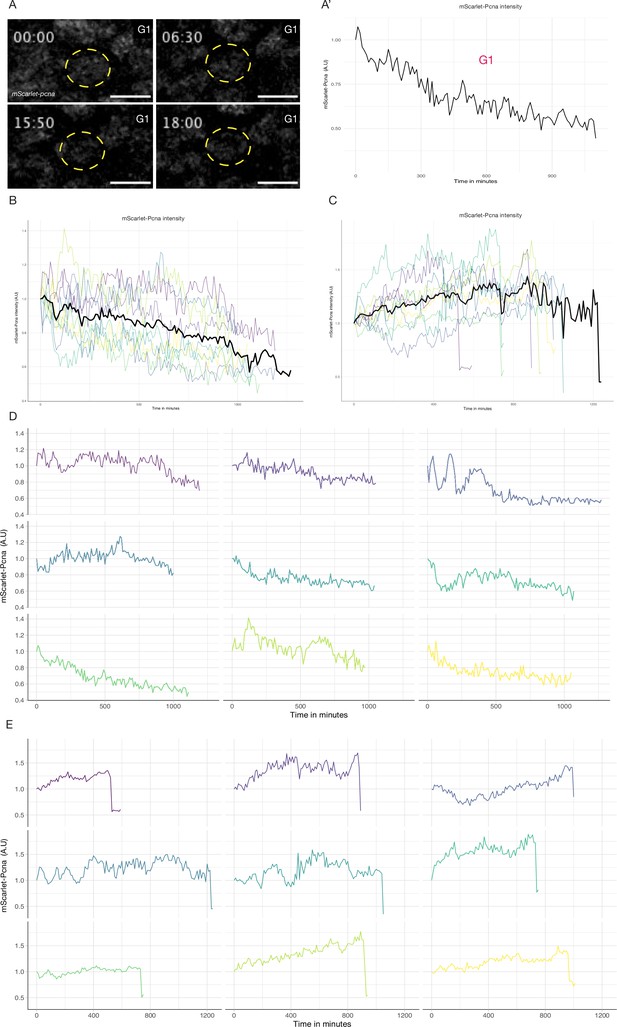
Quantification of endogenous mScarlet-pcna levels utilized for cell cycle phase classification.
(A–A’) Selected frames from a time-lapse of a mScarlet-Pcna-positive epithelial cell nucleus that did not undergo cell division over the course of the time-lapse (A, yellow dashed circle). Time in hours. Quantification of normalized mScarlet-Pcna levels shows a decrease of endogenous expression over time indicative of cells in the G1 phase of the cell cycle (A’, n = 9 cells). (B) Tracking of endogenous mScarlet-Pcna dynamics in nine epithelial cell nuclei that do not undergo cell division over the course of the time-lapse (black line = mean). Endogenous mScarlet-Pcna levels decrease over time indicative of cells in the G1 phase of the cell cycle. (C) Tracking of endogenous mScarlet-Pcna dynamics in nine epithelial cell nuclei that undergo cellular division (black line = mean). Endogenous mScarlet-Pcna levels increase over time, indicative of cells in the S phase of the cell cycle. (D) Individual traces of normalized mScarlet-Pcna expression in nine epithelial cells shown in (B). The tracked cells did not divide over the course of the time-lapse. Normalized intensity of mScarlet-Pcna decreases over time indicative of cells in the G1 phase of the cell cycle. (E) Individual traces of normalized mScarlet-Pcna expression in nine epithelial cells shown in (C). The tracked cells underwent a cell division over the course of the time-lapse. Normalized intensity of mScarlet-Pcna increases over time indicative of cells in the S phase of the cell cycle. The beginning of the sharp drop in endogenous mScarlet-Pcna intensity marks entry into M phase.
