The integrated stress response remodels the microtubule-organizing center to clear unfolded proteins following proteotoxic stress
Figures
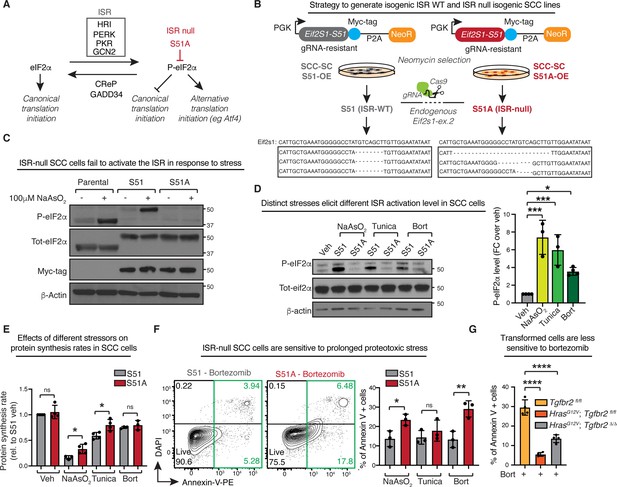
The integrated stress response (ISR) induces a pro-survival program in squamous cell carcinoma (SCC) cells upon exposure to proteotoxic stress.
(A) Schematic of the integrated stress response pathway showing activating kinases, inhibitory phosphatases, and downstream effects. Mutation of eIF2α serine 51 to alanine renders cells insensitive to the ISR activating kinases (‘ISR-null’). (B) Reconstitution and knockout strategy to generate ISR-null SCC cell lines. Constructs encoding a myc-epitope tagged and CRISPR-Cas9-resistant eIF2α (either S51 or S51A) are packaged into lentivirus and integrated into a parental SCC stem cell (SCC-SC) line. Following neomycin selection, CRISPR-Cas9 is delivered by RNP transfection to selectively knock out endogenous eIF2α alleles. Clones grown from single cells are analyzed for knockout by deep sequencing the gRNA target locus. (C) Representative immunoblot confirms both the replacement of the endogenous eIF2α with myc-tagged eIF2α and the failure of cells expressing eIF2α-S51A to activate the ISR. Cells were treated with 100 μM sodium arsenite for 6 hr to induce oxidative stress. (D) ISR-competent cells induce eIF2α phosphorylation upon stress. Representative immunoblot shows levels of eif2α phosphorylation of cells treated with either 50 μM sodium arsenite, 100 ng/mL tunicamycin, or 100 nM bortezomib for 6 hr. Bar graph shows normalized levels of eIF2a phosphorylation ± SD in three independent biological experiments. *p<0.05, ***p<0.001 (t-test). (E) Bar graph shows normalized protein synthesis rates ± SD relative to vehicle-treated S51 SCC cells in S51 and S51A cells treated with either 50 μM sodium arsenite, 100 ng/mL tunicamycin, or 100 nM bortezomib for 6 hr in at least three independent biological experiments. *p<0.05, ns, no statistical significance (t-test). (F) ISR-null SCC cells are more sensitive to stress. Early signs of apoptosis are measured by Annexin-V binding in control and ISR-null cells treated with 5 μM sodium arsenite, 100 ng/mL tunicamycin, or 4 nM bortezomib for 24 hr. Bar graph shows percentage of Annexin-V-positive cells ± SD of N = 3 independent biological experiments. *p<0.05, **p<0.01, ns, no statistical significance (t-test). (G) Keratinocytes isolated from littermate controls were treated with 4 nM bortezomib for 24 hr. Wild-type (Tgfbr2fl/fl), pre-oncogenic (HrasG12V; Tgfbr2fl/fl), and SCC keratinocytes (HrasG12V; Tgfbr2Δ/Δ) were compared. Bar graph shows percentage of Annexin-V-positive cells ± SD in four independent biological replicates. ***p<0.001 (t-test).
-
Figure 1—source data 1
Original immunoblots show the characterization of S51 and S51A squamous cell carcinoma (SCC) cell lines (related to Figure 1C).
- https://cdn.elifesciences.org/articles/77780/elife-77780-fig1-data1-v2.pdf
-
Figure 1—source data 2
Original immunoblots show phosphorylation status of eIF2α upon different stresses (related to Figure 1D).
- https://cdn.elifesciences.org/articles/77780/elife-77780-fig1-data2-v2.pdf
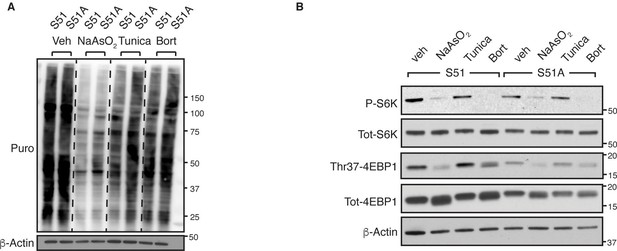
Effect on different stresses on protein synthesis rates and mTORC1 activation in integrated stress response (ISR)-competent and ISR-null cells.
(A) ISR-null cells show reduced capacity to activate the ISR as judged by global protein synthesis rate. No difference in protein synthesis rate was observed at steady state (vehicle-treated cells). ISR-WT and ISR-null cells are treated with 50 μM sodium arsenite (to induce oxidative stress), 100 ng/mL tunicamycin (to induce endoplasmic reticulum [ER] stress), or 100 nM bortezomib (to induce proteotoxic stress) for 6 hr followed by incubation with puromycin at 1 μM for 30 min and anti-puromycin immunoblot. (B) Representative immunoblot shows inhibition of mTORC1 pathway in both S51 and S51A cells upon stress. Cells were treated with either vehicle, 50 μM sodium arsenite, 100 ng/mL tunicamycin, or 100 nM bortezomib for 6 hr. Representative blots from two independent biological replicates are shown.
-
Figure 1—figure supplement 1—source data 1
Original immunoblots show protein synthesis rates upon different stresses (related to Figure 1—figure supplement 1A).
- https://cdn.elifesciences.org/articles/77780/elife-77780-fig1-figsupp1-data1-v2.pdf
-
Figure 1—figure supplement 1—source data 2
Original immunoblots show components of the mTORC1 pathway upon different stresses (related to Figure 1—figure supplement 1B).
- https://cdn.elifesciences.org/articles/77780/elife-77780-fig1-figsupp1-data2-v2.pdf
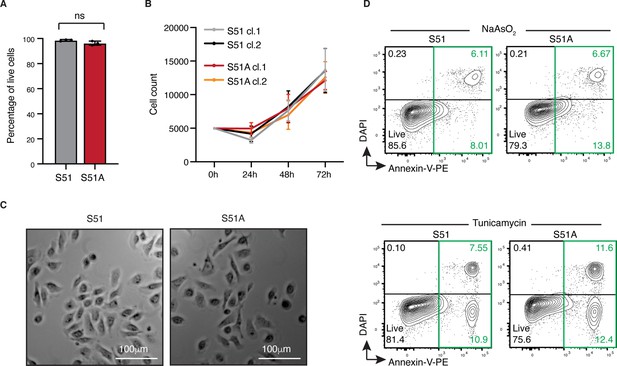
Integrated stress response (ISR)-null cells are more sensitive to stress but show no difference at steady state.
(A) Bar graph shows no difference in viability in control and ISR-null cells at steady state in 3 independent biological replicates. Error bars denote SD. ns, no statistical significance (t-test). (B) Quantification of cell count over time demonstrates no difference in proliferation rate between ISR-competent and ISR-null cells. Representation of N = 4 experiments and n = 16 total technical replicates per condition, error bars denote SD of pooled technical replicates. (C) Representative brightfield microscopy images of control and ISR-null cells show similar morphology in the absence of stress. (D) ISR-null cells are more sensitive to stress. Representative fluorescence-activated cell sorting (FACS) plot show increased Annexin-V staining in ISR-null cells after 24 hr treatment with 5 μM sodium arsenite or 100 ng/mL tunicamycin.
