Highly efficient generation of isogenic pluripotent stem cell models using prime editing
Figures
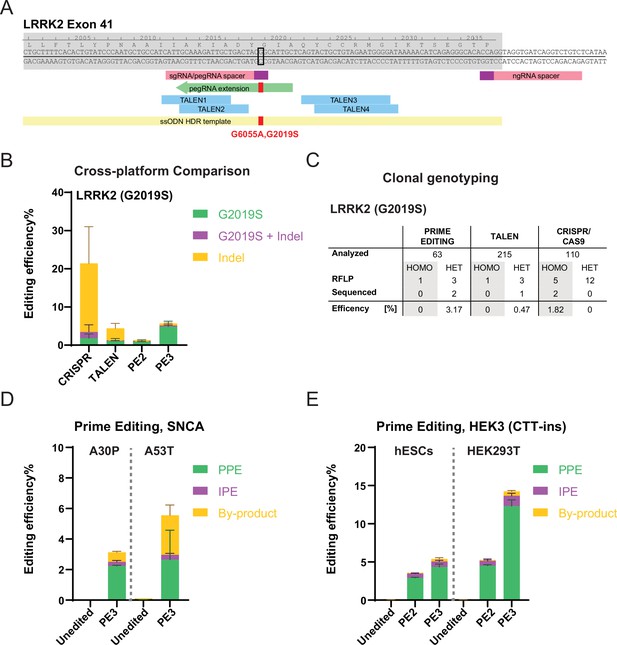
Systematic comparison of CRISPR/Cas9, transcription activator-like effector nucleases (TALEN), and prime editing (PE)-based genome editing efficiencies in human embryonic stem cells (hESCs) using plasmid-based delivery.
(A) Schematic depicting genome editing strategies to generate the leucine rich repeat kinase 2 (LRRK2; G2019S[G6055A]) mutation. Exon, gray shade; prospacers for CRISPR/Cas9 and PE, pink boxes; protospacer adjacent motif (PAM) sequences, purple boxes; representative PE guide RNA (pegRNA) extension, green arrow; TALEN recognition sites, blue boxes; single-stranded oligodeoxyribonucleotide (ssODN) homology-directed repair (HDR) template for CRISPR/Cas9 targeting, yellow box; the nucleotide to mutate, black open square; intended mutation, red filled square. (B) Comparison of bulk genome editing outcomes between CRISPR/Cas9, TALEN, and PE to insert the LRRK2 (G2019S) mutation (aggregated analysis using four different pegRNA designs and two TALEN pairs), N=2 for CRISPR/Cas9, N=3 for TALEN, N=2 for PE2, and N=6 for PE3. For individual analysis see Figure 1—figure supplement 1. (C) A summary of clonal genotyping comparing different genome editing strategies indicating the number and editing efficiency of single cell-derived clones carrying the correct heterozygous (HET) or homozygous (HOMO) G2019S substitution as identified by restriction fragment length polymorphism analysis (RLFP) followed by Sanger sequencing to excluded additional insertions or deletions (indels). (D) Generating α-Synuclein (SNCA) familiar Parkinson’s disease (PD) mutations, A30P, and A53T, using PE. pure prime editing (PPE), precise PE; IPE, impure PE; by-product, other indels. A30P, N=3; A53T, N=2. (E) Comparison of bulk PE outcomes on HEK3 (CTT-insertion) edits between hESCs and HEK293T cells. Color scheme, same as (D). N=3. (Error bars indicate the SD, N=number of biological replicates).