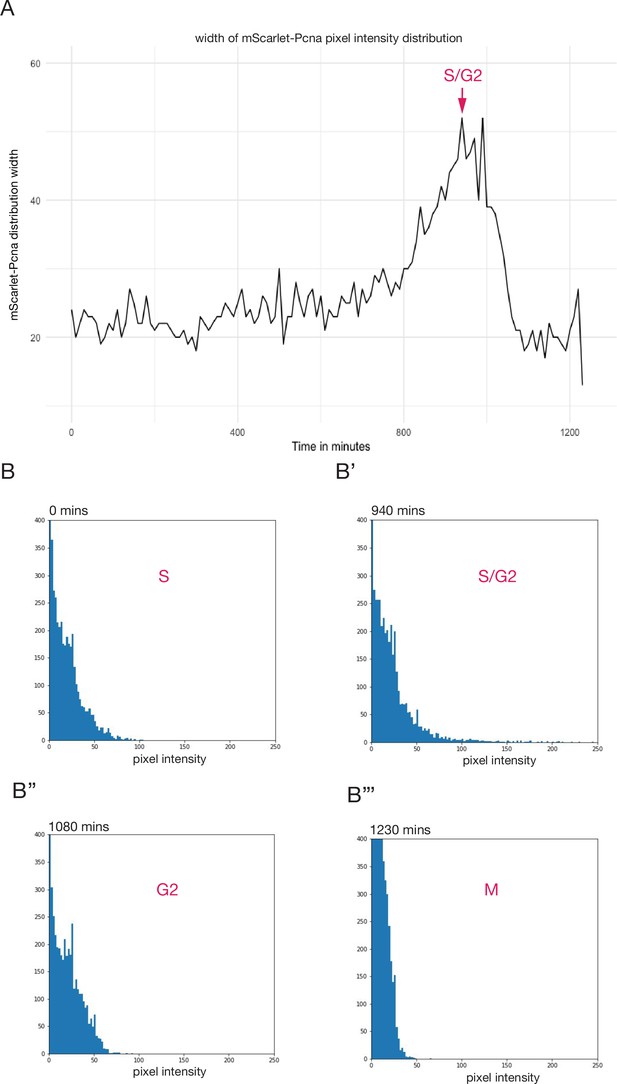
mScarlet-pcna histograms of pixel intensity distribution during cell cycle progression.
(A) Width of mScarlet-Pnca pixel intensity over the course of one cell cycle, the S/G2 transition corresponds to the point of peak pixel intensity distribution within the nucleus and is marked by a red arrow. Data from Figure 4. (B–B’’’) Histograms of pixel intensity distribution within the nucleus of the same cell shown in Figure 4A,B over the course of one cell cycle. The different phases of the cell cycle are marked in red based on the combination of endogenous intensity profiles, 3D surface plots, and histograms of pixel intensity distributions. The S/G2 transition is reached at 940 min and marks the point of peak pixel intensity distribution within the nucleus. M phase is marked by a sharp drop in endogenous mScarlet-Pcna expression. Time in minutes. n = 9 epithelial cells.
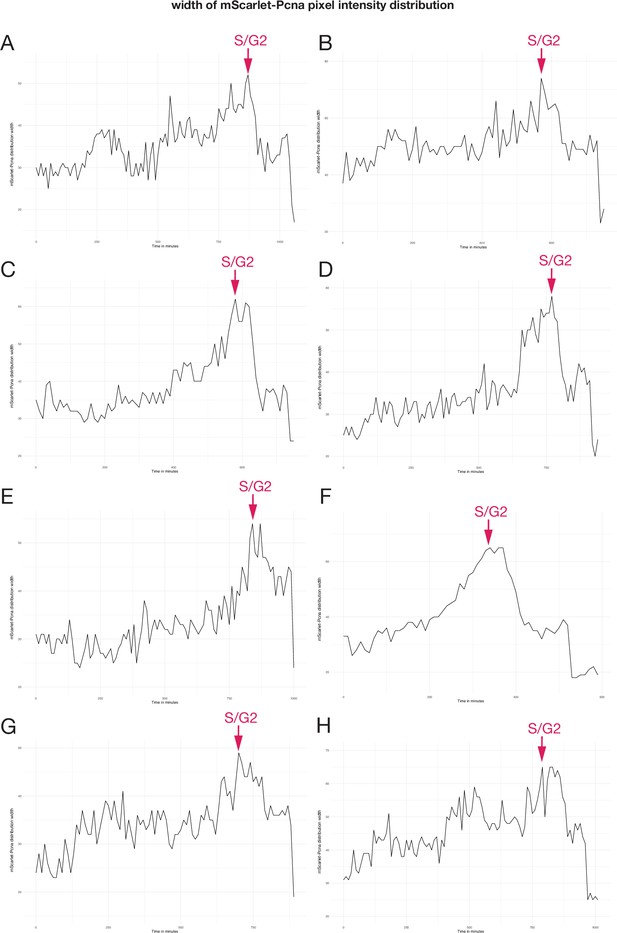
mScarlet-pcna histograms of pixel intensity distribution from eight cells.
