Chronic Ca2+ imaging of cortical neurons with long-term expression of GCaMP-X
Figures
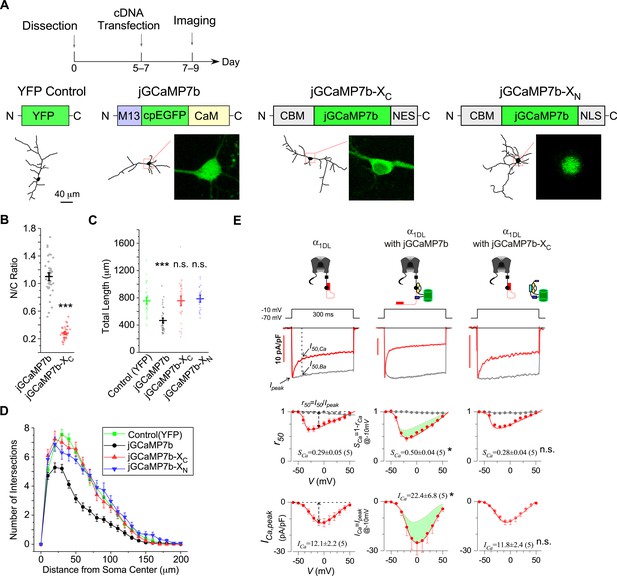
The design principles applicable to jGCaMP7 and jGCaMP7-X.
(A) Cultured cortical neurons from newborn mice were transiently transfected with YFP, jGCaMP7b, jGCaMP7b-XC, or jGCaMP7b-XN, respectively, on DIV 5–7, then imaged by confocal microscopy on DIV 7–9. As illustrated, apoCaM-binding motif (CBM) was fused onto N-terminus of GCaMP and the tags of localization signals (nuclear export signal or nuclear localization signal, NES/NLS) were fused to the C-terminus of GCaMP to construct GCaMP-XC or GCaMP-XN, respectively. Neurite tracing and subcellular GCaMP distributions are shown below. (B) N/C ratio of jGCaMP7b or jGCaMP7b-XC in neurons, by calculating the nucleus versus cytosol ratio of fluorescence intensities. Total length (C) and Sholl analysis (D) for cortical neurons expressing YFP or GCaMP variants. Two independent experiments from two independent culture preparations (A–D). (E) Electrophysiological validations of jGCaMP7b versus jGCaMP7b-XC with recombinant CaV1.3 channels. Full-length CaV1.3 channels (α1DL) were expressed in HEK293 cells alone (left) or with jCaMP7b (middle) or with jGCaMP7b-XC (right). At −10 mV, Ca2+ current traces (red, with scale bars indicating current amplitudes) and Ba2+ current traces (gray, rescaled) are shown. SCa and ICa (quantified by the equations shown in the first column) are the indices of calcium-dependent inactivation and voltage-dependent activation, respectively. Cell numbers are indicated in the parentheses right after the values. Standard error of the mean (SEM) and two-tailed unpaired Student’s t-test (B) or one-way analysis of variance (ANOVA) followed by Bonferroni for post hoc tests (C, E) (criteria of significance: *p < 0.05; ***p < 0.001; n.s. denotes ‘not significant’) were applied.
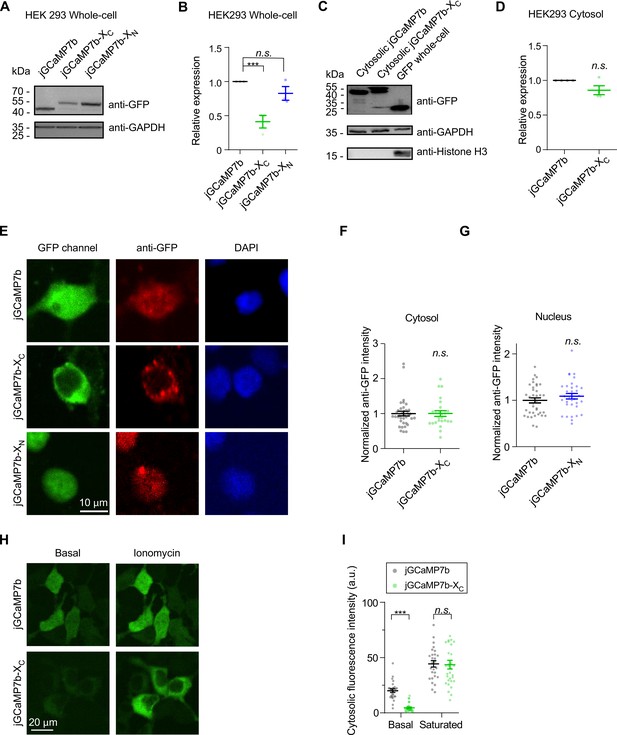
Expression levels of indicators in HEK293 cells.
(A–D) jGCaMP7b, jGCaMP7b-XC, and jGCaMP7b-XN were transiently transfected into HEK293 cells to examine the level of protein expression by western blot. Whole-cell extracts (A, B) and cytosolic extracts (C, D) were separately examined. The anti-GFP bands and anti-GAPDH bands indicate the expression levels and the amount of inputs, respectively. The anti-Histone H3 bands served as the nuclear marker to confirm the efficacy of the fractionation. The relative expression levels were normalized to the jGCaMP7b control for the whole cell (B) or cytosol (D) proteins. (E–G) Comparison of protein expression of indicators in HEK293 cells by immunostaining. HEK cells expressing jGCaMP7b, jGCaMP7b-XC, and jGCaMP7b-XN were stained with anti-GFP (red channel) and DAPI (4′,6-diamidino-2-phenylindole, blue channel) to measure the level of expression and illustrate the nuclear envelop, respectively. Confocal images in GFP channels indicate GCaMP fluorescence (E). The anti-GFP signals were normalized over jGCaMP7b to compare the expression levels. The cytosolic and nuclear levels of jGCaMP7b were compared with jGCaMP7b-XC (F) and jGCaMP7b-XN (G), respectively. (H, I) HEK293 cells expressing jGCaMP7b or jGCaMP7b-XC were stimulated by 2.5 μM ionomycin (a potent calcium ionophore) to saturate the indicators (H). Indexed by cytosolic fluorescence intensity, the two indicators were compared at the basal or saturated state (I), suggesting that it is possible to use probe fluorescence to estimate the expression level if the conditions are carefully controlled. Three independent experiments from three independent samples. Standard error of the mean (SEM) and one-way analysis of variance (ANOVA) followed by Bonferroni for post hoc tests (B) or two-tailed unpaired Student’s t-test (D, F, G, I) (criteria of significance: ***p < 0.001; n.s. represents ‘not significant’) were applied.
-
Figure 1—figure supplement 1—source data 1
Source data for Figure 1—figure supplement 1A.
Original uncropped western blotting gels with indication of the cropped areas.
- https://cdn.elifesciences.org/articles/76691/elife-76691-fig1-figsupp1-data1-v2.zip
-
Figure 1—figure supplement 1—source data 2
Source data for Figure 1—figure supplement 1C.
Original uncropped western blotting gels with indication of the cropped areas.
- https://cdn.elifesciences.org/articles/76691/elife-76691-fig1-figsupp1-data2-v2.zip

Expression levels of indicators in cultured cortical neurons.
(A–C) The indicators of jGCaMP7b, jGCaMP7b-XC, and jGCaMP7b-XN expressed in cultured cortical neurons were examined by immunostaining. Similar to HEK293 cells, cultured cortical neurons from newborn mice transiently transfected by jGCaMP7b, jGCaMP7b-XC, or jGCaMP7b-XN were stained by anti-GFP (red channel) and DAPI (blue channel) to measure the protein expression and illustrate the nuclear envelop, respectively. Confocal images in GFP channels pointed out the positive neurons. The anti-GFP signals were normalized over the jGCaMP7b groups. The cytosolic and nuclear levels of jGCaMP7b were compared with jGCaMP7b-XC (B) and jGCaMP7b-XN (C), respectively. (D, E) Cortical neurons expressing jGCaMP7b or jGCaMP7b-XC were stimulated by 2.5 μM ionomycin (D). Indexed by cytosolic fluorescence intensity, the two indicators were compared at the basal or saturated state (E). (F–H) jGCaMP7b-XC and jGCaMP7b-XN were cotransfected into cortical neurons to compare with jGCaMP7b. Cultured cortical neurons expressing YFP served as the control. Anti-GFP and DAPI fluorescence images indicate the protein expression and the nuclear envelop, respectively (F). The total length of neurites was calculated and compared for the three groups of neurons (G). Indexed by normalized anti-GFP intensity (over jGCaMP7b) cells coexpressing jGCaMP7b-XC and jGCaMP7b-XN resulted in an even higher level of probe expression than jGCaMP7b. Three independent experiments from three independent culture preparations. Standard error of the mean (SEM) and two-tailed unpaired Student’s t-test (B, C, E, H) or one-way analysis of variance (ANOVA) followed by Bonferroni for post hoc tests (G) (criteria of significance: *p < 0.05, **p < 0.01, ***p < 0.001; n.s. represents ‘not significant’) were applied.