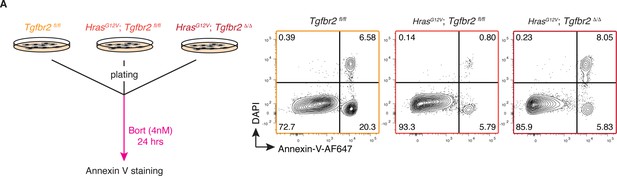
Transformed keratinocytes are less sensitive to proteotoxic stress.
(A) Schematic of the experiment. Briefly keratinocytes from healthy control (Tgfbr2fl/fl), pre-oncogenic (HrasG12V; Tgfbr2fl/fl), or fully transformed (HrasG12V; Tgfbr2Δ/Δ) skin were isolated and incubated with 4 nM bortezomib for 24 hr, before evaluating percentage of apoptotic cells. Right: representative fluorescence-activated cell sorting (FACS) plot illustrate percentages of Annexin-V and DAPI-positive cells.
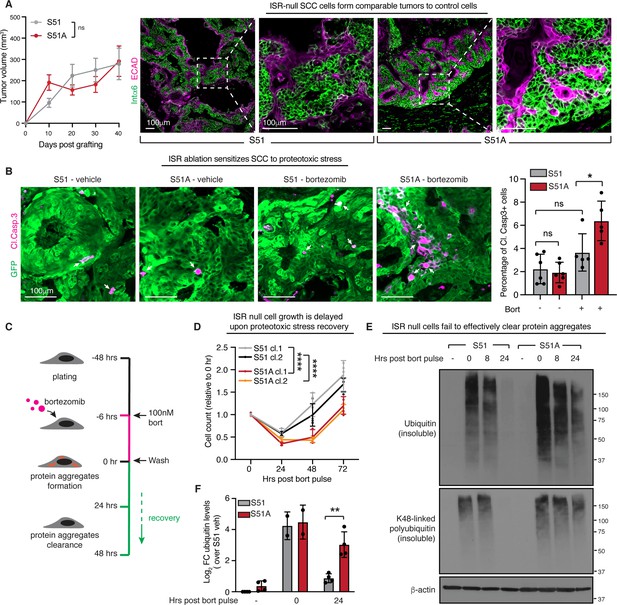
The integrated stress response (ISR) is required for efficient clearance of protein aggregates.
(A) Left: growth of tumor allografts derived from control or ISR-null squamous cell carcinoma (SCC) cells. Tumor volume is comparable between conditions. Error bars represent SEM from N = 16 tumors per condition. ns, no statistical significance (simple linear regression). Right: α6-integrin and E-cadherin immunofluorescence of SCC on day 17 reveals that ISR-ablation does not impact tumor morphology. Boxed regions are shown at higher magnification images to the right of each image. Scale bar 100 μm. (B) Cleaved caspase 3 immunofluorescence of tumor sections reveals elevated apoptosis, a sign of increased sensitivity of ISR-null SCC tumors to proteotoxic stress (bortezomib treatment 1.2 mg/kg i.p. on days 13, 15, and 17). No significant difference in viability is observed in vehicle-treated SCC tumors. White arrows indicate cleaved caspase 3-positive cells. Bar graph on the right shows percentage of cleaved caspase 3-positive cells ± SD within the GFP+ tumor cells, in at least five independent tumors. * p<0.05, ns, no statistical significance (one-way ANOVA, multiple comparisons). Scale bar 100 μm. (C) Schematic of protein aggregate pulse-recovery experiments. Cells are treated with a saturating (100 nM) dose of bortezomib for 6 hr, a timepoint at which protein aggregates have accumulated, but cells are still viable. Bortezomib is washed from the media and cells are then monitored during the recovery phase. (D) Cellular proliferation during the protein aggregate recovery phase indicates that control cells regain proliferative capacity 24 hr prior to ISR-null cells. N = 4 biological replicates, n = 16 total technical replicates, error bars denote SD of technical replicates. ****p<0.0001 (two-way ANOVA, multiple comparisons). (E) Protein aggregates evaluated by anti-ubiquitin and K48-linked polyubiquitin immunoblot following cellular fractionation of soluble and insoluble proteins. Representative immunoblot of the insoluble fraction shows clearance of aggregates in control but not ISR-null cells at 24 hr. (F) Bar graph shows log2 fold change (FC) ubiquitin levels ± SD normalized over β-actin and relative to vehicle-treated control cells in at least two independent biological experiments. **p<0.01 (t-test).
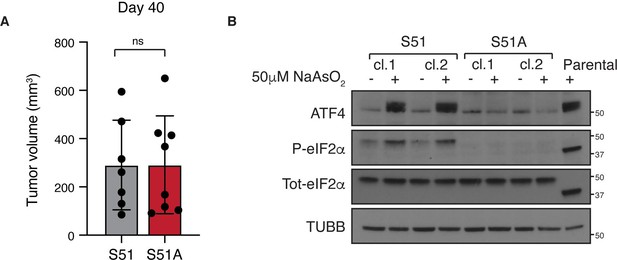
Characterization of integrated stress response (ISR)-null squamous cell carcinoma (SCC) tumors.
(A) Bar graph shows tumor volume quantification ± SD on day 40 in mice transplanted with either control or ISR-null SCCs. A minimum of seven tumors per condition were quantified. ns, no statistical difference (t-test). (B) Secondary ex vivo control and ISR-null SCC cell lines were generated by fluorescence-activated cell sorting (FACS) isolation of day 35 tumors initiated and grown from transplantation of our primary control and ISR-null SCC lines. p-eIF2α and ATF4 immunoblot following 4 hr treatment with 50 μM sodium arsenite shows that neither ISR-null clone (N = 2) has escaped ISR-ablation. This experiment rules out the trivial explanation that ISR-null SCC growth is due to ‘escaper’ cells that have reverted to a wild-type ISR state.
-
Figure 2—figure supplement 1—source data 1
Original immunoblots show phosphorylation status of eIF2α in cells isolated from allografted tumors (related to Figure 2—figure supplement 1B).
- https://cdn.elifesciences.org/articles/77780/elife-77780-fig2-figsupp1-data1-v2.pdf
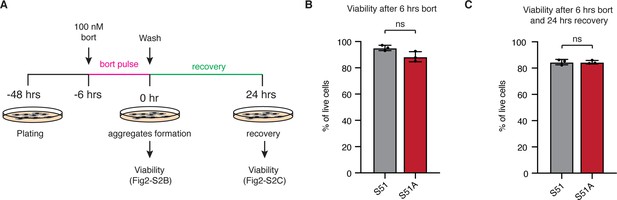
Characterization of bortezomib pulse and recovery system.
(A) Schematic illustrates experimental design for our bortezomib pulse and recovery system. Cells are treated for 6 hr with 100 nM bortezomib and let to recover for 24 hr. Viability is assessed at two timepoints (0 hr recovery and 24 hr recovery) (B) Integrated stress response (ISR)-null cells show no difference in viability after a short (6 hr) pulse of 100 nM bortezomib. Bar graph shows percentage of live cells ± SD quantified as DAPI-negative cells by fluorescence-activated cell sorting (FACS) in three independent experiments. ns, no statistical significance (t-test). (C) ISR-null cells show no difference in viability after a 24 hr recovery from a 6 hr pulse of 100 nM bortezomib. Bar graph shows percentage of live cells ± SD quantified as DAPI-negative cells by FACS in three independent experiments. ns, no statistical significance (t-test).