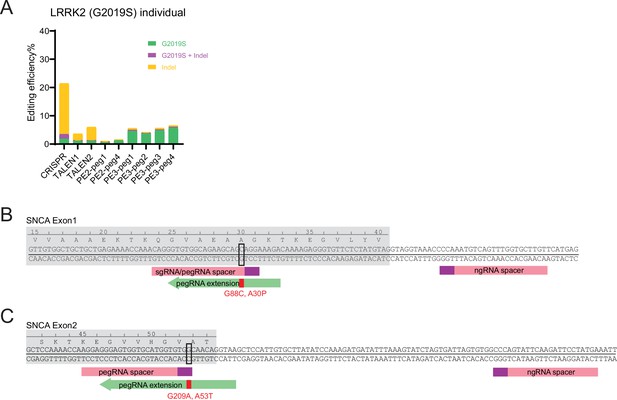
Schematics of α-Synuclein (SNCA) Parkinson’s disease (PD) mutation prime editing (PE) strategies and detailed leucine rich repeat kinase 2 (LRRK2) genome editing outcomes.
(A) Comparison of bulk genome editing outcomes between CRISPR, two transcription activator-like effector nucleases (TALEN) pairs, and four PE designs on LRRK2 (G2019S). Individual analysis of aggregated data is displayed in Figure 1B. CRISPR, N=2; TALEN pair#1, N=2; TALEN pair#2, N=1; PE2-peg1, N=1; PE2-peg4, N=1; PE3-peg1, N=2; PE3-peg2, N=1; PE3-peg3, N=1; PE3-peg4, N=2. (N=number of biological replicates). (B) Schematic of PE strategy for SNCA (A30P) mutation. Exon, gray shade; protospacers in PE, pink boxes; protospacer adjacent motif (PAM) sequences, purple boxes; PE guide RNA (pegRNA) extension, green arrow. (C) Schematic of PE strategy for SNCA (A53T) mutation. Color scheme, same as in B.
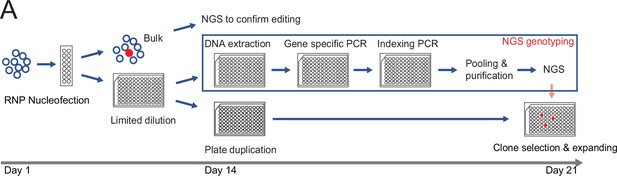
A high throughput human pluripotent stem cells (hPSCs) genome editing pipeline combining limited dilution and next generation sequencing (NGS) genotyping.
Nucleofected hPSCs are directly seeded into 96-well plates by limiting dilution. Upon colonies growing up, plates are duplicated to allocate one plate for cell maintenance and one plate for NGS genotyping. Clones with the desired genotype are expanded to establish genome-edited hPSCs line.
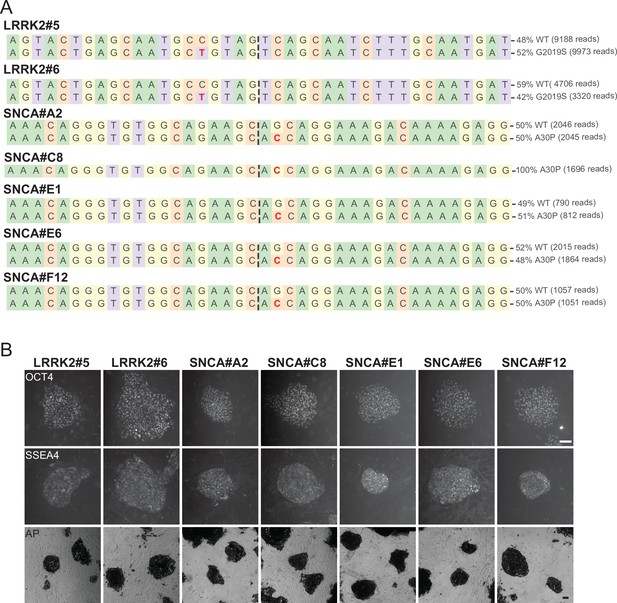
Genotyping and pluripotent marker staining of genome-edited human embryonic stem cells (hESCs) lines.
(A) Next generation sequencing (NGS) genotyping of the established hESCs lines harboring leucine rich repeat kinase 2 (LRRK2; G2019S) and α-Synuclein (SNCA; A30P) mutations, introduced by prime editing (PE) with plasmid-based delivery. The mutated nucleotides are highlighted in red. (B) Pluripotent marker staining of the edited hESCs lines shown in A for POU class 5 homeobox 1 (OCT4), stage-specific embryonic antigen-4 (SSEA4), and alkaline phosphatase (AP). Scale bar = 100 µm.