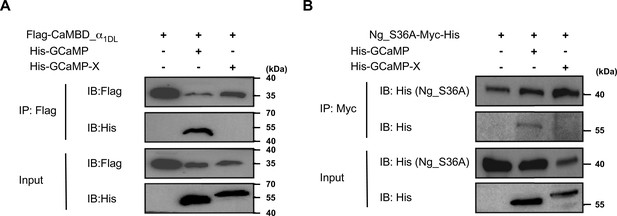
Differential interactions of GCaMP versus GCaMP-X with apoCaM-binding proteins.
Coimmunoprecipitation results of His-tagged GCaMP or GCaMP-XC with Flag-tagged apoCaM-binding domain of α1DL (CaMBD_α1DL) (A), or with Myc- and His-tagged YFP-Ng_S36A (B). HEK293 cell lysates were added with 5 mM EGTA; n = 3 replicates.
-
Figure 1—figure supplement 3—source data 1
Source data for Figure 1—figure supplement 3A, B.
Original uncropped western blotting gels with indication of the cropped areas.
- https://cdn.elifesciences.org/articles/76691/elife-76691-fig1-figsupp3-data1-v2.zip
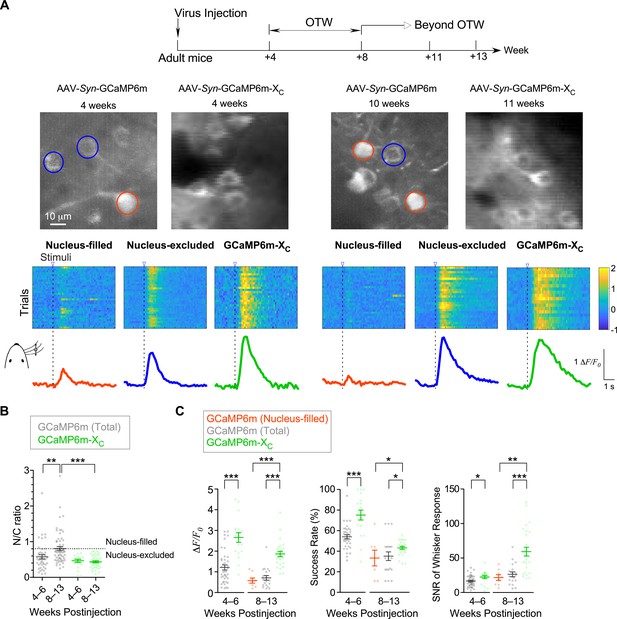
In vivo Ca2+ imaging of sensory-evoked responses in cortical neurons virally infected with GCaMP-X versus GCaMP.
(A) Ca2+ dynamics of GCaMP6m and GCaMP6m-XC in S1 primary somatosensory cortex under whisker stimulation by in vivo two-photon Ca2+ imaging. 4–6 and 8–13 weeks postinjection were considered as optimal time window (OTW) or beyond OTW, respectively. The orange and blue circles in the representative two-photon images indicate nucleus-filled and nucleus-excluded GCaMP6m, respectively. The colored scale bar indicates the fluorescence intensity of Ca2+ probes. Multiple trials of the same neuron are shown for nucleus-filled GCaMP6m, nucleus-excluded GCaMP6m, or GCaMP6m-XC. The averaged Ca2+ responses are shown at the bottom. (B) Subcellular distributions of GCaMP6m and GCaMP6m-XC in vivo within OTW or beyond OTW. Neurons were divided into two distinct subgroups, nucleus-excluded and nucleus-filled, by applying the cutoff value (N/C ratio) of 0.8. (C) Responses to repetitive whisker stimuli were evaluated and compared for GCaMP6m nucleus-filled neurons, total GCaMP6m neurons, and GCaMP6m-XC. The average amplitude (ΔF/F0) (left), success rate of the trials (middle) and SNR (right) during whisker stimuli were compared. Data were obtained from five or four mice for GCaMP6m and GCaMP6m-XC, respectively. Standard error of the mean (SEM) and one-way analysis of variance (ANOVA) followed by Bonferroni for post hoc tests (criteria of significance: *p < 0.05; **p < 0.01; ***p < 0.001) were calculated when applicable.
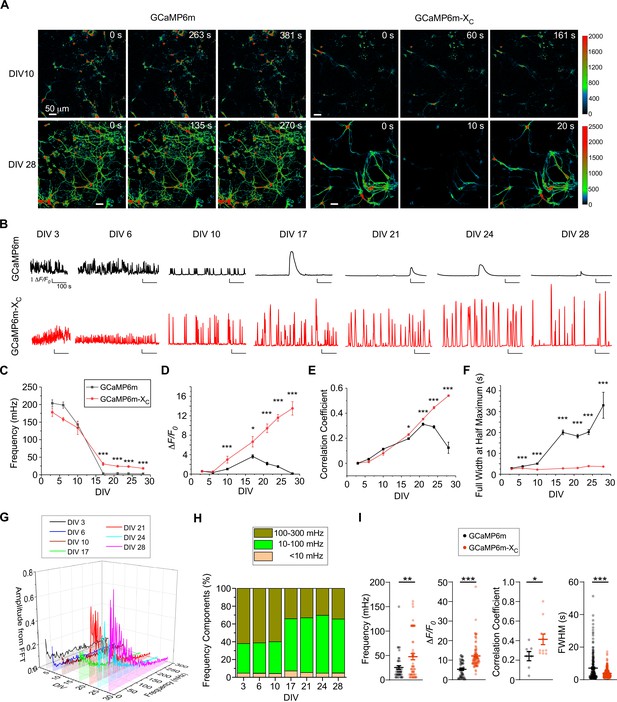
Chronic Ca2+ fluorescence imaging for autonomous Ca2+ oscillations in cultured cortical neurons.
(A) Time-lapse images of cultured cortical neurons infected with AAV-Syn-GCaMP6m or AAV-Syn-GCaMP6m-XC. Spontaneous Ca2+ activities by the two probes are shown for DIV 10 and DIV 28. See Figure 3—videos 1–3 for details. Ca2+ signals (color coded) were monitored by the confocal microscope with a live-cell imaging chamber to maintain the cell culture conditions (37°C, 5% CO2, 97–100% humidity) at different timepoints. (B) Representative traces of Ca2+ activities in cultured cortical neurons expressing GCaMP6m (upper) or GCaMP6m-XC (lower) from DIV 3 to DIV 28. Temporal profiles of key indices measured from spontaneous Ca2+, including the average frequency (10–3 Hz or mHz) (C) and peak amplitude (ΔF/F0, D); synchrony (quantified by the mean of correlation coefficient per view, E) and full width at half maximum (FWHM, F). (G) Power spectral analyses by FFT (Fast Fourier Transformation) for Ca2+ traces of cortical neurons from DIV 3 to DIV 28. (H) Frequency components in percentage. By integrating the absolute amplitudes over each frequency band (G), three major bands are shown:<10 mHz (ultra-slow), 10–100 mHz (slow), and 100–300 mHz (fast), where the 10–100 mHz band indicates the major component. (I) Summary over three independent experiments with three independent culture preparations (see Figure 3—figure supplement 2 for the other two experiments). Key indices of frequency (DIV 35), ΔF/F0 (DIV 28), correlation coefficient per view (DIV 28) and FWHM (DIV 28) were calculated and compared for GCaMP6m versus GCaMP6m-XC. Standard error of the mean (SEM) and the Student’s t-test (two-tailed unpaired with criteria of significance: *p < 0.05; **p < 0.01; ***p < 0.001) were calculated when applicable.
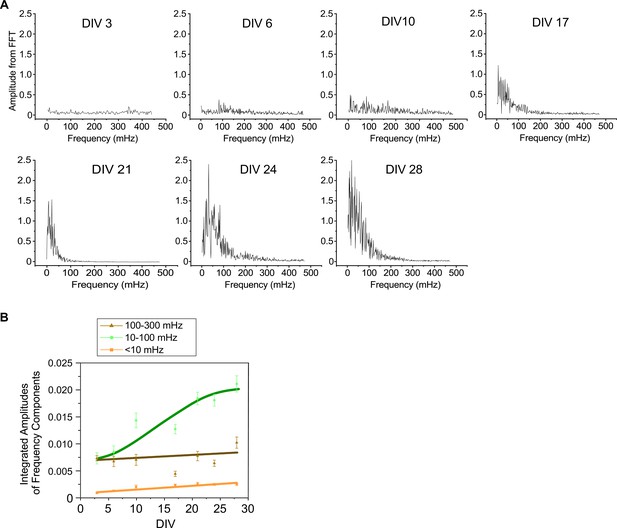
Spectral analysis for Ca2+ waveforms acquired by GCaMP-X.
(A) Representative FFT-based power spectra of individual neurons at different timepoints (DIV). Neurons from DIV 3 to DIV 10 exhibited low FFT amplitudes, whereas neurons from DIV 21 to DIV 28 showed significantly large amplitudes with the central frequency around 10–100 mHz. DIV 17 appeared to be the transition time for neurons to develop from weak oscillations (DIV 3 to DIV 10) to strong oscillations (DIV 21 to DIV 28). (B) Temporal profiles for the major frequency components of Ca2+ oscillations. Three frequency components including 100–300 mHz (fast), 10–100 mHz (slow), and <10 mHz (ultraslow) were summarized (n = 3 individual experiments). Frequency components were evaluated by the amplitude integration over each frequency band in FFT spectra. Standard error of the mean (SEM) was calculated when applicable.
