Evolutionary convergence of a neural mechanism in the cavefish lateral line system
Figures
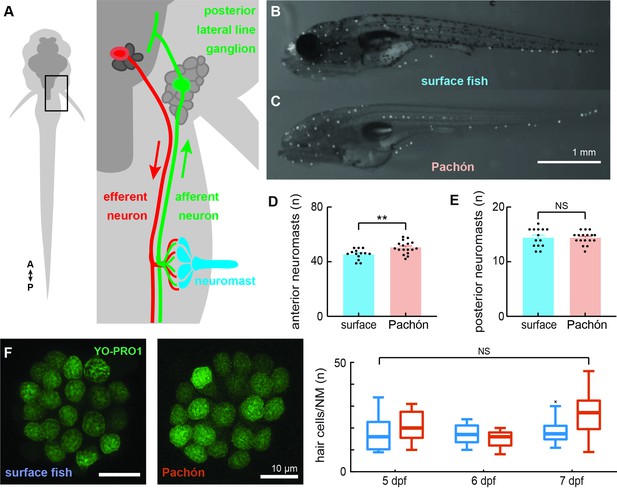
Surface fish and cavefish lateral lines are anatomically similar in early development.
Illustration (not to scale) of the canonical circuit of lateral line function depicting the neuromast (blue) comprised of mechanoreceptive hair cells that are innervated by ascending afferent neurons (green) that collect into the posterior lateral line ganglion. Putative cholinergic efferent neurons (red) descend from the hindbrain and project onto neuromast hair cells (A). 2-[4-(Dimethylamino)styryl]-1-ethylpyridinium iodide (DASPEI) staining of 6 dpf surface (n = 14, B) and cavefish (n = 17, C) show significantly different quantities of anterior lateral line neuromasts (p < 0.01, D) and similar quantities of posterior neuromasts (p = 0.77, E). Hair cells were labeled with a 30-min treatment of YO-PRO1 (green) and found to be of similar quantities between surface (n = 9) and cave (n = 11) populations (unpaired two-way t-test, p = 0.09, F). Box-and-whisker plot representing the number of hair cells per neuromast was indistinguishable across development (5–7 dpf; p = 0.06, G). Error bars are ± standard error (SE) and ** denotes a significant difference (p < 0.01) detected by an unpaired t-test.
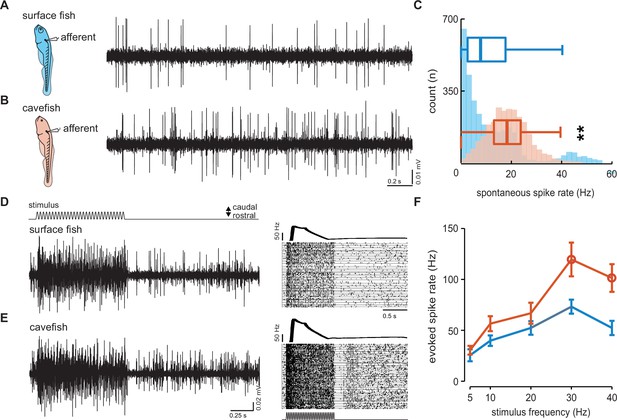
Dynamics of spontaneous and evoked afferent neuron spike activity is elevated in cavefish.
Extracellular recordings were made in posterior lateral line afferents where the neuromast densities and hair cell quantities were similar to resolve the differences observed in afferent activity between larval surface fish (A) and Pachón cavefish (B) between 4 and 7 dpf. The number of occurrences and median intrinsic spike rates in both surface (blue; 12.4 Hz, n = 10 fish) and Pachón (red; 18.6 Hz, n = 5 fish) fish suggests that lateral line response thresholds in cavefish are lower than those of surface fish (C). Evoked afferent activity during stimulation of a single neuromast in surface fish (n = 10, D) and cavefish (n = 12, E) and peristimulus time histograms demonstrate elevated spike rate in cavefish. Evoked spike rate was stimulus frequency dependent and pairwise comparisons reveal significantly (open circles) elevated sensitivity in cavefish at 30 and 40 Hz (p = 0.03 and p < 0.01, respectively, F). Error bars are ± standard error (SE) and ** denotes a significant difference (p < 0.01) detected by an unpaired t-test.