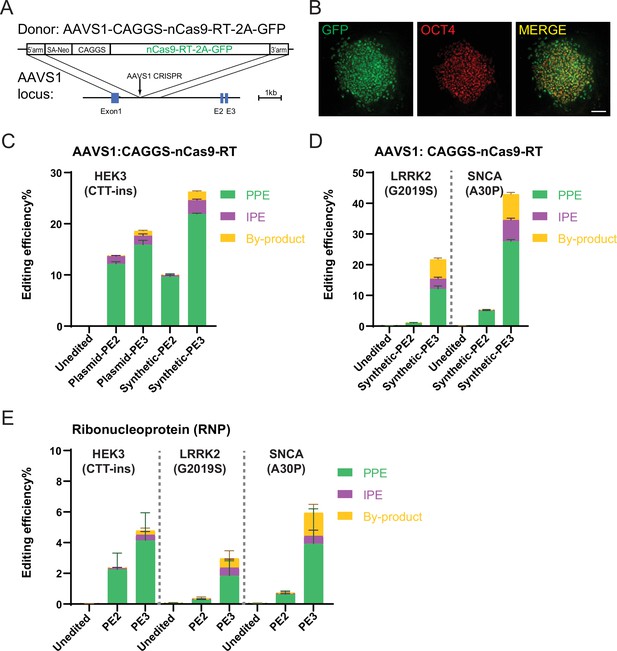
Prime editing (PE) in human embryonic stem cells (hESCs) expressing Cas9-nickase fused to a reverse transcriptase (nCas9-RT) protein from the AAVS1 safe harbor or delivered as RNP.
(A) Schematic of the genome editing strategy to knock-in nCas9-RT-2A-GFP (PE2 version of the prime editor protein as described in Anzalone et al., 2019) into the AAVS1 locus. (B) Expression of green fluorescent protein (GFP) and immunostaining of OCT4 on hESCs with nCas9-RT-2A-GFP knock-in. Scale bar = 100 µm. (C) Comparison of bulk PE outcomes between plasmid-expressed PE guide RNAs (pegRNAs)/nicking guide RNAs (ngRNAs) and synthetic pegRNAs/ngRNAs on HEK3 (CTT-insertion) edits in hESCs with nCas9-RT-2A-GFP knock-in. N=3. (D) Bulk PE outcomes on leucine rich repeat kinase 2 (LRRK2; G2019S) and α-Synuclein (SNCA; A30P) edits using synthetic pegRNAs/ngRNAs in hESCs with nCas9-RT-2A-GFP knock-in. N=3. (E) Bulk PE outcomes from RNP delivery on HEK3 (CTT-insertion), LRRK2 (G2019S), and SNCA (A30P) edits in human pluripotent stem cells (hPSCs). N=6. (Error bars indicate the SD, N=number of biological replicates).
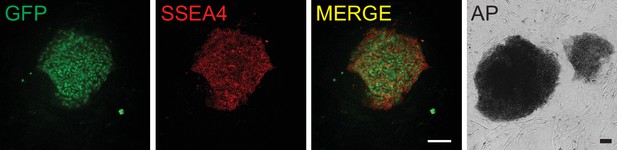
Pluripotent marker staining of human embryonic stem cells (hESCs) expressing Cas9-nickase fused to a reverse transcriptase (nCas9-RT) protein from the AAVS1 safe harbor locus.
Green fluorescent protein (GFP) expression, SSEA4 immunostaining and alkaline phosphatase staining on hESCs with nCas9-RT-2A-GFP knock-in. Scale bar = 100 µm.