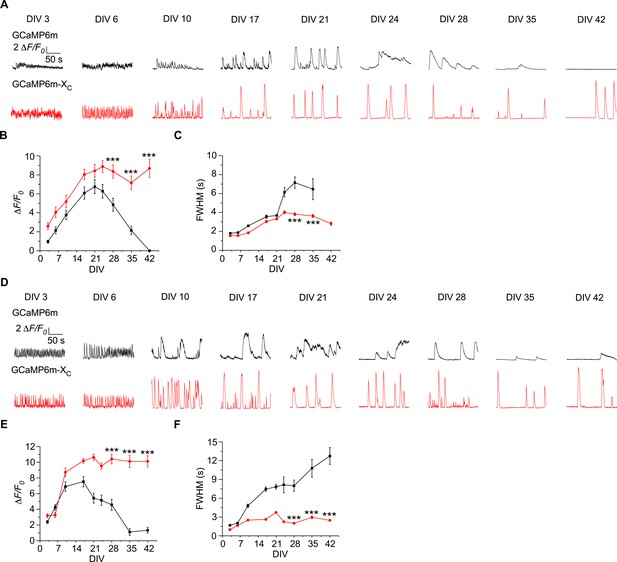
Ca2+ oscillations in long-term cultured cortical neurons expressing GCaMP6m or GCaMP6m-XC in vitro.
(A–C) Ca2+ activities in cultured cortical neurons expressing GCaMP6m (black) or GCaMP6m-XC (red), respectively. Exemplar traces from DIV 3 to DIV 42 (A), summarized by peak amplitudes (ΔF/F0, B) and full width at half maximum (FWHM, C). Note that GCaMP6m on DIV 42 did not record any noticeable response. (D–F) Another set of Ca2+ oscillation data and analyses over multiple weeks similar to (A–C). Standard error of the mean (SEM) and Student’s t-test (two-tailed unpaired with criteria of significance: ***p < 0.001) were calculated when applicable.
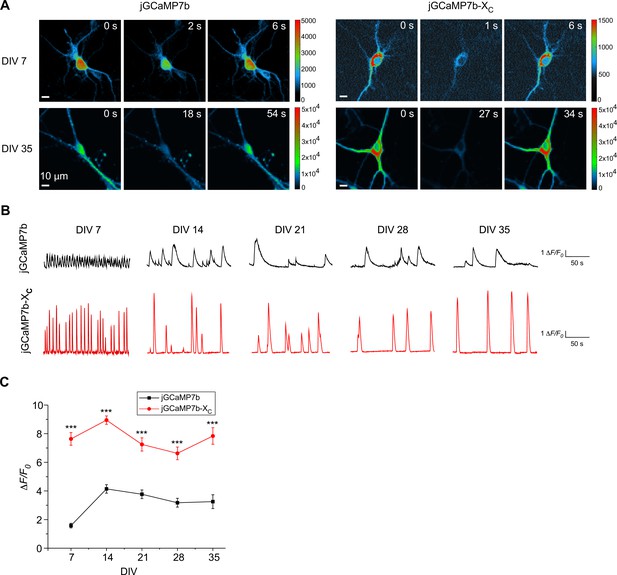
Ca2+ oscillations in cultured cortical neurons expressing jGCaMP7b or jGCaMP7b-XC.
(A) Representative Ca2+ fluorescence images (color coded) of cultured cortical neurons expressing jGCaMP7b or jGCaMP7b-XC on DIV 7 or DIV 35. (B) Representative Ca2+ activity traces of cultured cortical neurons expressing jGCaMP7b (black) or jGCaMP7b-XC (red) across 5 weeks (from DIV 7 to DIV 35). (C) Summarized peak amplitudes in time-dependent profiles (ΔF/F0). Three independent experiments from three independent culture preparations. Standard error of the mean (SEM) and Student’s t-test (two-tailed unpaired with criteria of significance: ***p < 0.001) were calculated when applicable.
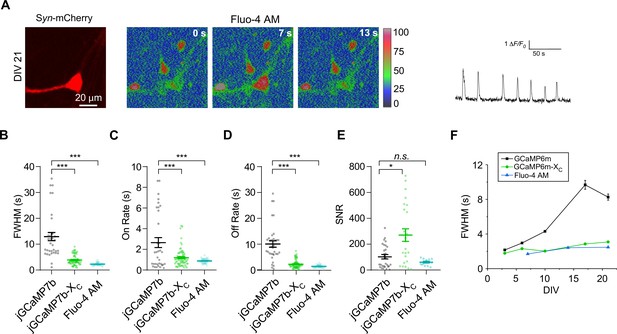
Ca2+ oscillations in cultured cortical neurons imaged by Fluo-4 AM.
(A) Ca2+ signals of cultured cortical neurons on DIV 21. Neurons were infected with pLenti-Syn-mCherry virus to illustrate the cell body (left). Ca2+ fluorescence intensities from neurons loaded with Fluo-4 AM were color coded (middle). A trace of Fluo-4 AM fluorescence represents the Ca2+ signals in a neuron (right). Summary of the key indices: FWHM (in seconds, B), on rate (in seconds, C), off rate (in seconds, D), and SNR (E) of Ca2+ signals acquired by jGCaMP7b, jGCaMP7b-XC, or Fluo-4 AM. (F) Temporal profiles of FWHM measured from spontaneous Ca2+ oscillations acquired by GCaMP6m, GCaMP6m-XC, or Fluo-4 AM. Standard error of the mean (SEM) and one-way analysis of variance (ANOVA) followed by Bonferroni for post hoc tests (criteria of significance: *p < 0.05, ***p < 0.001; n.s. represents ‘not significant’) were applied.
Spontaneous Ca2+ oscillation of cultured cortical neurons virally expressing GCaMP6m-XC on DIV 28.
Neurons exhibited highly synchronized Ca2+ oscillations with robust spikes and complicated neurite connections. Video plays at 60× speed, with each frame in the size of 512×512 pixels or 631×631 µm2.
Spontaneous Ca2+ oscillation of cultured cortical neurons virally expressing GCaMP6m on DIV 17.
Neurons exhibited ultralong lasting Ca2+ signals which may be associated with apoptotic death of neurons. Video plays at 60× speed, with each frame in the size of 512×512 pixels or 631×631 µm2.
Spontaneous Ca2+ oscillation of cultured cortical neurons virally expressing GCaMP6m on DIV 28.
Most of the neurons were broken and no longer exhibited Ca2+ oscillation. Video plays at 60× speed, with each frame in the size of 512×512 pixels or 631×631 µm2.
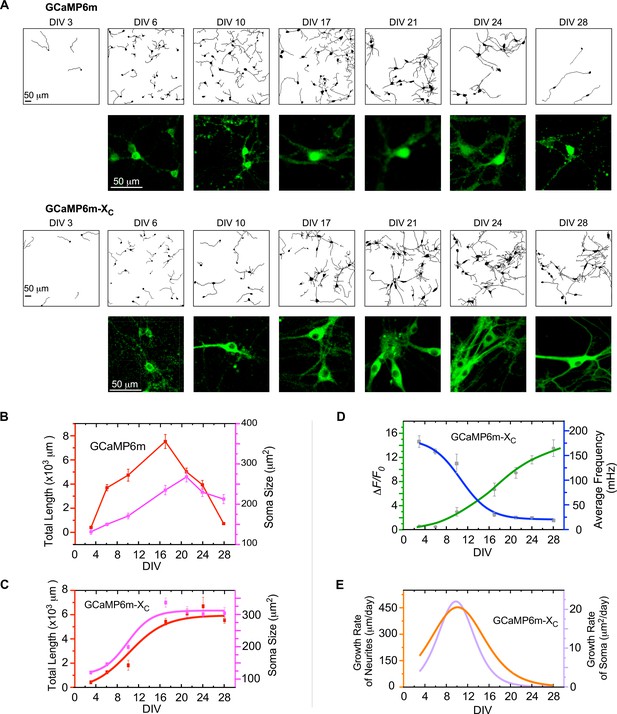
The correlations between neuronal development and Ca2+ oscillations unveiled by GCaMP-X.
(A) Time-lapse images with neurite tracing for cultured cortical neurons expressing GCaMP6m (upper two rows) or GCaMP6m-XC (lower two rows). Enlarged fluorescence images are to show subcellular distributions of the probes, indicative of the nuclear accumulation of GCaMP6m versus GCaMP6m-XC. (B) Temporal profiles of total neurite length per view (red) or soma size per neuron (pink) for neurons expressing GCaMP6m. (C) Temporal profiles of total neurite length per view (red) or soma size per neuron (pink) for neurons expressing GCaMP6m-XC. (D) The temporal profiles of the average frequency of Ca2+ oscillations (blue) and the peak amplitude (ΔF/F0, green), adopted from Figure 3C, D. (E) Temporal profiles of the growth rates (μm/day) of neurite length (orange) or soma size (purple), respectively. Analyses were performed in parallel on both Ca2+ waveforms and neuronal morphology based on the data obtained from the same three independent culture preparations as in Figure 3 (see Figure 4—figure supplement 1 for details on morphology data). Standard error of the mean (SEM) was calculated when applicable.