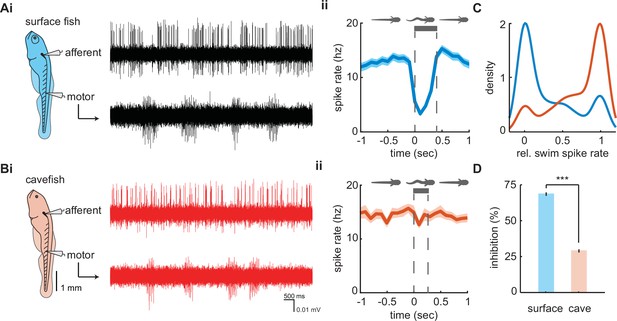
Afferent spike rate decreases during swimming in surface fish but not cavefish.
Simultaneous recordings from afferent neurons from the posterior lateral line afferent ganglion and ventral motor roots along the body in (Ai) surface fish and (Bi) Pachón cavefish. Afferent spike rate decreases at the onset of swimming (time = 0) in (Aii) surface fish while (Bii) Pachón cavefish spike rate remained relatively constant during swimming. Bars represent average swim duration for surface fish (357 ± 5 ms, n = 2272 swims) and Pachón cavefish (264 ± 4 ms, n = 2612 swims). (C) Kernel density estimate of spike rate during swimming relative to the pre-swim interval in both surface fish (blue) and cavefish (red). (D) Surface fish experience greater levels of inhibition during swimming than cavefish. Significance level p < 0.001 indicated by ‘***’ detected via unpaired two-way t-test. Error bars are ± standard error (SE).
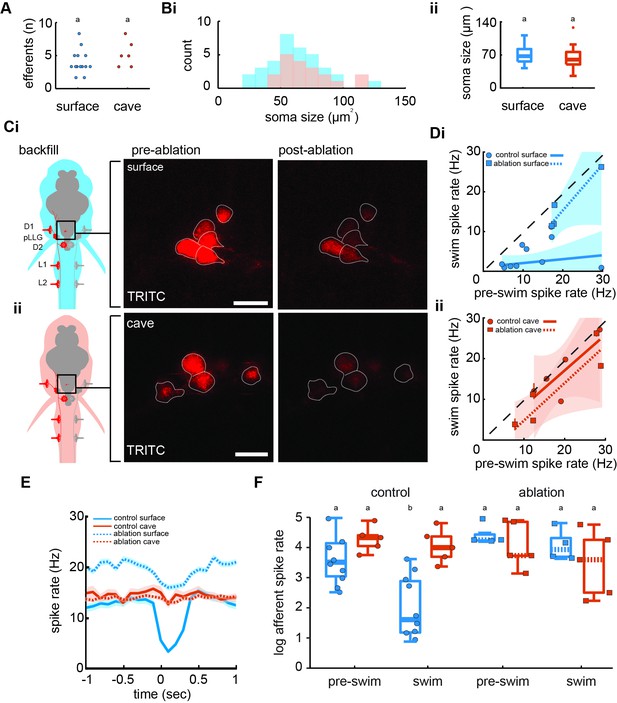
Efferent neurons are necessary for inhibition observed in afferents during swimming in surface fish but not cavefish.
(A) Backfilled hindbrain cholinergic efferent neurons were present in comparable numbers (2–3 cells) in both surface fish (n = 14) and cavefish (n = 6, unpaired two-way t-test: p = 0.2). (Bi) Efferent soma size in surface (blue) and cavefish (red) is similar in both populations (unpaired two-way t-test: p = 0.1, ii). (C) Efferent cell bodies were identified by backfilling rhodamine through posterior lateral line neuromasts in both surface fish (i) and cavefish (ii) and were ablated with a 30-s UV pulse. Scale bar: 20 µm. (D) The line of best fit of spike rates before compared to during the swim significantly excludes unity in nonablated, control surface fish (CI: -0.2 to 0.4, circle), but not in ablated surface fish (CI: -0.1 to 2.4, square), implying spike rate suppression in the former but not the latter (i). The slope of the line for control fish suggests the inhibition is not correlated to the spontaneous afferent activity preceding the swim (p = 0.2). The line of best fit of Pachón cavefish pre-swim and swim spike rates did not exclude unity in both control (CI: -0.5 to 2.2, circle) and ablated (CI: 0.9 to 1.8, square) treatments (ii). Dashed line indicates the line of unity, corresponding to no average difference of spike rate during swimming. (E) Instantaneous spike rates of Pachón cavefish were unaffected by ablating the lateral line whereas the inhibitory effect was eliminated in ablated surface fish. Time is relative to the onset of motor activity. (F) Surface fish (blue) display reduced spike rates during swimming compared to before swimming in control fish (Tukey’s post-hoc test: p < 0.01). Pachón cavefish (red) did not display reduced spike rate during swimming in neither control nor ablated treatments. Ablated surface individuals also did not display reduced spike rate during swimming resulting in a signaling phenotype comparable to cavefish. Groupings of statistical similarity are denoted by ‘a’ and ‘b’, whereas a is significantly different from b. All error bars represent ± standard error (SE).