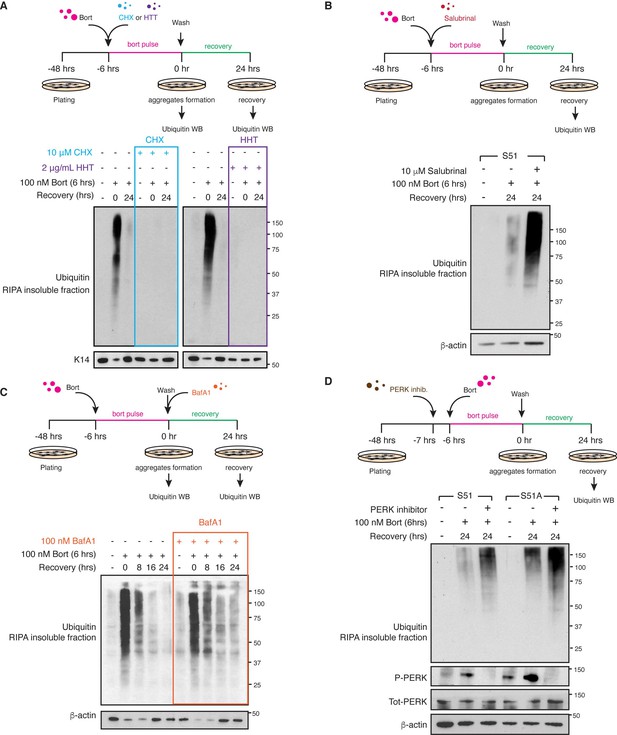
Characterization of protein aggregate source and clearance mechanism.
(A) Protein aggregates formation is dependent on newly synthesized proteins. Ubiquitin immunoblot in the radio-immunoprecipitation assay (RIPA)-insoluble fraction shows absence of protein aggregates in control cells either treated with 10 μM of the translation elongation inhibitor cycloheximide or 2 μg/mL of the translation initiation inhibitor harringtonine concomitantly with the bortezomib pulse. (B) Protein aggregates clearance is dependent on eIF2α dephosphorylation. S51 squamous cell carcinoma (SCC) cells were treated concomitantly with bortezomib and 10 μM salubrinal to inhibit eIF2α phosphatases. After 6 hr, cells were washed and let to recover for 24 hr in the presence of salubrinal. Representative immunoblot shows accumulation of protein aggregates in the RIPA-insoluble fraction of salubrinal-treated S51 cells. (C) Autophagy is only partially required to clear protein aggregates. Ubiquitin immunoblot in the RIPA-insoluble fraction shows partial accumulation of protein aggregates in the presence of 100 nM of the autophagy inhibitor bafilomycin A1. (D) PKR-like endoplasmic reticulum kinase (PERK) inhibition promotes aggregates formation in an integrated stress response (ISR)-independent manner. S51 and S51A cells were pretreated with 2.5 μM GSK2606414 for 1 hr prior to a 6 hr bortezomib pulse. Following wash, cells were let to recover for 24 hr in the presence of 2.5 μM GSK2606414. Representative immunoblot shows further increase in protein aggregates accumulation in both S51 and S51A cells.
-
Figure 2—figure supplement 3—source data 1
Original immunoblots show ubiquitin levels in the insoluble fraction of S51 cells treated with cycloheximide or harringtonine and following recovery from proteotoxic stress (related to Figure 2—figure supplement 3A).
- https://cdn.elifesciences.org/articles/77780/elife-77780-fig2-figsupp3-data1-v2.pdf
-
Figure 2—figure supplement 3—source data 2
Original immunoblots show ubiquitin levels in the insoluble fraction of S51 cells treated with salubrinal and following recovery from proteotoxic stress (related to Figure 2—figure supplement 3B).
- https://cdn.elifesciences.org/articles/77780/elife-77780-fig2-figsupp3-data2-v2.pdf
-
Figure 2—figure supplement 3—source data 3
Original immunoblots show ubiquitin levels in the insoluble fraction of S51 cells treated with bafilomycin A1 and following recovery from proteotoxic stress (related to Figure 2—figure supplement 3C).
- https://cdn.elifesciences.org/articles/77780/elife-77780-fig2-figsupp3-data3-v2.pdf
-
Figure 2—figure supplement 3—source data 4
Original immunoblots show ubiquitin levels in the insoluble fraction of S51 cells treated with PERK inhibitor and following recovery from proteotoxic stress (related to Figure 2—figure supplement 3D).
- https://cdn.elifesciences.org/articles/77780/elife-77780-fig2-figsupp3-data4-v2.pdf
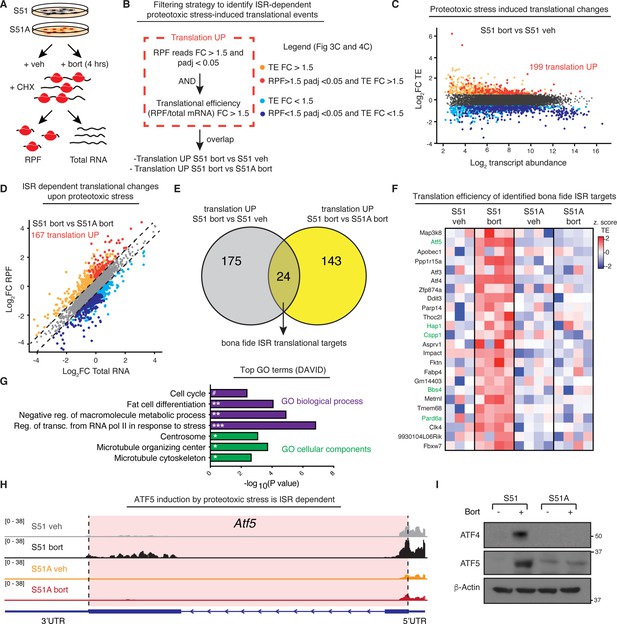
The integrated stress response (ISR) induces synthesis of centrosomal proteins in response to proteotoxic stress.
(A) Schematic of ribosome profiling experiment. N = 4 samples per condition of control or ISR-null cells were treated with or without 100 nM bortezomib for 4 hr. Cells were lysed in the presence of 100 μg/mL cycloheximide and total mRNA and ribosome-protected fragments (RPFs) were sequenced to analyze translational efficiency (TE) genome-wide. (B) Filtering strategy to identify a high-confidence list of translationally regulated mRNAs targeted by the ISR following protein aggregate stress. To identify translationally regulated mRNAs, a two-step approach was taken. First, genes with RPF of a fold change >1.5 and padj <0.05 (DESeq2 generalized linear model) were identified. Second, within this list, genes with TE fold change >1.5 were selected (red dots, panels C and D). Finally, ISR target genes are identified by the overlap of mRNAs with significantly upregulated translation in two comparisons: (1) ISR-competent-bort vs. ISR-competent-vehicle and (2) ISR-competent-bort vs. ISR-null-bort. (C) Dot plot shows TE log2 fold change vs. log2 mean transcript abundance genome-wide in ISR-competent-bort vs. ISR-competent-vehicle highlights both upregulated genes as well as a downregulated group of highly abundant transcripts (see legend in panel B). As expected, highly expressed housekeeping transcripts are translationally downregulated. (D) Dot plot shows RPF log2 fold change vs. total mRNA log2 fold change genome-wide in ISR-competent-bort vs. ISR-null-bort (see legend in panel B). (E) Pie chart shows high-confidence translational upregulated ISR targets. Overlap between translationally upregulated genes in (1) ISR-competent-bort vs. ISR-competent-vehicle and (2) ISR-competent-bort vs. ISR-null-bort is shown. (F) Z-scored heatmap of TEs for 24 genes translationally induced by the ISR. Centrosomal proteins are highlighted in green lettering. (G) Top Gene Ontology (GO) terms identified in categories of biological processes and cellular components using translationally induced genes by the ISR. Centrosomal proteins are enriched among cellular components. #p=0.061, *p<0.05, **p<0.01, ***p<0.001 (Benjamini test). (H) Representative RPF tracks for Atf5 reveal coding sequence translation are specifically induced in control cells treated with bortezomib. Atf5 coding sequence is shaded in red. (I) Representative immunoblot confirms ISR-dependent induction of ATF4 and ATF5 following protein aggregate stress.
-
Figure 3—source data 1
Original immunoblots show ATF5 and ATF4 level in S51 and S51A cells with or without proteotoxic stress (related to Figure 3I).
- https://cdn.elifesciences.org/articles/77780/elife-77780-fig3-data1-v2.pdf
-
Figure 3—source data 2
Raw read counts for ribosome profiling experiment.