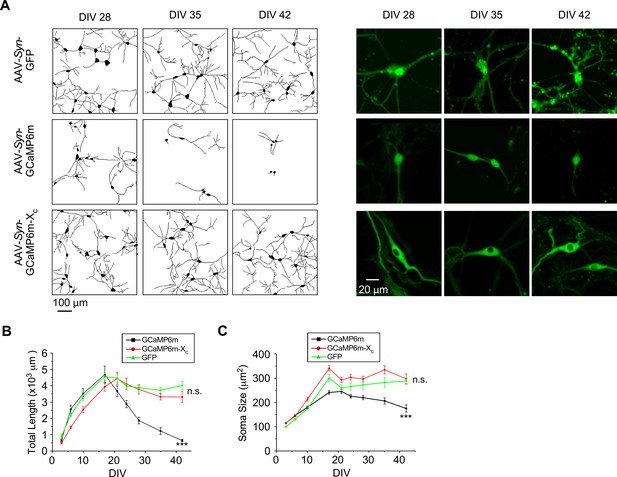
Indistinguishable neuronal morphology between GCaMP6m-XC and GFP of long-term expression.
(A) Representative neurite tracing of multiple neurons (left) and fluorescence images of individual neurons (right) for cultured cortical neurons expressing GCaMP6m-XC, GCaMP6m, or GFP on DIV 28, DIV 35, and DIV 42. Temporal profiles of the total length of neurites (B) and the soma size from DIV 3 to DIV 42 (C). Compared on DIV 42, no significant difference exists between GCaMP6m-Xc and GFP control; and the differences between GCaMP6m-Xc and GCaMP6m are significant. Three independent experiments from three independent culture preparations. Standard error of the mean (SEM) and one-way analysis of variance (ANOVA) followed by Bonferroni for post hoc tests (criteria of significance: ***p < 0.001; n.s. represents ‘not significant’) were calculated when applicable.
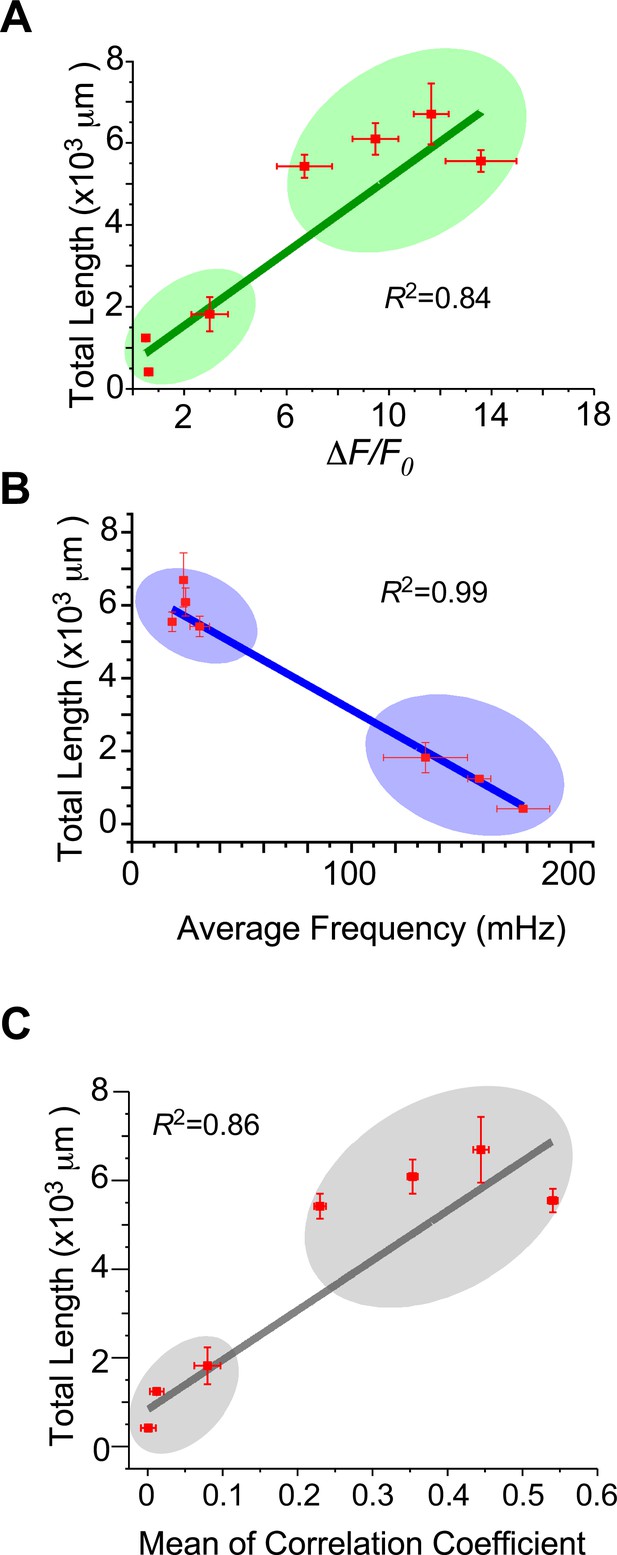
Potential relationships between and neurite length and oscillation characteristics.
The peak amplitude (A), the average frequency (B), or the level of synchrony (C) was examined for its potential correlation with the total length of neurites, based on the data acquired by GCaMP6m-XC in Figures 3 and 4. The linear correlation coefficient (R2) was evaluated between each index and neurite length. Standard error of the mean (SEM) was calculated when applicable.
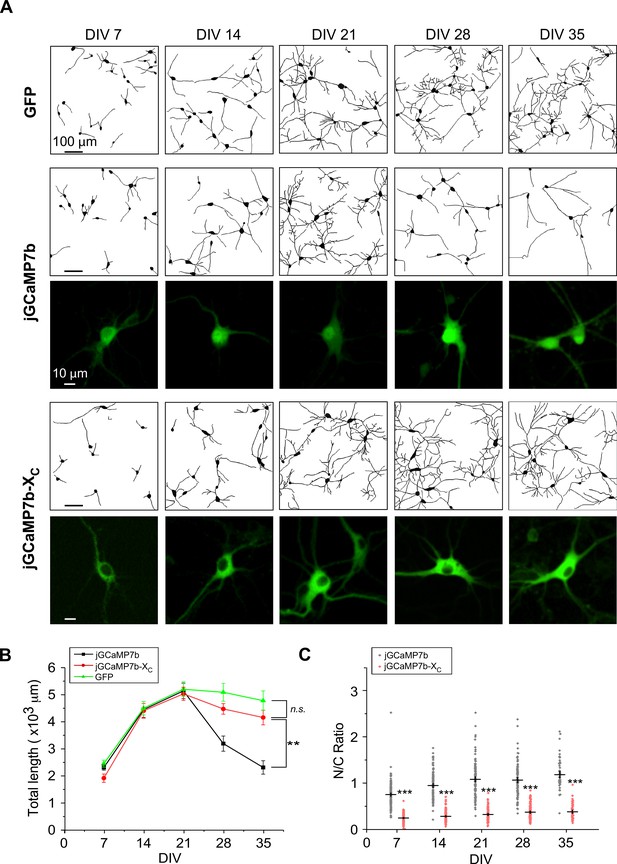
Morphological analysis to compare jGCaMP7b-XC and jGCaMP7b in cultured cortical neurons.
(A) Cortical neurons virally expressing jGCaMP7b-XC, jGCaMP7b, and GFP control, respectively. For each group, neurite tracing of multiple neurons (upper rows) and representative confocal fluorescence images of individual neurons (lower rows) at the selected timepoints of DIV 7 to DIV 35. (B) Temporal profiles of the total length of neurites summarized for jGCaMP7b-XC and jGCaMP7b versus GFP control. (C) N/C ratio of neurons expressing jGCaMP7b or jGCaMP7b-XC. Three independent experiments from three independent culture preparations. Standard error of the mean (SEM) and one-way analysis of variance (ANOVA) followed by Bonferroni for post hoc tests (B) or two-tailed unpaired Student’s t-test (C) (criteria of significance: **p < 0.01; ***p < 0.001; n.s. represents ‘not significant’) were calculated.
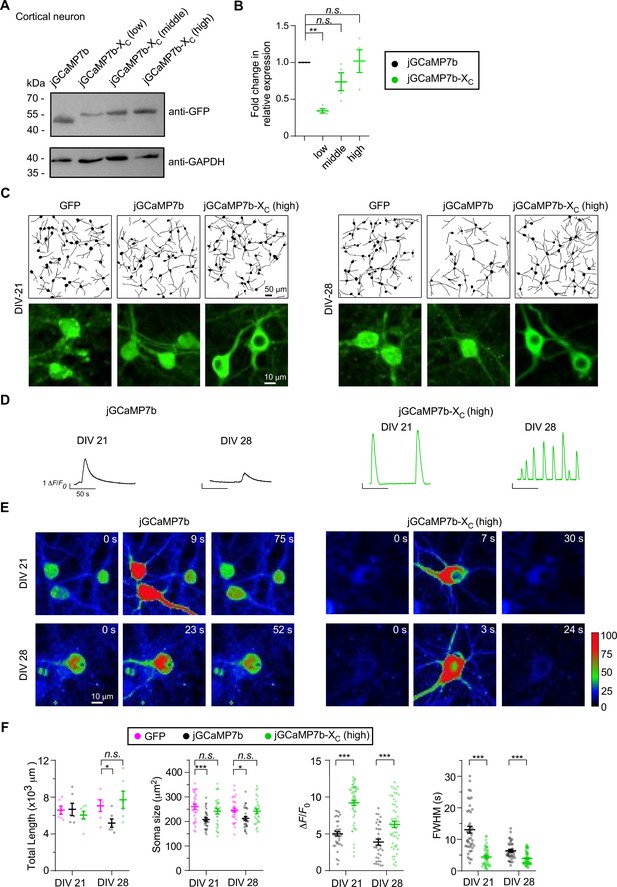
The long-term effects of GCaMP-X with enhanced expression levels in cultured neurons.