(A–H) Width of mScarlet-Pnca pixel intensity over the course of one cell cycle, the S/G2 transition (red arrow) corresponds to the point of peak pixel intensity distribution within the nucleus and is marked by a red arrow. Data from eight individual epithelial cells. Time in minutes.
Videos
Z-stack through the caudal fin region of a stage 39–40 cbx1-eGFP medaka embryo.
eGFP-Cbx1b is expressed in all nuclei of the different cell types in the caudal fin region. n > 10 embryos. Scale bar = 30 µm.
Live imaging in the caudal fin region of a stage 39–40 eGFP-rab11a medaka embryo.
eGFP-Rab11a is an intra-cellular trafficking marker and localizes to intra-cellular vesicles. Notice the dynamics of vesicle trafficking in epithelial cells, neuromasts (magenta arrowhead), and peripheral lateral line nerve (yellow arrowhead). Time in minutes. n = 4 embryos. Scale bar = 10 µm.
Live imaging of skin epithelial cells in the mid-trunk region of a stage 39–40 eGFP-rab11a medaka embryo.
On the left panel is a merged view of epithelial cells in bright-field and eGFP-Rab11a in green. On the right panel, eGFP-Rab11a in grey scale. eGFP-Rab11a vesicles appear as granules within the cytoplasm of epithelial cells. Time in minutes. n = 4 embryos. Scale bar = 10 µm.
Z-stack through the caudal fin region of a stage 39–40 eGFP-rab11a medaka embryo.
eGFP-Rab11a is strongly expressed in the caudal neuromast and peripheral lateral line nerve. And is also expressed in epithelial cells, the notochord, and the spinal cord. n = 4 embryos. Scale bar = 30 µm.
Live imaging of stage 34–35 g3bp1-eGFP under normal conditions (temperature 21°C) reveals the cytoplasmic localization of G3bp1-eGFP in epithelial and muscle cells in the mid-trunk region of medaka embryos.
Upon stress conditions (temperature shift to 34°C, 60 min after the beginning of the time-lapse), G3bp1-eGFP localization begins to shift into localized clusters of stress granule puncta. Time in hours. n = 8 embryos. Scale bar = 50 µm.
Live imaging of stage 34 mNG-myosinhc medaka embryo during muscle formation.
Muscle cell growth is driven by local buckling of individual muscle cells. Muscle growth and expression of mNG-Myosinhc does not seem to be polarized in an anterior–posterior or dorsal–ventral axis. Instead muscle cells have a heterogenous expression of mNG-Myosinhc that increases as muscle cells grow in length and mature. Time in hours. n = 9 embryos. Scale bar = 50 µm.
Z-stack through the posterior trunk region of a stage 39–40 cdh2-eGFP medaka embryo.
Cdh2-eGFP is expressed on the cellular membranes of neuronal tissue including neuromasts, the spinal cord, and the notochord. n = 3 embryos. Scale bar = 50 µm.
Live imaging of the dorsal side of a cdh2-eGFP medaka embryo at the 12-somite stage reveals the endogenous dynamics of Cdh2-eGFP at high temporal resolution.
Time in minutes. n = 2. Scale bar = 30 µm.