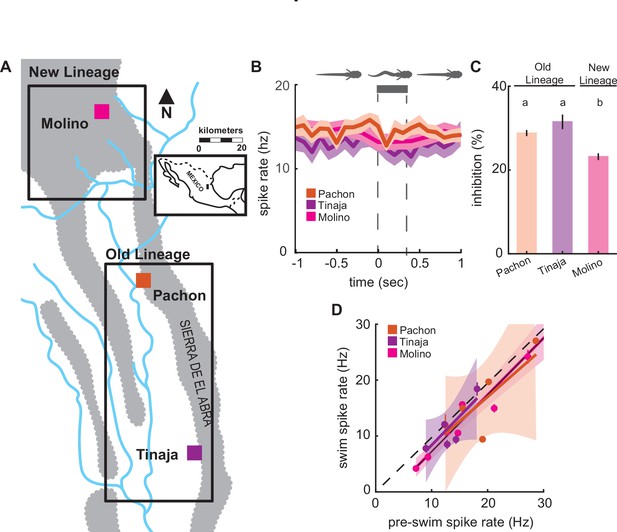
Enhanced lateral line sensitivity during swimming convergently evolved across three blind populations.
(A) Molino cave populations (pink; New Lineage) have evolved more recently relative to Pachón (red) and Tinaja (purple; Old Lineage) cave populations. Lineage delineations inferred from phylogenetic data (Herman et al., 2018). (B) Mean spontaneous afferent spike rate remains constant at the onset of fictive swimming (time = 0) in Pachón (n = 5), Molino (n = 8), and Tinaja (n = 5) populations (N-way ANOVA: p = 0.52).Bar represents average swim duration for Pachón (0.27 s, n = 2429 swim bouts), Molino (0.42 s, n = 1,474 swim bouts), and Tinaja (0.35 s, n = 464). (C) Percent change in spike rate from pre-swim to swim intervals (i.e., inhibition) was small, but significantly different between blind cave populations. Post hoc Tukey test revealed that Molino cavefish experienced significantly less reduction in spike rate when compared to Pachón and Tinaja populations (p < 0.01). Statistically similar groups are indicated by ‘a’ and ‘b’. (D) The line of best fit of pre-swim and swim spike rates does not significantly exclude unity in any of the blind cavefish populations implying there is no detectable inhibitory effect (Pachón: CI = -0.5 to 2.2, Tinaja: CI = -0.2 to 2.2, Molino: CI = 0.8 to 1.2). Dashed line indicates the line of unity, corresponding to no average difference of spike rate during swimming. All values represent mean ± standard error (SE).
Additional files
-
Supplementary file 1
Data collected from electrophysiology recordings and hair cell imaging.
Afferent spike rate during motor inactivity, fictive swimming, and neuromast deflection. Data include information related to the parameters of fictive swim bouts (i.e., tail-beat frequency, swim duration, and duty cycle) as well as stimulus frequency associated with evoked activity. Imaging data denote the population (Pachón or surface), age, and neuromast identity associated with each hair cell count.
- https://cdn.elifesciences.org/articles/77387/elife-77387-supp1-v2.xlsx
-
MDAR checklist
- https://cdn.elifesciences.org/articles/77387/elife-77387-mdarchecklist1-v2.pdf