(A, B) Protein expression levels in whole-cell extracts from cortical neurons infected with 1 μl AAV-Syn-jGCaMP7b (1.0 × 1012 v.g./ml) or 1 μl (low), 2 μl (middle), 3 μl (high) AAV-Syn-jGCaMP7b-XC (1.0 × 1012 v.g./ml). By western blots, anti-GFP bands and anti-GAPDH bands indicated the level of probe expression and the amount of inputs, respectively. Statistical summary for whole-cell expression levels of low/middle/high-dose jGCaMP7b-XC in comparison to jGCaMP7b. (C–E) Comparison between jGCaMP7b and high-dose jGCaMP7b-XC in cultured cortical neurons. To compare neuritogenesis (C), neurons virally expressing GFP served as the control. Representative Ca2+ waveforms (D) and images (E) are shown for cultured cortical neurons virally expressing jGCaMP7b or jGCaMP7b-XC (high) on DIV 21 and DIV 28. (F) Statistical summary of neurite growth and Ca2+ oscillation, to compare the three groups by the key indices including total neurite length per view, soma size of individual neurons, and peak amplitude and FWHM of Ca2+ waveform. Two independent experiments from two independent culture preparations. Standard error of the mean (SEM) and one-way analysis of variance (ANOVA) followed by Bonferroni for post hoc tests (criteria of significance: *p < 0.05; **p < 0.01; ***p < 0.001; n.s. represents ‘not significant’) were applied.
-
Figure 4—figure supplement 4—source data 1
Source data for Figure 4—figure supplement 4A.
Original uncropped western blotting gels with indication of the cropped areas.
- https://cdn.elifesciences.org/articles/76691/elife-76691-fig4-figsupp4-data1-v2.zip
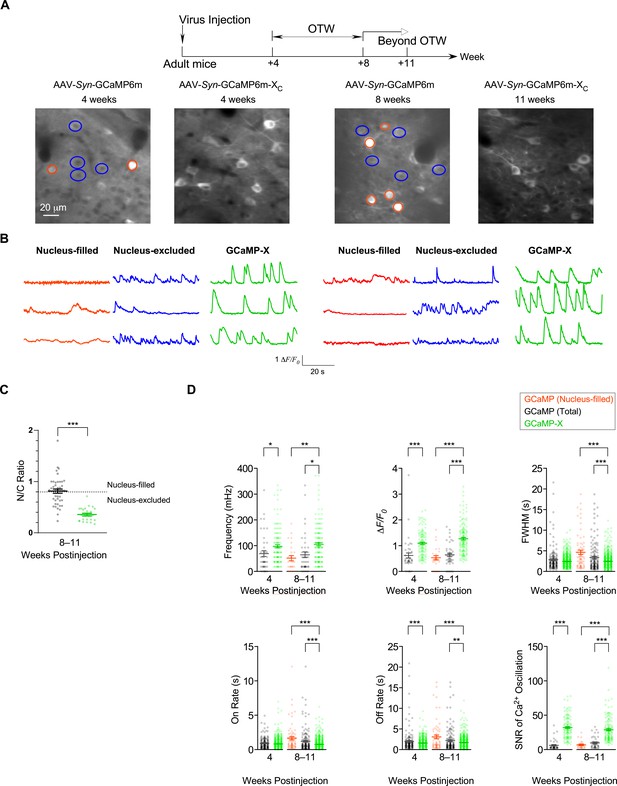
Chronic Ca2+ imaging for spontaneous Ca2+ activities in vivo.
In vivo two-photon fluorescence images (A) and spontaneous nuclear Ca2+ activities (B) of virus-infected neurons in S1 primary somatosensory cortex. Neurons expressing GCaMP6m-XC or GCaMP6m (nucleus-filled and nucleus-excluded) within OTW (optimal time window, 4–8 weeks postinjection) and beyond OTW (8–11 weeks postinjection) were analyzed and compared. (C) N/C ratio summary of GCaMP6m and GCaMP6m-XC in vivo beyond OTW. Neurons were divided into two groups: nucleus-excluded and nucleus-filled, by applying the criteria of N/C ratio (0.8). (D) Key parameters of spontaneous Ca2+ activities. Standard error of the mean (SEM) and two-tailed unpaired Student’s t-test (C) or one-way analysis of variance (ANOVA) followed by Bonferroni for post hoc tests (D) (criteria of significance: *p < 0.05; **p < 0.01; ***p < 0.001) were calculated when applicable. Data were obtained from three or two mice for GCaMP6m and GCaMP6m-XC, respectively.
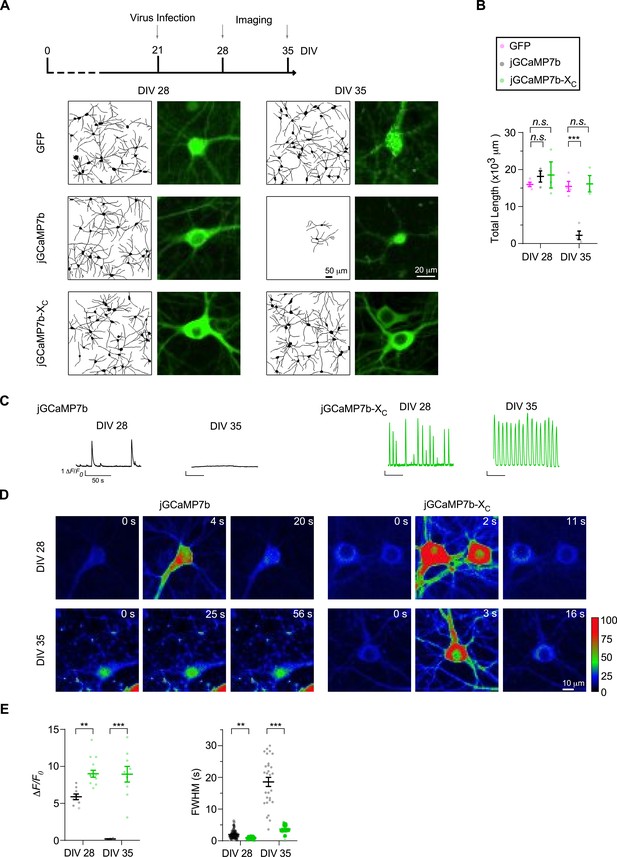
Effects of virally expressed GCaMP versus GCaMP-X on mature cortical neurons.
(A, B) Cultured cortical neurons from newborn ICR mice were infected on DIV 21 with GFP, jGCaMP7b and jGCaMP7b-XC viruses (2 μl of each), respectively. Then neurons were traced by confocal microscopy on DIV 28 and DIV 35 (A). Total length per view is summarized in (B). (C, D) Oscillatory Ca2+ waveforms associated with color-coded fluorescence images on DIV 28 and DIV 35. (E) The key indices with ΔF/F0 and FWHM measured from spontaneous Ca2+ in cultured neurons. Two independent experiments from two independent culture preparations. Standard error of the mean (SEM) and one-way analysis of variance (ANOVA) followed by Bonferroni for post hoc tests (B) or two-tailed unpaired Student’s t-test (E) (criteria of significance: **p < 0.01; ***p < 0.001; n.s. represents ‘not significant’) were calculated.
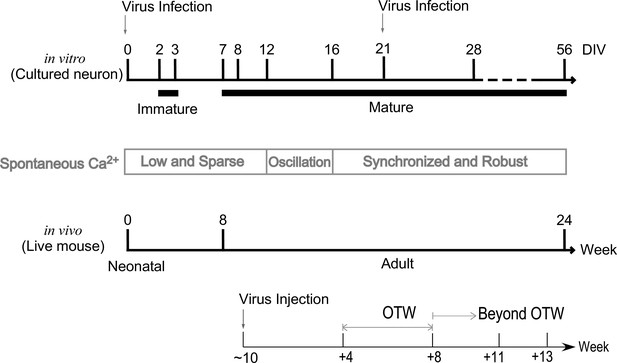
The critical timepoints of the protocols for both in vitro and in vivo experiments.