- https://cdn.elifesciences.org/articles/77780/elife-77780-fig3-data2-v2.xlsx
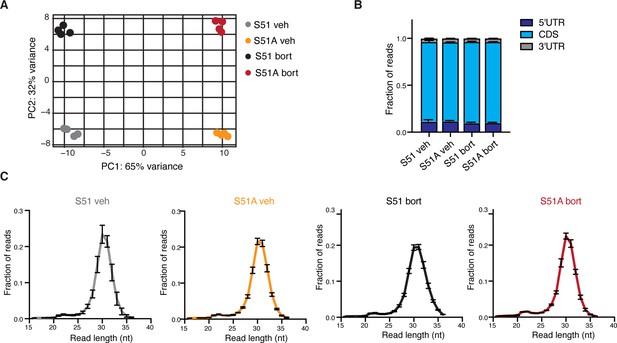
Quality control of ribosome profiling libraries.
(A) Principal component analysis (PCA) plot shows variance of four independent replicates. Two major sources of variance are identified in our dataset: S51 vs. S51A (x-axis) and vehicle vs. bortezomib (y-axis). (B) Metagene analysis shows fraction of ribosome-protected fragment (RPF) reads ± SD respectively aligned to 5′UTR, CDS, and 3′UTR in at least three independent biological replicates. (C) Histograms show fraction of RPF reads ± SD corresponding to their length in nucleotides in at least three independent biological replicates. As expected, the majority of reads are found between 29 and 30 nt.
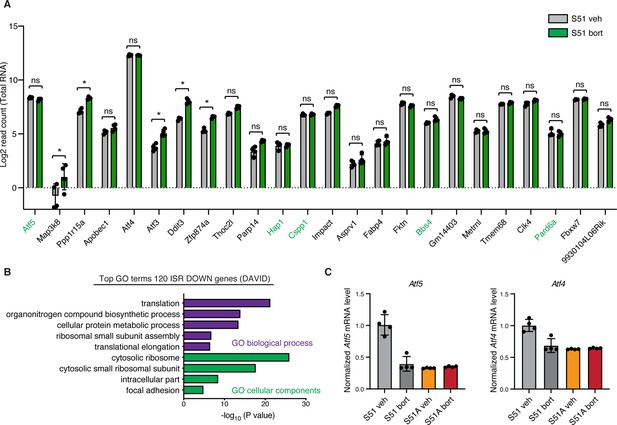
Ribosome occupancy changes occur in the absence of transcriptional changes.
(A) Bar graph shows log2 read counts of total RNA ± SD comparing vehicle and bortezomib-treated S51 cells in four independent biological replicates. While most mRNAs show no transcriptional differences (including all the identified centrosomal components), a few mRNAs show mild transcriptional upregulation (1 < log2FC < 2), while retaining significant increase in both ribosome occupancy (log2FC > 2.5 and padj < 0.01) and translational efficiency (log2 FC > 1). ns, no statistical difference, * log2FC > 1 and padj < 0.05 (DeSeq2). (B) Gene Ontology (GO) analysis shows top terms for biological processes and cellular components of 120 genes translationally downregulated by the integrated stress response (ISR). 120 genes are defined by the overlap of mRNAs with significantly downregulated translation in two comparisons: (1) ISR-competent-bort vs. ISR-competent-vehicle and (2) ISR-competent-bort vs. ISR-null-bort. (C) qPCR quantification of Atf5 and Atf4 mRNA levels ± SD in S51 and S51A cells either vehicle-treated or treated for 6 hr with bortezomib in four independent biological replicates. Data were normalized over Rps16.
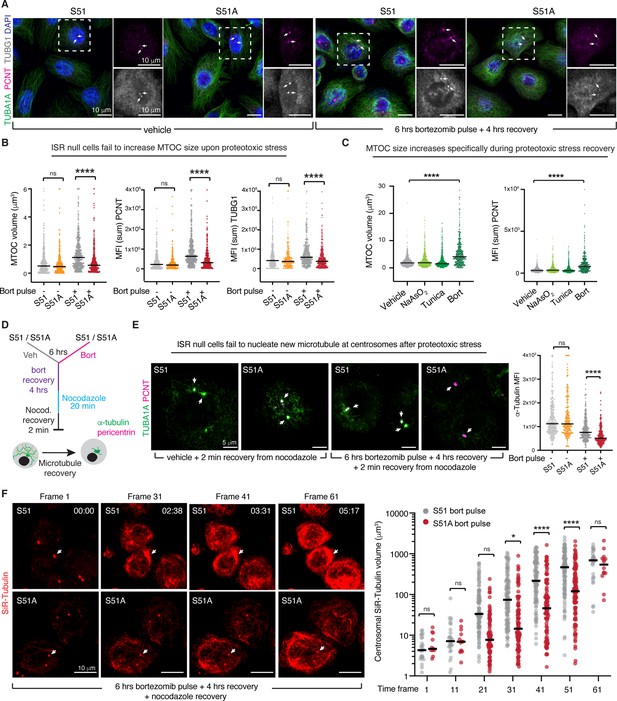
The integrated stress response (ISR) protects centrosomal microtubule dynamics during recovery from protein aggregate stress.
(A, B) Representative immunofluorescence images of S51 and S51A cells vehicle-treated or treated with a bortezomib pulse (100 nM, 6 hr) and let to recover for 4 hr. Immunolabeling is to highlight the centrosomal microtubule-organizing center (MTOC) (pericentrin, PCNT, and γ-tubulin, TUBG1), and the cytoplasmic microtubules (α-tubulin, TUBA1A). Scale bar 10 μm. White arrows indicate centrosomal MTOCs. (B) Both volume and mean sum fluorescence intensity of MTOC markers are increased specifically in control cells recovering from protein aggregate stress. All data are visualized on a per centrosome basis from N = 2 independent experiments. ****p<0.0001, ns, no statistical significance (two-way ANOVA with multiple comparisons). (C) S51 squamous cell carcinoma (SCC) cells were treated with either vehicle, 50 μM sodium arsenite, 100 ng/μL tunicamycin, or 100 nM bortezomib for 6 hr, followed by a recovery period of 4 hr. MTOC volume and mean sum fluorescence intensity are plotted for two independent biological experiments. ****p<0.0001 (two-way ANOVA with multiple comparisons). (D) Schematic of microtubule recovery assay to assess the capacity of centrosomes to nucleate new microtubules following 4 hr recovery from protein aggregate stress. Cells with or without protein aggregates are treated with 13 μM nocodazole for 20 min to depolymerize microtubules. Nocodazole is washed from the media, and new microtubules are allowed to form during a 2 min incubation at room temperature. Cells are fixed and MTOCs (PCNT+) and cytoplasmic microtubules (TUBA1A) are quantified and analyzed by immunofluorescence. (E) Representative images of centrosomal microtubule nucleation following nocodazole wash out in control and ISR-null cells with or without protein aggregates. Centrosomal microtubule nucleation is analyzed by measuring α-tubulin signal at the centrosome, which is defined as the pericentrin-positive volume. White arrows indicate centrosomal MTOCs. Scale bar 5 μm. Quantification of mean centrosomal α-tubulin intensity indicates that control cells are partially protected against impaired microtubule dynamics during stress. N = 2 independent experiments. ****p<0.0001, ns, no statistical significance (two-way ANOVA with multiple comparisons). (F) Still images from live-imaging experiments show time-dependent growth of microtubule at the centrosome following nocodazole removal. S51 and S51A SCC cells were treated with a bortezomib pulse and let to recover for 4 hr prior to nocodazole treatment. Quantification on the right shows volume of SiR-tubulin-labeled microtubules growing from the centrosome. Each dot represents one cell. Data from two independent biological experiments are plotted. *p<0.05, ****p<0.0001, ns, no statistical significance (two-way ANOVA with multiple comparisons). Scale bar 10 μm.