Z-stack through the trunk region of a stage 35 mapre1b-mScarlet medaka embryo.
Mapre1b-mScarlet is expressed in a number of tissues including epithelial cells, muscle cells, the notochord, neuromasts and is highly expressed in the spinal cord. n = 5 embryos. Scale bar = 100 µm.
Live imaging of stage 35 mapre1b-mScarlet medaka embryo.
A close-up view on the developing spinal cord. Microtubule dynamics can be observed within the spinal cord (yellow arrowhead). Time in minutes. n = 5 embryos. Scale bar = 10 µm.
Live imaging of stage 39–40 mScarlet-Pcna-positive non-dividing epithelial cell nucleus (shown in Figure 4—figure supplement 1).
Intensity profiles were extracted from within the nucleus (yellow circle). The cell does not divide over the course of the time-lapse. A decrease of mScarlet-Pcna levels over time is indicative of cells in the G1 phase of the cell cycle. Time in hours. n = 9 mScarlet-Pcna-positive non-dividing epithelial cells. Scale bar = 5 µm.
Live imaging of stage 39–40 mScarlet-Pcna-positive epithelial cell nucleus undergoing cell division (shown in Figure 4).
Intensity profiles were extracted from within the nucleus (yellow circle). An increase of mScarlet-Pcna levels over time is indicative of cells in the S phase of the cell cycle. The appearance of nuclear speckles is indicative of cells in late S phase. The S/G2 transition is marked as the point of peak pixel intensity distribution within the nucleus (yellow circle). The sharp drop of endogenous mScarlet-Pcna levels is indicative of cells in M phase. Time in hours. n = 9 mScarlet-Pcna-positive and dividing epithelial cells. Scale bar = 15 µm.
Left panel: mScarlet-Pcna-positive epithelial cell nucleus undergoing cell division (shown in Figure 4).
Middle panel: histogram of pixel intensity distribution of mScarlet-Pcna within the nucleus of the tracked cell. Right panel: frequency distribution width obtained from the histogram of pixel intensity distribution. Peak pixel intensity distribution is reached at 15:40 hr and is used to mark the S/G2 transition. Time in hours. n = 9 dividing epithelial cells. Scale bar = 15 µm.
Left panel: 3D surface plot of mScarlet-Pcna-positive epithelial cell nucleus undergoing cell division (from Figure 4).
Middle panel: normalized endogenous mScarlet-Pcna levels of epithelial cell (from Figure 4). Right panel: histogram of pixel intensity distribution. Time in hours. n = 9 epithelial cells that undergo cell division.
Tables
Reagent type (species) or resource | Designation | Source or reference | Identifiers | Additional information |
---|---|---|---|---|
Strain, strain background (Oryzias latipes) | Cab | Other | Medaka Southern wild-type population | |
Strain, strain background (Oryzias latipes) | eGFP-cbx1b | This paper | CRISPR KI line, Aulehla Lab EMBL Heidelberg | |
Strain, strain background (Oryzias latipes) | mGL-cbx1b | This paper | CRISPR KI line, Aulehla Lab EMBL Heidelberg | |
Strain, strain background (Oryzias latipes) | cdh2-eGFP | This paper | CRISPR KI line, Aulehla Lab EMBL Heidelberg | |
Strain, strain background (Oryzias latipes) | g3bp1-eGFP | This paper | CRISPR KI line, Aulehla Lab EMBL Heidelberg | |
Strain, strain background (Oryzias latipes) | mapre1b-linker-3XFlag-mScarlet | This paper | CRISPR KI line, Aulehla Lab EMBL Heidelberg | |