Neurons at different timepoints exhibit diverse patterns of spontaneous Ca2+ fluctuations. In general, cultured neurons from neonatal mice around DIV 2–3 are considered to be immature with low and sparse oscillations (Isaev et al., 2018). Cultured neurons could enter into mature stage as early as ~DIV 7 (up to DIV 56), corresponding to 8- to 24-week-old adult mice in vivo (Dutta and Sengupta, 2016), where periodical oscillations from multiple neurons emerge around DIV 12–16. Robust and synchronized oscillations are observed usually after DIV 16 (Murphy et al., 2020; Opitz et al., 2002), which correspond to neural network formation and synaptic maturation (Mori and Mook-Jung, 2015). Virus infection was conducted for both neonatal (DIV 0) and mature (DIV 21) neurons to culture further for in vitro experiments. For in vivo studies, adult mice (>8 weeks) were used for virus injection. One to two months (4–8 weeks) postinjection and thereafter (8–13 weeks) are considered as within optimal time window (OTW) and beyond OTW, respectively (Chen et al., 2013; Resendez et al., 2016; Tian et al., 2009).
In vivo two-photon imaging of spontaneous Ca2+ oscillation of neurons virally expressing GCaMP6m-XC in S1 primary somatosensory cortex beyond optimal time window (11 weeks postinjection).
Enlarged images (512 × 512 pixels, 300 × 300 µm2) of L2/3 cells (150–250 µm under the pia) in S1BF were collected at 7.4 Hz frame rate. Video plays at 6× speed.
In vivo two-photon imaging of spontaneous Ca2+ oscillation of neurons virally expressing GCaMP6m in S1 primary somatosensory cortex beyond optimal time window (8 weeks postinjection).
Enlarged images (512 × 512 pixels, 300 × 300 µm2) of L2/3 cells (150–250 µm under the pia) in S1BF were collected at 7.4 Hz frame rate. Neurons with nucleus-filled GCaMP6m barely fired. Video plays at 6× speed.
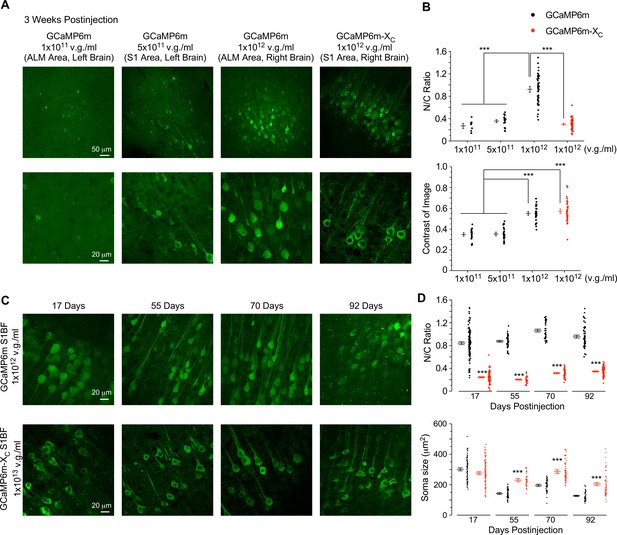
Evaluation of neuronal morphology for long-term in vivo expression of GCaMP-X versus GCaMP.
(A) Confocal brain-slice images for ALM (anterolateral motor cortex) or S1 (primary somatosensory cortex). For the same mouse, AAV-Syn-GCaMP6m or AAV-Syn-GCaMP6m-XC (30 nl) of the indicated titers were injected into the left or right brain. Brain slices were dissected 3 weeks after virus injection. (B) N/C ratio (upper) and contrast of images (lower), summarized over three mice. (C) Confocal images of brain slices expressing GCaMP6m or GCaMP6m-XC acquired at different timepoints up to 92 days postinjection. AAV-Syn-GCaMP6m or AAV-Syn-GCaMP6m-XC (60 nl) viruses of the indicated titers were microinjected into the left or right S1BF (barrel field of S1) of the same mouse, respectively. (D) Summary of N/C ratio (upper) and soma size (lower) for the neurons expressing GCaMP6m or GCaMP6m-XC (eight mice in total). Standard error of the mean (SEM) and one-way analysis of variance (ANOVA) followed by Bonferroni for post hoc tests (criteria of significance: ***p < 0.001) were calculated when applicable.
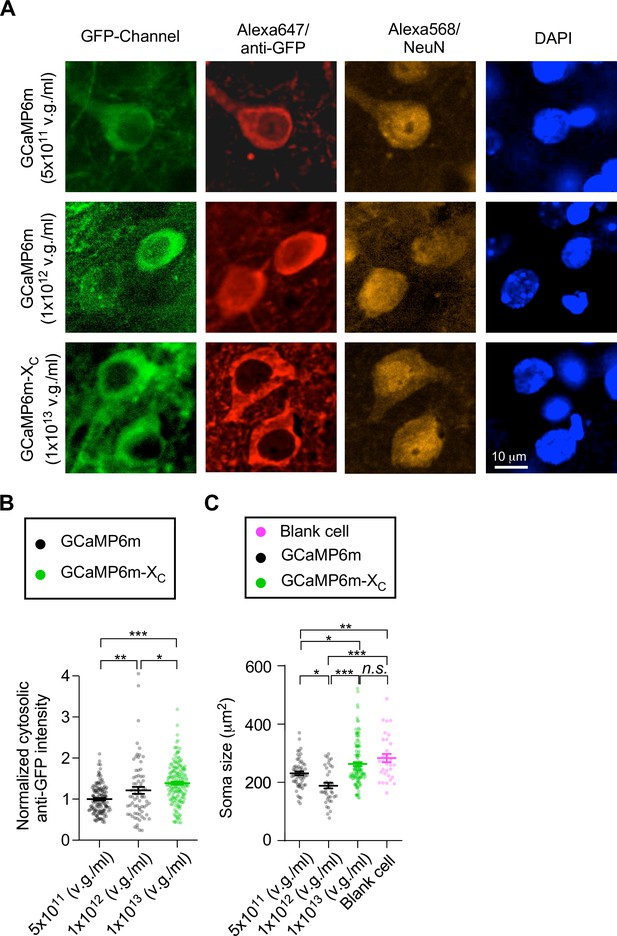
Long-term effects of GCaMP-X with high-expression levels in vivo.
(A) Immunostaining images of neurons infected by AAV-Syn-GCaMP6m (5 × 1011 or 1.0 × 1012 v.g./ml, 60 nl) and AAV-Syn-GCaMP6m-XC (1.0 × 1013 v.g./ml, 60 nl) at left or right S1BF. Thirteen weeks after microinjection, brain slices of injected mice were stained with anti-GFP (Alexa Fluo 647 nm, red), NeuN (Alexa Fluo 568 nm, yellow), and DAPI (blue) to reflect the protein expression of the indicators, cell bodies, and nuclear envelops, respectively. The GFP channel (green) was to indicate the positive neurons. Neurons without fluorescence in GFP channel and anti-GFP channel served as the blank cell control. (B) Cytosolic fluorescence intensity of anti-GFP of each neuron was normalized by that of low-dose GCaMP6m group (5 × 1011 v.g./ml) then summarized and compared. (C) Soma size calculated for each neuron and summarized to compare. The group of high-dose GCaMP6m-XC had the highest level of expression whereas causing the least damage to neurons judged from its largest soma size among the three groups of neurons. Standard error of the mean (SEM) and one-way analysis of variance (ANOVA) followed by Bonferroni for post hoc tests (criteria of significance: *p < 0.05; **p < 0.01; ***p < 0.001; n.s. represents ‘not significant’) were applied.
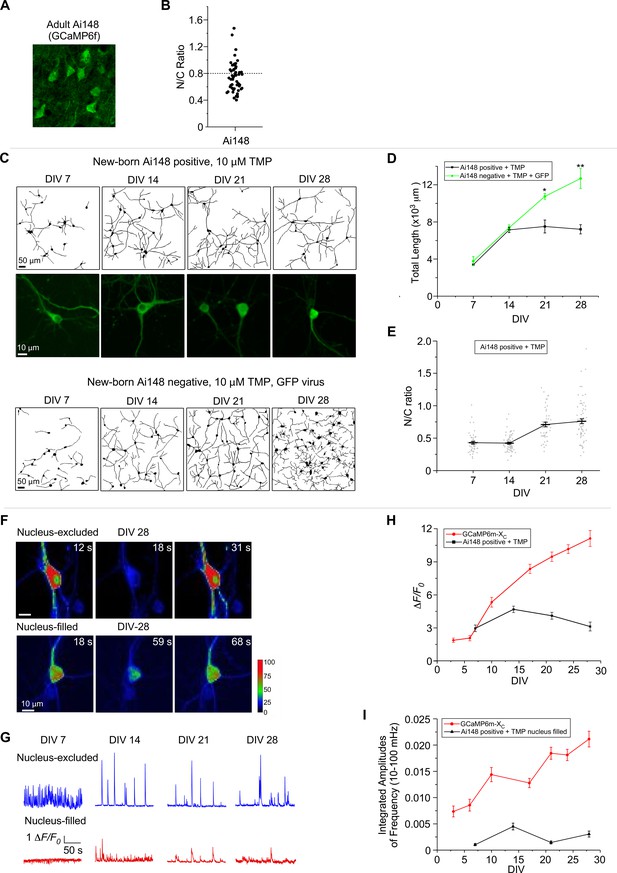
Chronic imaging for cultured cortical neurons of GCaMP transgenic mice.