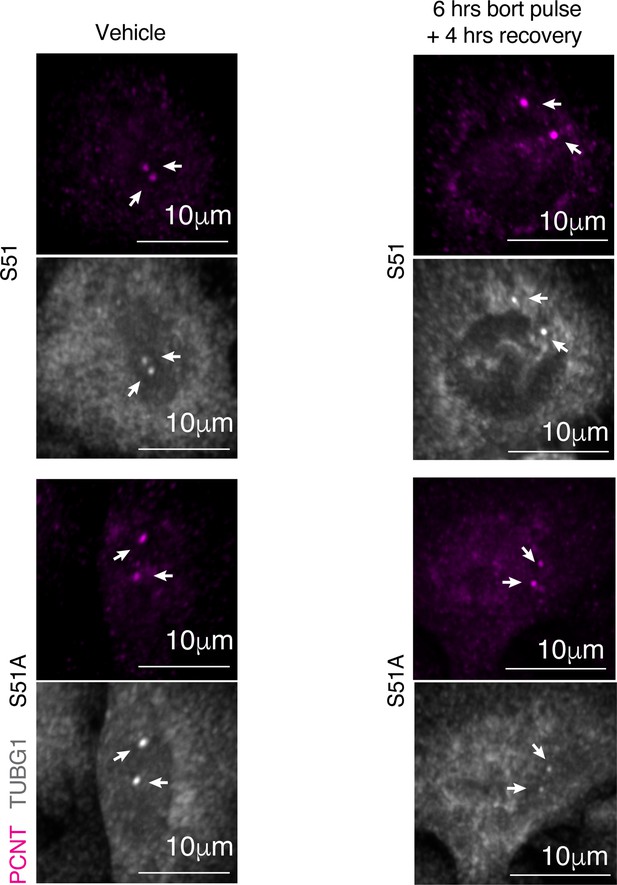
Increased centrosomal microtubule-organizing center (MTOC) size upon proteotoxic stress is dependent on the integrated stress response (ISR).
High-magnification images show centrosomal MTOC by pericentrin and γ-tubulin staining in S51 and S51A cells vehicle-treated or treated with a bortezomib pulse (100 nM, 6 hr) and let to recover for 4 hr. White arrows indicate MTOCs. Scale bar 10 μm.
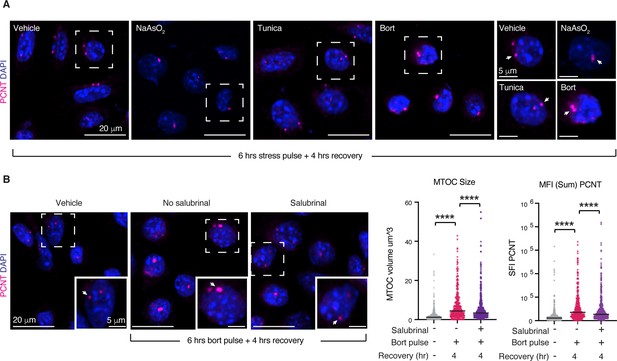
Increased centrosomal microtubule-organizing center (MTOC) size is restricted to proteotoxic stress and depends on eIF2α dephosphorylation.
(A) Representative immunofluorescence images show enlarged MTOC in bortezomib-treated cells and not in cells treated with sodium arsenite or tunicamycin. Cells were treated with either vehicle, 50 μM sodium arsenite, 100 ng/μL tunicamycin, or 100 nM bortezomib for 6 hr, followed by a recovery period of 4 hr. Scale bar 20 and 5 μm for inset magnification. (B) Immunofluorescence analysis of MTOC size in S51 cells treated with bortezomib and 10 μM salubrinal shows that MTOC enlargement depends on eIF2α dephosphorylation. Scale bar 20 and 5 μm for inset magnification. Quantification shows MTOC volume and mean sum fluorescence intensity for pericentrin in at least two independent biological replicates. ***p<0.001 (t-test).
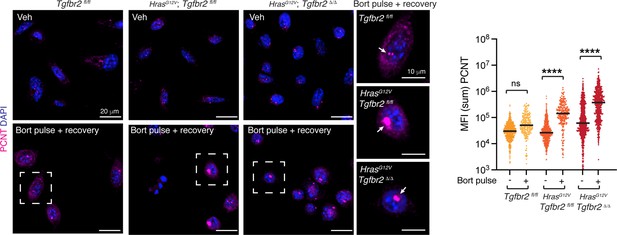
Increased centrosomal microtubule-organizing center (MTOC) size is unique to transformed keratinocytes.
Representative immunofluorescence images show MTOC staining in keartinocytes isolated from healthy control (Tgfbr2fl/fl), pre-oncogenic (HrasG12V; Tgfbr2fl/fl), or fully transformed (HrasG12V; Tgfbr2Δ/Δ) skin. Cells were incubated with 100 nM bortezomib for 6 hr followed by a 4 hr recovery. Scale bar 20 and 10 μm for inset magnification. Quantification shows mean sum fluorescence intensity for pericentrin in two independent biological replicates. ****p<0.0001, ns, no statistical significance (t-test).
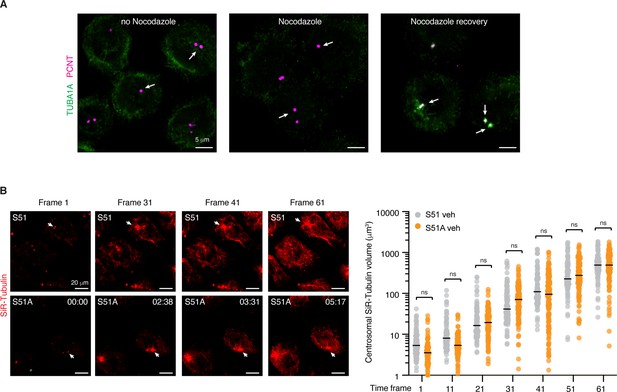
Characterization of nocodazole recovery experiment.
(A) Representative images demonstrate complete depolymerization of microtubules by nocodazole and nucleation of new centrosomal microtubules following nocodazole wash. White arrows indicate centrosomal microtubule-organizing centers (MTOCs). Scale bar 5 μm. (B) Still images from live-imaging experiments show time-dependent growth of microtubule at the centrosome following nocodazole removal. S51 and S51A squamous cell carcinoma (SCC) cells were treated with 13 μm nocodazole for 20 min, washed, and placed on fresh media prior to imaging. Quantification on the right shows volume of SiR-tubulin-labeled microtubules growing from the centrosome. Each dot represents one cell. Data from two independent biological experiments are plotted. ns, no statistical significance (two-way ANOVA with multiple comparisons). Scale bar 20 μm.
Microtubule growth is not affected in integrated stress response (ISR)-null cells at steady state.
Microtubule growth from the centrosome is delayed in integrated stress response (ISR)-null cells recovering from proteotoxic stress.
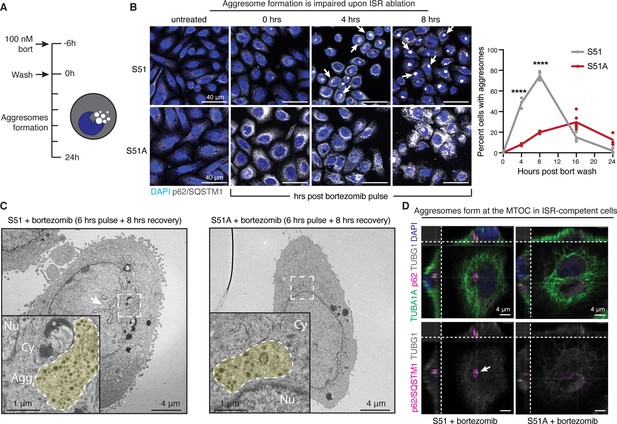
The integrated stress response (ISR) is required for aggresome formation.