Strain, strain background (Oryzias latipes) | mNG-HAtag-Linker-myosinhc | This paper | CRISPR KI line, Aulehla Lab EMBL Heidelberg | |
Strain, strain background (Oryzias latipes) | mScarlet-pcna | This paper | CRISPR KI line, Aulehla Lab EMBL Heidelberg | |
Strain, strain background (Oryzias latipes) | eGFP-rab11a | This paper | CRISPR KI line, Aulehla Lab EMBL Heidelberg | |
Strain, strain background (Oryzias latipes) | (eGFP-cbx1b) × (mScarlet-pcna) | This paper | CRISPR KI line, Aulehla Lab EMBL Heidelberg | |
Antibody | Primary rabbit anti-GFP (polyclonal) | TorreyPines Biolabs | #TP401 | 1:500 |
Antibody | Secondary goat anti-rabbit | Abcam AlexaFluor 488 | #ab150077 | 1:500 |
Recombinant DNA reagent | Cas9-mSA plasmid | PMID:29889212 | ||
Recombinant DNA reagent | Cas9 no-mSA plasmid | PMID:29889212 | ||
Recombinant DNA reagent | Cas9 plasmid | PMID:24873830 | ||
Sequence-based reagent | gRNA actb | Sigma | Sequence (spacer)GGAUGAUGACAUUGCCGCAC | |
Sequence-based reagent | gRNA cbx1b | Sigma | Sequence (spacer)GGAAGAUGUGGCAGAAGAAG | |
Sequence-based reagent | gRNA cdh2 | Sigma | Sequence (spacer)GGGAGCGAUGACUAAGACAA | |
Sequence-based reagent | gRNA g3bp1 | Sigma | Sequence (spacer)CCCCAGCGAGAGCCGCUUCU | |
Sequence-based reagent | gRNA mapre1b | Sigma | Sequence (spacer)UCCAGAUGCUGAGGAACAGG | |
Sequence-based reagent | gRNA myosinhc | Sigma | Sequence (spacer)CAUCUCUGCGUCAGUGCUCA | |
Sequence-based reagent | gRNA pcna | Sigma | Sequence (spacer)GACCAGGCGAGCCUCAAACA | |
Sequence-based reagent | gRNA rab11a | Sigma | Sequence (spacer)UCGGAUUAACGCGAGGACGA | |
Commercial assay or kit | RNAeasyMiniKit | Qiagen | #74104 | |
Commercial assay or kit | QIAquickGel Extraction Kit | Qiagen | #28115 | |
Commercial assay or kit | mMachineSP6 Transcription Kit | Invitrogen | #AM1340 | |
Software, algorithm | CC-Top | PMID:25909470 | ||
Software, algorithm | Ensembl | Public | ||
Chemical compound, drug | Hoechst 33342 | Thermo Fischer | #H3570 | 1:500 dilution of the 10 mg/ml stock solution |
Chemical compound, drug | Tricane | Sigma-Aldrich | #A5040-25G | |
Chemical compound, drug | LMP Agarose | Biozyme | Plaque Agarose #840,101 | 0.6% in 1× H2O |
Additional files
-
Supplementary file 1
Detailed protocol for cloning-free CRISPR/Cas9 Knock-In of fluorescent reporters in medaka.
- https://cdn.elifesciences.org/articles/75050/elife-75050-supp1-v3.docx
-
Supplementary file 2
Detailed sequence design for mNeonGreen-HAtag-Linker-myosinhc tagging.
- https://cdn.elifesciences.org/articles/75050/elife-75050-supp2-v3.docx
-
Supplementary file 3
Details of targeted loci and sequences for all KI lines.
(a) Details of targeted loci. (b) PCR repair donors used in this study. (c) PCR Primers used in this study. (d) Plasmids used in this study. (e) sgRNAs used in this study. (f) Medaka lines generated and maintained in this study. (g) Comparison of Cas9 mRNAs and PCR repair donors.
- https://cdn.elifesciences.org/articles/75050/elife-75050-supp3-v3.xlsx
-
Supplementary file 4
Quantification of targeting efficiency.
- https://cdn.elifesciences.org/articles/75050/elife-75050-supp4-v3.xlsx
-
Transparent reporting form
- https://cdn.elifesciences.org/articles/75050/elife-75050-transrepform1-v3.docx
-
Source code 1
Histograms for pixel intensity distribution.
- https://cdn.elifesciences.org/articles/75050/elife-75050-supp5-v3.zip