(A) Confocal brain-slice images of cortex layer II–III from 6-month-old GCaMP6f-positive transgenic mice (Rasgrf2-2A-dCre;Ai148 mice). GCaMP6f was examined ~4 months after induction of expression by intraperitoneal injection of TMP. (B) The N/C ratio of layer II–III neurons of adult Ai148 mice. (C) Neurite growth of cultured cortical neurons from newborn Ai148 mice. Neurite tracing for GCaMP-positive neurons (upper two rows) versus GCaMP-negative neurons (infected by GFP virus, bottom row), both added with 10 µM TMP to induce transgene expression. Zoomed confocal images illustrate subcellular distributions of GCaMP (middle row). Temporal profiles of total neurite length (D) or N/C ratio (E) for GCaMP-expressing Ai148 neurons, in comparison with GCaMP-negative GFP-infected neurons. (F) Time-laps images of single-neuron Ca2+ dynamics on DIV 28 from the nucleus-excluded and nucleus-filled subgroups. Color coding indicates fluorescence intensity. (G) Spontaneous Ca2+ activities of GCaMP-positive neurons in the nuclear-excluded and nuclear-filled subgroups from DIV 7 to DIV 28. Temporal profiles of the peak amplitudes (ΔF/F0, H) and the integrated amplitudes (over the frequency band of 10–100 mHz, I) for Ai148 neurons, compared with GCaMP-XC. Data were based on 3 Ai148 mice (A, B), and two independent experiments from two independent culture preparations (C–I). Standard error of the mean (SEM) and two-tailed unpaired Student’s t-test (D) (criteria of significance: *p < 0.05; **p < 0.01) were calculated when applicable.
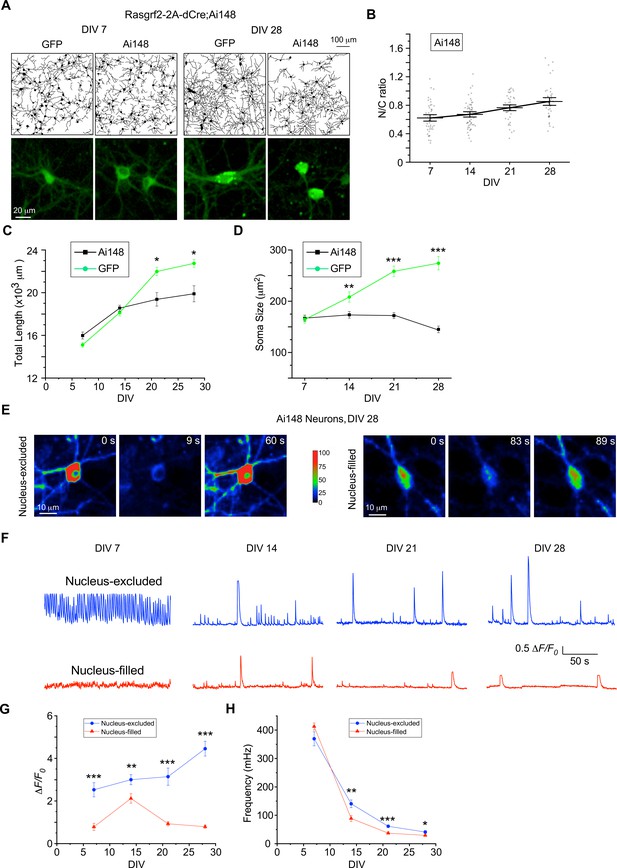
Chronic evaluation of cultured cortical neurons from Ai148 mice with inducible GCaMP6f expression.
(A) Representative neurite tracing and fluorescence images for cultured cortical neurons expressing GCaMP6f or GFP on DIV 7 and DIV 28, respectively. GCaMP6f expression was induced by 10 μM TMP from Rasgrf2-2A-dCre;Ai148 mice. GFP control neurons were also treated with the same dose of TMP. Temporal profiles of N/C ratio (B), total neurite length (C), and soma size (D) for Ai148 neurons. (E, F) Spontaneous Ca2+ oscillations from the nucleus-excluded versus nucleus-filled (N/C ratio >0.8) subgroups of neurons from Ai148 mice. Time-laps color-coded Ca2+ fluorescence images on DIV 28 (E) and Ca2+ waveforms from DIV 7 to DIV 28 (F). Temporal profiles of peak amplitude (ΔF/F0, G) and oscillation frequency (mHz, H) Standard error of the mean (SEM) and Student’s t-test (two-tailed unpaired with criteria of significance: *p < 0.05; **p < 0.01; ***p < 0.001) were calculated when applicable.
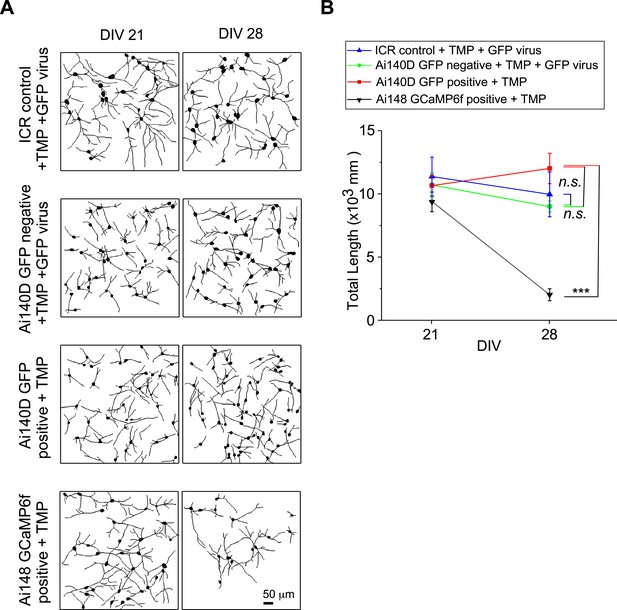
Neuritogenesis of cortical neuron from transgenic mice with TMP-inducible GCaMP.
(A) Neurite tracings for Ai148 GCaMP6f-positive neurons (Rasgrf2-2A-dCre;Ai148 mice) versus Ai140D GFP-positive neurons (Rasgrf2-2A-dCre;Ai140D mice) on DIV 21 and DIV 28, both induced by 10 μM TMP. Ai140D GFP-negative neurons and ICR mouse neurons serves as additional controls, both infected GFP viruses, and also added with 10 μM TMP. (B) Statistical summary of total neurite length per view. No significant difference was found among ICR control, Ai140D GFP-negative, and Ai140D GFP-positive neurons, thus ruling out the potential biases or artifacts due to tTA toxicity, different strains of mice, or virus effects. The total length of neurites was significantly reduced in Ai148 GCaMP6f-positive neurons, as compared with Ai140D GFP-positive neurons. Standard error of the mean (SEM) and one-way analysis of variance (ANOVA) followed by Bonferroni for post hoc tests (criteria of significance: ***p < 0.001; n.s. represents ‘not significant’) were applied.
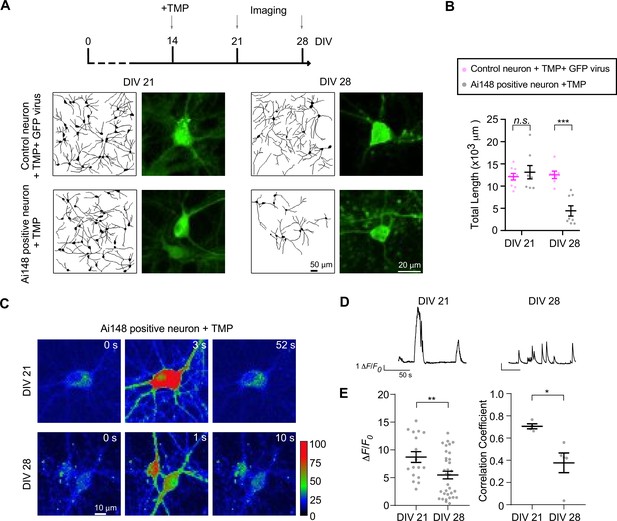
Effects on cortical neurons by transgenic GCaMP6f expression induced at the mature stage.
(A, B) GCaMP6f was induced in cultured cortical neurons from Rasgrf2-2A-dCre;Ai148 mice by 10 μM TMP on DIV 14, then imaged by confocal microscopy on DIV 21 and DIV 28. Neurons from ICR mice treated with TMP treatment and infected by GFP virus served as the control. Based on neurite tracings (A), the total length per view (B) was summarized. Fluorescence images were zoomed in to show single neurons. (C, D) Oscillatory Ca2+ waveforms associated with color-coded fluorescence images on DIV 21 and DIV 28. (E) Statistical summary for the key indices of ΔF/F0 (from each neuron) and correlation coefficient (from each view). Two independent experiments were performed with two independent culture preparations. Standard error of the mean (SEM) and two-tailed unpaired Student’s t-test (criteria of significance: *p < 0.05; **p < 0.01; ***p < 0.001; n.s. represents ‘not significant’) were applied.