(A) Schematic of aggresome formation assay. During recovery from proteotoxic stress, aggresome formation is assessed by anti-p62/SQSTM1 immunofluorescence, which appears as a well-defined, perinuclear puncta. (B) Time course of aggresome formation in control and ISR-null cells indicates diminished aggresome formation in cells with an ablated ISR. White arrows indicate aggresomes. Quantification of the percentage of aggresome-positive cells. N = 2 independent experiments with at least 145 cells quantified per condition. Error bars represent 95% confidence interval calculated by Wilson’s method for confidence interval of a proportion. ****p<0.001 using two-sample Z-test for a proportion (comparisons: control vs. ISR-null at 4 and 8 hr). Scale bar 40 μm. (C) Aggresomes visualized at the ultrastructural level by transmission electron microscopy. In control cells, aggresomes characteristically appear as a perinuclear cluster of electron-dense granules and partially degraded organelles that often deform the nuclear membrane. Fewer aggresomes form in ISR-null cells, and those that do are smaller, more diffuse and rarely indent the nucleus. White arrow indicates nuclear envelope deformation. Scale bar 4 μm. Yellow shade in the zoomed-in images at the lower left highlights aggresomes and protein aggregates. Nu, nucleus. Cy, cytoplasm, Agg, aggresome. *Lipid droplet. Scale bar 1 μm. (D) Confocal immunofluorescence microscopy and 3D reconstruction to visualize the location of the aggresome in relation to the microtubule-organizing center (MTOC) (γ-tubulin, TUBG1) and cytoplasmic microtubules (α-tubulin, TUBA1A). In control cells, aggresomes appear as dense p62-positive structures directly adjacent to the MTOC puncta. Representative cells from 8 hr post-bortezomib washout are shown. White arrow indicates MTOC. Scale bar 4 μm.
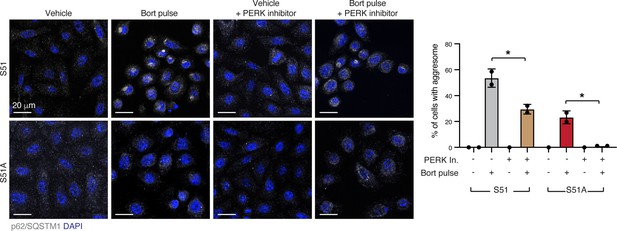
PKR-like endoplasmic reticulum kinase (PERK) inhibition inhibits aggresome formation independently from the integrated stress response (ISR).
Immunofluorescence images show reduced aggresome (p62 puncti) formation in S51 and S51A cells treated with 2.5 μM GSK2606414. Briefly, S51 and S51A cells were pretreated with GSK2606414 for 1 hr prior to a 6 hr bortezomib pulse. Following wash, cells were let to recover for 6 hr in the presence of GSK2606414. Bar graph shows percentage of cells with aggresomes in two independent biological replicates. *p<0.05 (ANOVA with multiple comparisons). Scale bar 20 μm.
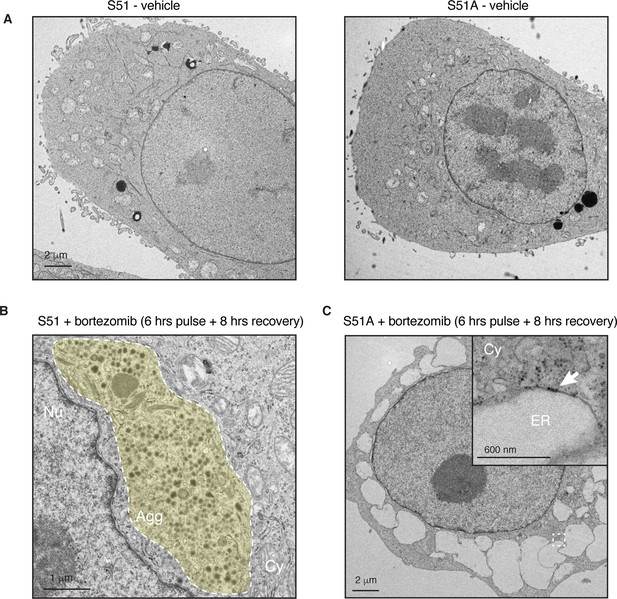
The integrated stress response (ISR) promotes aggresome formation upon recovery from proteotoxic stress.
(A) Transmission electron microscopy shows no difference at steady state between ISR-competent and ISR-null cells. No aggresomes are observed in the absence of bortezomib. Scale bar 2 μm. (B) Transmission electron microscopy shows aggresomes, which form in S51 cells during recovery from proteotoxic stress. Aggresomes are highlighted by a yellow shadow. As expected, aggresomes are perinuclear structures distorting the nucleus. Nu, nucleus. Cy, cytoplasm. Agg, aggresome. Scale bar 1 μm. (C) Transmission electron microscopy reveals swollen endoplasmic reticulum (ER) that is present in some of the ISR-null cells following proteotoxic stress. These cells are viable and do recover from stress, but their presence is indicative of ER stress and unfolded protein that accumulate in the ER as well as the cytoplasm. White arrows indicate ribosomes attached to the ER membranes. Scale bar 2 μm.
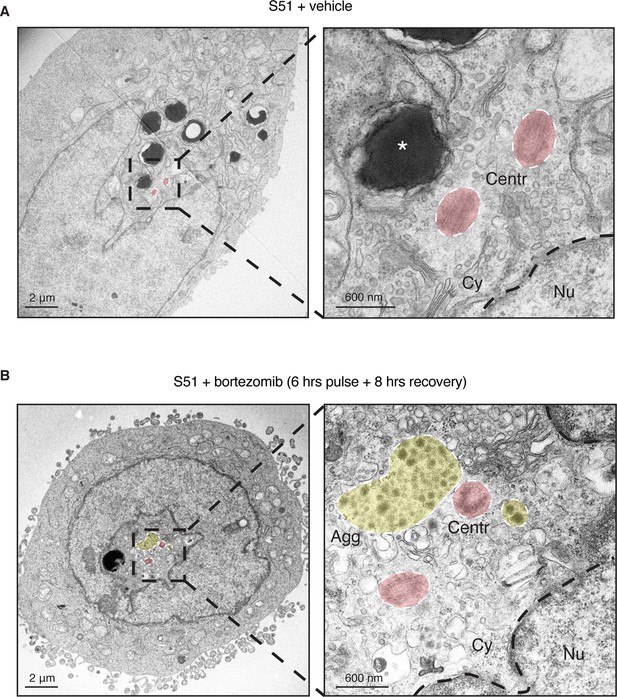
Aggresomes accumulate at the centrosome upon recovery from proteotoxic stress.
(A) Transmission electron microscopy shows centrosomes in vehicle-treated S51 squamous cell carcinoma (SCC) cells. Centrosomes are highlighted by a red shadow. Nu, nucleus. Cy, cytoplasm. Centr, centrosome, * lipid droplet. Scale bar 2 μm and 600 nm. (B) Transmission electron microscopy shows accumulation of aggresomes at the centrosomes upon recovery from proteotoxic stress. S51 SCC cells treated with 100 nm bortezomib followed by 8 hr recovery period are shown. Aggresomes are highlighted by a yellow shadow. Centrosomes are highlighted by a red shadow. Scale bar 2 μm and 600 nm.
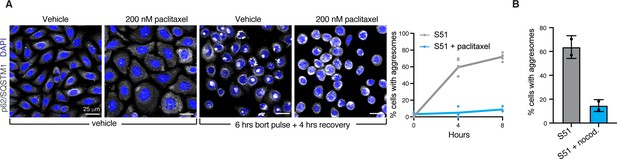
Aggresome formation is dependent on microtubule dynamics.
(A) Formation of aggresomes is dependent on microtubule-mediated transport. Blocking microtubule dynamics by using 200 nM of paclitaxel inhibits aggresome formation. Graph shows percentage of cells with aggresomes as quantified by immunofluorescence in two independent biological experiments. (B) Aggresomes formation is dependent on microtubule-mediated transport. Microtubule polymerization was blocked adding 13 μM nocodazole at the time of bortezomib washout. Percentage of cells with aggresomes ± SD is quantified in two independent biological replicates.