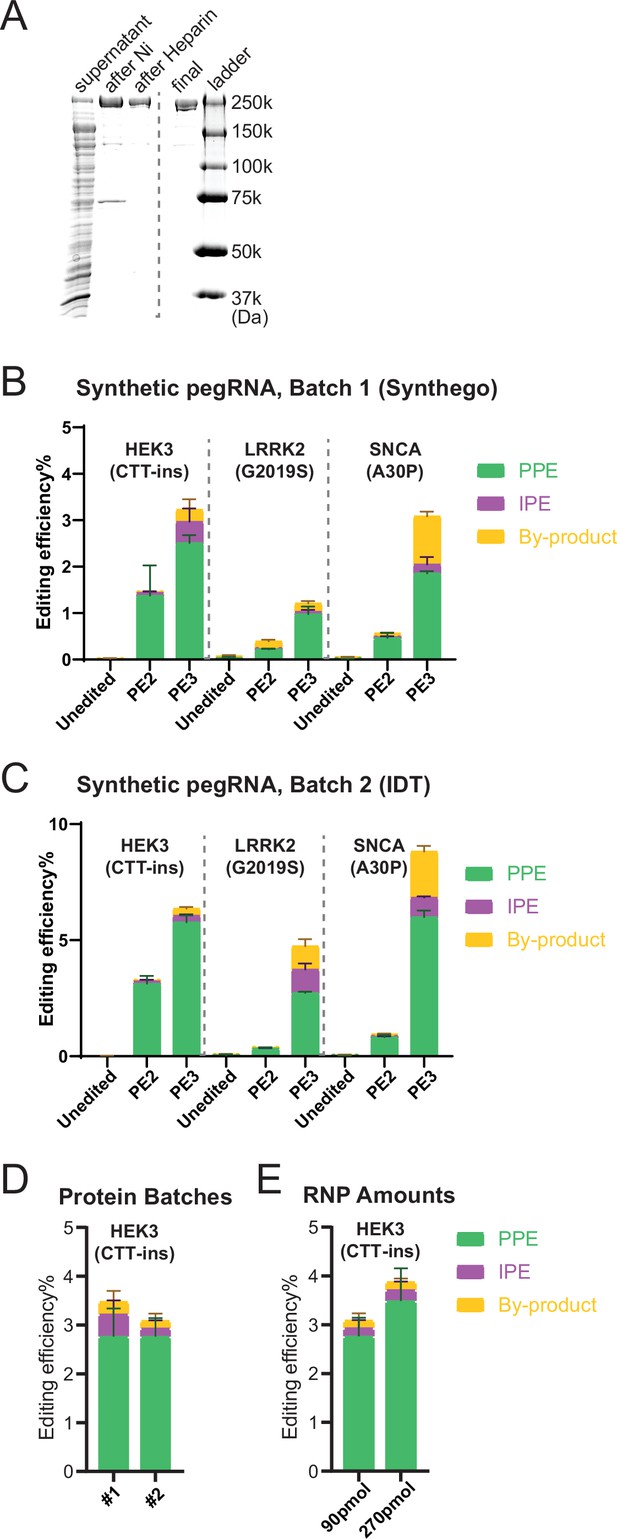
Quality control and parameter testing of RNP-based prime editing (PE).
(A) Coomassie blue staining at each step during the Cas9-nickase fused to a reverse transcriptase (nCas9-RT) protein purification (PE2 version of the prime editor protein as described in Anzalone et al., 2019). (B) Bulk PE outcomes of RNP-based delivery on three mutations in human embryonic stem cells (hESCs) with indicated batch 1 of synthetic PE guide RNA (pegRNA)/nicking guide RNAs (ngRNAs). A subset of the data shown in Figure 2E is analyzed in this panel. N=3. (C) Bulk PE outcomes of RNP-based delivery on three mutations in hESCs with indicated batch 2 of synthetic pegRNA/ngRNAs. A subset of the data shown in Figure 2E is analyzed in this panel. (D) Comparison of bulk PE outcomes between two batches of purified proteins on HEK3 (CTT insertion) edits in hESCs. N=3. (E) Comparison of bulk PE outcomes between two doses of RNP on HEK3 (CTT insertion) edits in hESCs. N=3. (Error bars indicate the SD, N=number of biological replicates).