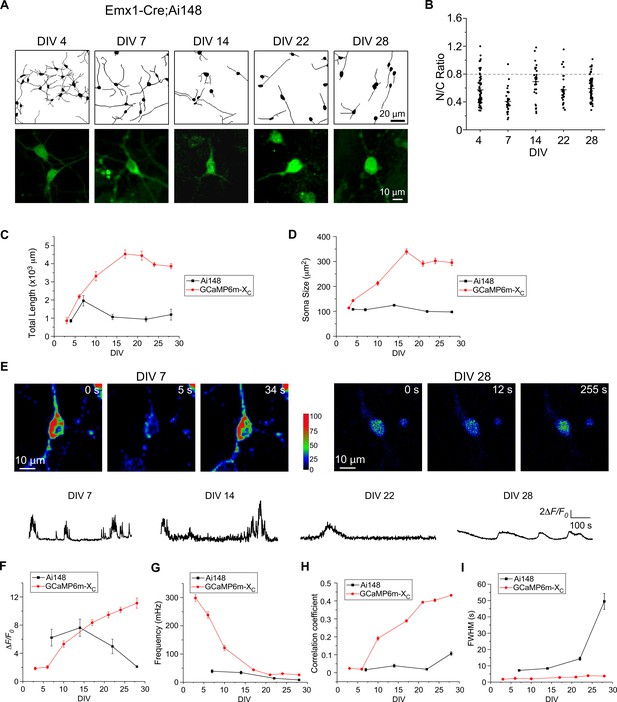
Long-term culturing and imaging of cortical neurons from Emx1-Cre;Ai148 mice with constitutive GCaMP6f expression.
(A) Representative neurite tracing and fluorescence images for cultured cortical neurons constitutively expressing GCaMP6f from Emx1-Cre;Ai148 mice on selected DIV. (B) Temporal profiles of N/C ratio collected from Ai148 neurons. The dotted line indicates the criteria of N/C ratio 0.8. (C, D) Temporal profiles of total neurite length (C) and soma size (D). Data points and lines in red represent the average results (over 3 independent trials of ~4-week culturing/imaging) from viral GCaMP6m-XC. (E) Oscillatory Ca2+ waveforms associated with color-coded fluorescence images. (F-I) Temporal profiles of peak amplitude (ΔF/F0, F), oscillation frequency (mHz, G), synchronization (correlation coefficient, H), and FWHM (sec, I). Data points and lines in red represent the average results (over 3 independent trials of ~4-week culturing/imaging) from viral GCaMP6m-XC. Standard error of the mean (SEM) was calculated when applicable.
Tables
Reagent type (species) or resource | Designation | Source or reference | Identifiers | Additional information |
---|---|---|---|---|
Strain, strain background (Mus musculus) | ICR | Institute of Cancer Research | Produced by Tsinghua University & Beihang University | |
Strain, strain background (Mus musculus) | C57 | Jackson Labs | C57BL/6J; stock no. 000664 | Produced by Tsinghua University |
Strain, strain background (Mus musculus) | Ai148 (or Ai148D) | Jackson Labs | B6.Cg-Igs7tm148.1(tetO-GCaMP6f,CAG-tTA2)Hze/J; stock no.030328 | |
Strain, strain background (Mus musculus) | Rasgrf2-2A-dCre | Jackson Labs | B6;129S-Rasgrf2tm1(cre/folA)Hze/J; stock no.022864 | |
Strain, strain background (Mus musculus) | Ai140D | Jackson Labs | B6.Cg-Igs7tm140.1(tetO-EGFP,CAG-tTA2)Hze/J; stock no.030220 | |
Strain, strain background (Mus musculus) | Emx1-cre | Jackson Labs | B6.129S2-Emx1tm1(cre)Krj/J; stock no.005628 | |
Cell line (Homo sapiens) | Kidney (normal epithelial, embryo) | ATCC | HEK293 | |
Antibody | anti-GFP antibody (Rabbit polyclonal) | Abcam | Cat#ab290; RRID: AB_2313768 | WB (1:1000); IF (1:200) |
Antibody | anti-GAPDH antibody (Rabbit polyclonal) | Gene-Protein Link | Cat#P01L081 | WB (1:10,000) |
Antibody | anti-Histone H3 antibody (Mouse monoclonal) | Beyotime | Cat#AF0009; RRID: AB_2715593 | WB (1:1000) |
Antibody | anti-Calmodulin 1/2/3 antibody (Rabbit monoclonal) | Abcam | Cat#ab45689; RRID: AB_725815 | WB (1:10,000) |
Antibody | anti-His antibody (Rabbit monoclonal) | Gene-Protein Link | Cat#P01L071 | WB (1:2000) |
Antibody | HRP-conjugated Affinipure Goat Anti-Mouse IgG (H + L) | Proteintech | Cat#SA00001-1; RRID: AB_2722565 | WB (1:10,000) |
Antibody | HRP-conjugated Affinipure Goat Anti-Rabbit IgG (H + L) | Proteintech | Cat#SA00001-2; RRID: AB_2722564 | WB (1:10,000) |
Antibody | anti-NeuN antibody (Mouse monoclonal) | Millipore | Cat#MAB377; RRID: AB_2298772 | IF (1:500) |
Antibody | Goat anti-Mouse IgG (H+L) Alexa Fluor 568 (Goat polyclonal) | Invitrogen | Cat#A11004; RRID:AB_2534072 | IF (1:800) |
Antibody | Goat anti-Rabbit IgG (H+L) Alexa Fluor 647 (Goat polyclonal) | Invitrogen | Cat#A21244; RRID:AB_2535812 | IF (1:800) |
Chemical compound, drug | DAPI | Beyotime | 2-(4-Amidinophenyl)-6-indolecarbamidine dihydrochloride; Cat#C1002 | IF (1:1000) |
Chemical compound, drug | TMP | Sigma | Trimethoprim, Cat#T7883-5G | Dilute to DMSO |
Chemical compound, drug | Fluo-4 AM | Beyotime | Cat#S1060 | |
Chemical compound, drug | Pluronic F-127 | Beyotime | Cat#ST501-1g | |
Commercial assay or kit | Nuclear extraction kit | Abcam | Cat#113474 | |
Software, algorithm | GraphPad Prism v8.0.1 | GraphPad Software | RRID:SCR_002798 | |
Software, algorithm | Imaris Viewer x64 v7.7.2 | Oxford Instruments Group | RRID:SCR_007370 | |
Software, algorithm | Fiji v6.6.1 | National Institutes of Health | RRID:SCR_002285 | |
Software, algorithm | Origin 2019b | OriginLab | RRID:SCR_014212 | |
Software, algorithm | Clampex | Molecular Devices | RRID:SCR_011323 | |
Software, algorithm | Matlab | MathWorks | RRID:SCR_001622 | |
Recombinant DNA reagent | pGP-CMV-GCaMP6m (plasmid) | PMID:23868258 | RRID:Addgene_40754 | |
Recombinant DNA reagent | pEGFP-N1-GCaMP6m-XC (plasmid) | PMID:29666364 | RRID:Addgene_111543 | |
Recombinant DNA reagent | pGP-CMV-jGCaMP7b (plasmid) | PMID:31209382 | RRID:Addgene_104484 | |
Recombinant DNA reagent | pEGFP-N1-jGCaMP7b-XC (plasmid) | Created in this study | RRID:Addgene_178361 | NES tag added to jGCaMP7b-X,generated by Liu Lab. |
Recombinant DNA reagent | pEGFP-N1-jGCaMP7b-XN (plasmid) | Created in this study | RRID:Addgene_178362 | NLS tag added to jGCaMP7b-X,generated by Liu Lab. |
Recombinant DNA reagent | pcDNA3-α1DL (plasmid) | PMID:35589958 | Plasmid to express full length α1DL,generated by Liu Lab. | |
Recombinant DNA reagent | pcDNA3-α1DL-3xFlag (plasmid) | PMID:35589958 | Plasmid to express full length α1DL with C-terminal 3xFlag tag,generated by Liu Lab. | |
Recombinant DNA reagent | pcDNA3-Flag-CaMBD_α1DL (plasmid) | Created in this study | Plasmid to express CaMBD of α1DL with N-terminal Flag tag,generated by Liu Lab. | |
Recombinant DNA reagent | pcDNA3.1-YFP-Ng_S36A-Myc-HisA (plasmid) | Created in this study | Plasmid to express Ng_S36A with C-terminal Myc-His tag,generated by Liu Lab. | |
Other | AAV2/DJ-Syn-GCaMP6m | Hanbio Biotechnology | Adeno-associated virus to express GCaMP6m in vitro | |
Other | AAV2/DJ-Syn-GCaMP6m-XC | Hanbio Biotechnology | Adeno-associated virus to express GCaMP6m-XC in vitro | |
Other | AAV2/DJ-Syn-jGCaMP7b | Hanbio Biotechnology | Adeno-associated virus to express jGCaMP7b in vitro | |
Other | AAV2/DJ-Syn-jGCaMP7b-XC | Hanbio Biotechnology | Adeno-associated virus to express jGCaMP7b-XC in vitro | |
Other | AAV2/DJ-Syn-ZsGreen | Hanbio Biotechnology | Adeno-associated virus to express ZsGreen in vitro | |
Other | pLenti-Syn-mCherry | OBiO Technology | pLenti virus to express mCherry in vitro | |
Other | AAV2/9-Syn-GCaMP6m | BrainVTA | Adeno-associated virus to express GCaMP6m in vivo | |
Other | AAV2/9-Syn-GCaMP6m-XC | BrainVTA | Adeno-associated virus to express GCaMP6m-XC in vivo |