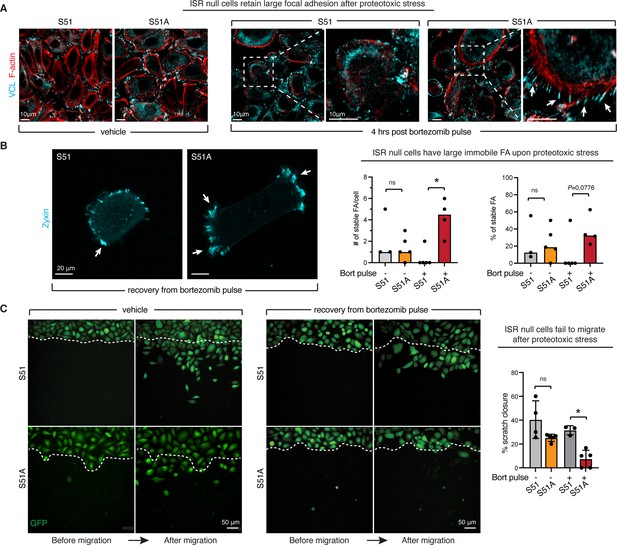
The integrated stress response (ISR) is required for focal adhesion remodeling and cell migration upon proteotoxic stress.
(A) Impaired focal adhesion dynamics in ISR-null cells. Immunofluorescence for vinculin and F-actin shows that during recovery from protein aggregate stress the ISR promotes the remodeling of focal adhesions. White arrows show characteristic long cellular extensions connected to enlarged focal adhesions in the ISR-null but not control cells recovering from protein aggregate stress. Consistent with the known role of microtubules in targeting focal adhesion turnover, these features resemble those of keratinocytes lacking microtubule-focal adhesion targeting proteins (Wu et al., 2008). Scale bar 10 μm. (B). Static frames from live imaging of zyxin-iRFP cells. White arrows show large (10 μm3 or greater) and stable (over the whole duration of imaging period) focal adhesion clusters. Bar graphs show total number of stable and large focal adhesion clusters per cell (left) and percentage of stable over total focal adhesion clusters (right) over a 14 min live-imaging time course. 3–5 cells were imaged per condition in two independent biological replicates. *p<0.05, ns, no statistical significance (t-test). (C) Live imaging of GFP-positive cells migrating in a scratch assay reveals loss of migratory capacity in ISR-null cells recovering from protein aggregate stress (6 hr of 100 nM bortezomib followed by wash). Scratch closure is quantified during an 8 hr interval, in N = 2 independent experiments with n = 3–5 technical replicates per condition. Bar graph shows percentage of scratch closure ± SD. *p<0.05, ns, no statistical significance (one-way ANOVA with multiple comparisons).
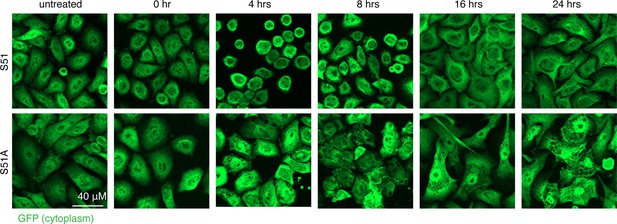
The integrated stress response (ISR) promotes dramatic morphological changes in response to proteotoxic stress.
ISR-competent or ISR-null cells are visualized via immunofluorescence by anti-GFP staining. Stress response in ISR-competent cells in vitro is characterized by a dramatic transient change in cell morphology, which is not observed in ISR-null cells.
Focal adhesion dynamics in integrated stress response (ISR)-competent (S51) or ISR-null S51A squamous cell carcinoma (SCC) cells in untreated conditions or upon recovery from proteotoxic stress.
Scratch assay in integrated stress response (ISR)-competent (S51) squamous cell carcinoma (SCC) cells in untreated conditions or upon recovery from proteotoxic stress.
Scratch assay in integrated stress response (ISR)-null (S51A) squamous cell carcinoma (SCC) cells in untreated conditions or upon recovery from proteotoxic stress.
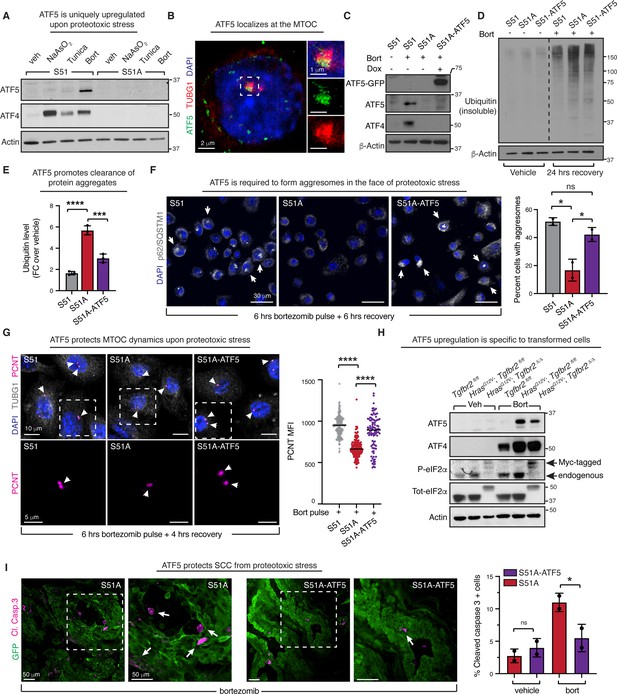
Integrated stress response (ISR)-induced ATF5 protects microtubule dynamics and promotes clearance of protein aggregates.
(A) ATF5 is selectively upregulated upon proteotoxic stress in an ISR-dependent fashion. S51 and S51A were treated with either vehicle, 50 μM sodium arsenite, 100 ng/μL tunicamycin, or 100 nM bortezomib for 6 hr. Representative blots from three independent experiment are shown. (B) Confocal microscopy confirms previously reported ATF5 localization at the microtubule-organizing center (MTOC). Insets on the right show higher magnification images with merged (top) and ATF5 (middle) and γ-tubulin (bottom) separately. (C) Immunoblot confirms ATF5 expression upon Dox administration (1 μg/mL for 24 hr) in proteotoxically stressed S51A-ATF5 cells where endogenous ATF5 translation is suppressed. (D) ATF5 expression partially rescues the ability of proteotoxically stressed ISR-null cells to clear ubiquitinated proteins. Representative immunoblot (of three independent biological replicates) shows level of ubiquitinated proteins in the radio-immunoprecipitation assay (RIPA)-insoluble fraction of S51, S51A, and S51A-ATF5 squamous cell carcinoma [SCC] cells in the absence or proteotoxic stress or following 24 hr recovery from a 6 hr bortezomib pulse. (E) Quantifications of experiments in (D). Bar graph shows ubiquitin levels ± SD at 24 hr recovery normalized over actin and relative to ubiquitin levels in untreated cells in three independent biological replicates. *p<0.05, ns, no statistical significance (one-way ANOVA with multiple comparisons). (F) ATF5 promotes aggresome formation. Aggresomes are visualized by p62/SQSTM1 immunofluorescence in SCC cells treated with a 6 hr bortezomib pulse followed by a 4 hr recovery period. White arrows point to aggresomes. Scale bar 30 μm. Bar graph shows percentage of cells with aggresomes ± SD in two independent biological replicates. Approximately 300 cells were quantified per each experiment. *p<0.05, ns, no statistical significance (one-way ANOVA with multiple comparisons). (G) ATF5 protects centrosomal MTOC dynamics. Centrosomal MTOC is visualized by pericentrin and γ-tubulin immunofluorescence. Cells were treated with bortezomib for 6 hr and let to recover for 4 hr. Scale bar 10 μm. Bottom row shows higher magnification of the area highlighted in top row. White arrows indicate MTOCs. Scale bar 5 μm. Bar graph shows mean fluorescence intensity for pericentrin in two independent biological replicates. ****p<0.0001 (one-way ANOVA with multiple comparisons). (H) Representative immunoblot shows selective upregulation of ATF5 upon bortezomib in transformed keratinocytes. Cells were treated with either vehicle or 100 nM bortezomib for 6 hr. Representative blot of three independent experiment is shown. (I) ATF5 induction protects ISR-null cells from protein aggregates-induced cytotoxicity. Immunofluorescence shows SCC tumors (GFP+) after treatment with bortezomib (1.2 mg/kg i.p.). Cell death is quantified by cleaved caspase 3-positive cells. White arrows point to cleaved caspase 3-positive cells. Scale bar 50 μm. Bar graph shows percentage of cleaved caspase 3-positive cells per tumor ± SD in two independent biological replicates. *p<0.05, ns, no statistical significance (one-way ANOVA with multiple comparisons).
-
Figure 7—source data 1
Original immunoblots show ATF5 and ATF4 level in S51 and S51A cells upon different stresses (related to Figure 7A).
- https://cdn.elifesciences.org/articles/77780/elife-77780-fig7-data1-v2.pdf
-
Figure 7—source data 2
Original immunoblots show ATF5 and ATF4 level in S51A cells overexpressing exogenous ATF5 (related to Figure 7C).
- https://cdn.elifesciences.org/articles/77780/elife-77780-fig7-data2-v2.pdf
-
Figure 7—source data 3
Original immunoblots show ubiquitin levels in S51A cells overexpressing exogenous ATF5 (related to Figure 7D).
- https://cdn.elifesciences.org/articles/77780/elife-77780-fig7-data3-v2.pdf
-
Figure 7—source data 4
Original immunoblots show ATF5 and ATF4 level in indicated genotypes with and without proteotoxic stress (related to Figure 7H).
- https://cdn.elifesciences.org/articles/77780/elife-77780-fig7-data4-v2.pdf
-
Figure 7—source data 5
Raw numerical data for all graphical representation (related to Figures 1—7).
- https://cdn.elifesciences.org/articles/77780/elife-77780-fig7-data5-v2.xlsx
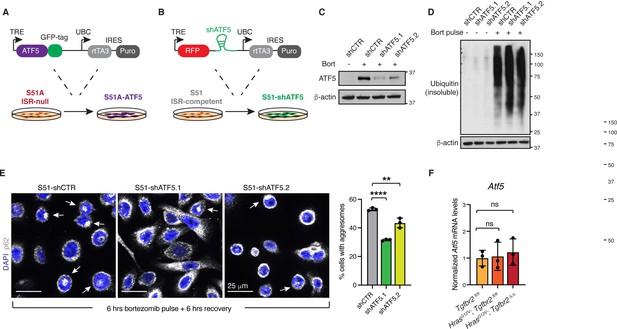
ATF5 is needed for efficient protein aggregate clearance.
(A) Schematic representation of rescue strategy to express a doxycycline-inducible GFP-tagged ATF5 in integrated stress response (ISR)-null squamous cell carcinoma (SCC) cells. (B) Schematic representation of knockdown strategy to inducibly reduce ATF5 levels in ISR-competent SCC cells. (C) Validation of ATF5 knockdown ISR-competent cells. Immunoblots shows ATF5 levels in cells pretreated with 1 μg/μL doxycycline for 48 hr and then treated with 100 nM bortezomib for 6 hr. (D) Immunoblot shows level of ubiquitinated protein aggregates in the radio-immunoprecipitation assay (RIPA)-insoluble fraction of indicated genotypes pretreated with doxycycline for 48 hr, treated with a bortezomib pulse for 6 hr and recovered for 24 hr. (E) Immunofluorescence analysis shows reduced aggresome formation (p62 puncti) in S51 cells knockdown for ATF5. Bar graph shows percentage of cells with aggresomes ± SD in two independent biological experiments. **p<0.01, ****p<0.0001 (ANOVA with multiple comparisons). (F) qPCR analysis shows Atf5 mRNA levels normalized over Rps16 ± SD in keratinocytes isolated from indicated genotypes in three independent biological replicates. ns, no statistical significance (ANOVA with multiple comparisons).
Tables
Reagent type (species) or resource | Designation | Source or reference | Identifiers | Additional information |
---|---|---|---|---|
Cell line (Mus musculus) | S51 | This paper | - | ISR-competent (HRasG12V TgfbrII D/D) |
Cell line (M. musculus) | S51A | This paper | - | ISR-null (HRasG12V TgfbrII D/D) |
Cell line (M. musculus) | TgfbrII fl/fl | Yang et al., 2015 | - | Keratinocytes |
Cell line (M. musculus) | HRasG12V TgfbrII fl/fl | Yang et al., 2015 | - | Keratinocytes |
Cell line (M. musculus) | HRasG12V TgfbrII D/D | Yang et al., 2015 | - | Keratinocytes |
Recombinant DNA reagent | pLKO-PGK-eIF2a(S51)-Myctag-P2A-NeoR | This paper | - | LV construct to overexpress WT eIF2a |
Recombinant DNA reagent | pLKO-PGK-eIF2a(S51A)-Myctag-P2A-NeoR | This paper | - | LV construct to overexpress ISR-null (S51A) eIF2a |
Recombinant DNA reagent | pLKO-TRE-ATF5-GFPtag_UBC-rtTA3-IRES-PuroR | This paper | - | LV construct to overexpress ATF5 |
Recombinant DNA reagent | pLKO-TRE-RFP-shATF5.1 UBC-rtTA3-IRES-PuroR | This paper; original Fellmann et al., 2013 | - | LV construct to inducibly knockdown ATF5 |
Recombinant DNA reagent | pLKO-TRE-RFP-shATF5.2 UBC-rtTA3-IRES-PuroR | This paper; original Fellmann et al., 2013 | - | LV construct to inducibly knockdown ATF5 |
Antibody | P-eIF2α (rabbit polyclonal) | Invitrogen | 44-728G | 1:1000 |
Antibody | Ubiquitin (rabbit polyclonal) | CST | 58395 | 1:1000 |
Antibody | ATF5 (mouse monoclonal) | Santa Cruz | Sc377168 | 1:1000 |
Antibody | ATF4 (rabbit monoclonal) | CST | 11815 | 1:1000 |
Antibody | p62 (rabbit monoclonal) | CST | 7695 | 1:250 |
Antibody | PCNT (rabbit polyclonal) | Abcam | Ab4448 | 1:250 |
Antibody | ATF5 (rabbit monoclonal) | Abcam | Ab184923 | 1:200 |
Antibody | Cl Casp 3 (rabbit polyclonal) | CST | 9661 | 1:200 |
Antibody | α-Tubulin (rabbit monoclonal) | Bio-Rad | MCA77G | 1:500 |
Antibody | β-Actin (mouse monoclonal) | CST | 3700 | 1:1000 |
Antibody | Puromycin (mouse monoclonal) | MilliporeSigma | MABE343 | 1:1000 |
Commercial assay or kit | RiboCop v2 | Lexogen | 144 | |
Commercial assay or kit | SiR-tubulin | Spirochrome | SC002 | |
Sequence-based reagent | eIF2a gRNA | Target sequence ATATTCCAACAAGCTGACAT | IDT | |
Peptide, recombinant protein | Cas9 | IDT | 1081058 | |
Chemical compound, drug | Sodium arsenite | VWR | BJ35000 | |
Chemical compound, drug | Tunicamycin | Sigma-Aldrich | T7765 | |
Chemical compound, drug | Bortezomib | Calbiochem | T7765 | 5043140001 |
Chemical compound, drug | Cyclohexamide | MilliporeSigma | T7765 | C7698 |
Chemical compound, drug | Rhodamine phalloidin | MilliporeSigma | R415 | |
Commercial assay or kit | Annexin-V PE | Thermo Fisher | A35111 | |
Commercial assay or kit | Annexin-V AF647 | Thermo Fisher | A23204 | |
Chemical compound, drug | Salubrinal | Sigma | SML0951 | |
Chemical compound, drug | PERK Inhibitor I, GSK2606414 | Sigma | 516535 | |
Chemical compound, drug | Bafilomycin A1 from Streptomyces griseus | MilliporeSigma | B1793 | |
Chemical compound, drug | Nocodazole | Sigma | M1404 | |
Chemical compound, drug | Paclitaxel | MilliporeSigma | T7191 |