-
Figure 2—figure supplement 2—source data 1
Unedited Coomassie blue staining gel.
- https://cdn.elifesciences.org/articles/79208/elife-79208-fig2-figsupp2-data1-v2.zip
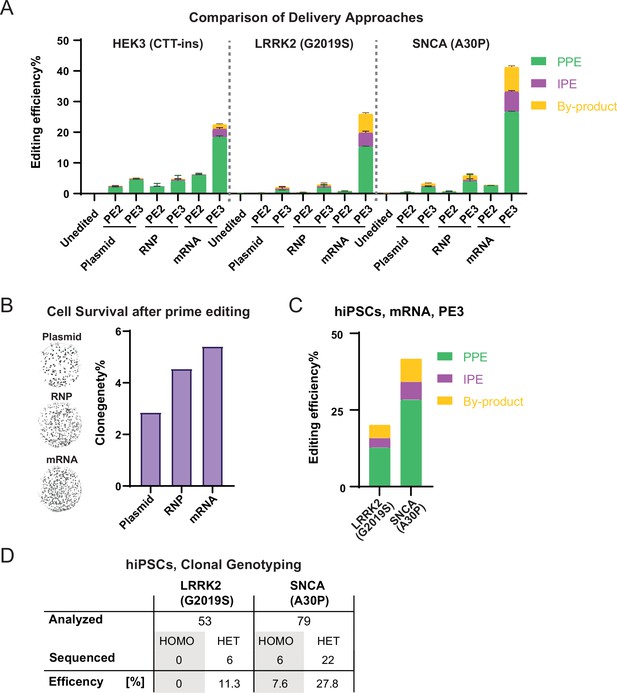
Highly efficient prime editing (PE) in human pluripotent stem cells (hPSCs) using mRNA-based delivery.
(A) Comparison of bulk PE outcomes between plasmid, RNP, and mRNA-based delivery on indicated modifications in hESCs. Plasmid, mRNA groups, N=3; RNP data shown in Figure 2E was included in this analysis for direct comparison, N=6. (B) Representative images and quantification of alkaline phosphatase staining comparing clonogenicity of hESCs after nucleofection between plasmid, RNP, and mRNA-based delivery. N=2. (C) Bulk PE outcomes on leucine rich repeat kinase 2 (LRRK2; G2019S) and α-Synuclein (SNCA; A30P) edits in a human-induced pluripotent stem cells (hiPSCs) line using mRNA-based delivery. N=2. (D) A summary of clonal genotyping from LRRK2 (G2019S) and SNCA (A30P) PE in hiPSCs indicating the number and editing efficiency of single cell-derived clones carrying the correct heterozygous (HET) or homozygous (HOMO) substitution. (N=number of biological replicates, Error bars indicate the SD for samples N>2).
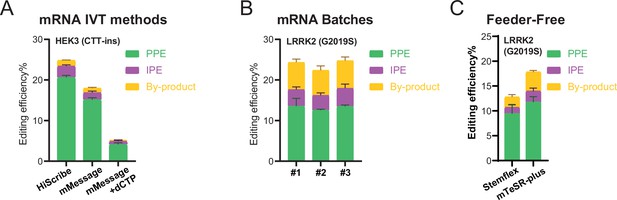
Quality control, parameter testing, and prime editing (PE) of feeder-free human embryonic stem cell (hESC) cultures with mRNA-based delivery.
(A) Comparison of bulk PE outcomes between three mRNA in vitro transcription methods on HEK3 (CTT insertion) edits in hESCs. N=3. (B) Comparison of bulk PE outcomes between three batches of in vitro transcribed mRNA on HEK3 (CTT insertion) edits in hESCs (HiScribe mRNA in vitro transcription). N=3. (C) Bulk PE outcomes on leucine rich repeat kinase 2 (LRRK2; G2019S) in hESCs cultured in feeder-free conditions. N=3. (Error bars indicate the SD, N=number of biological replicates).
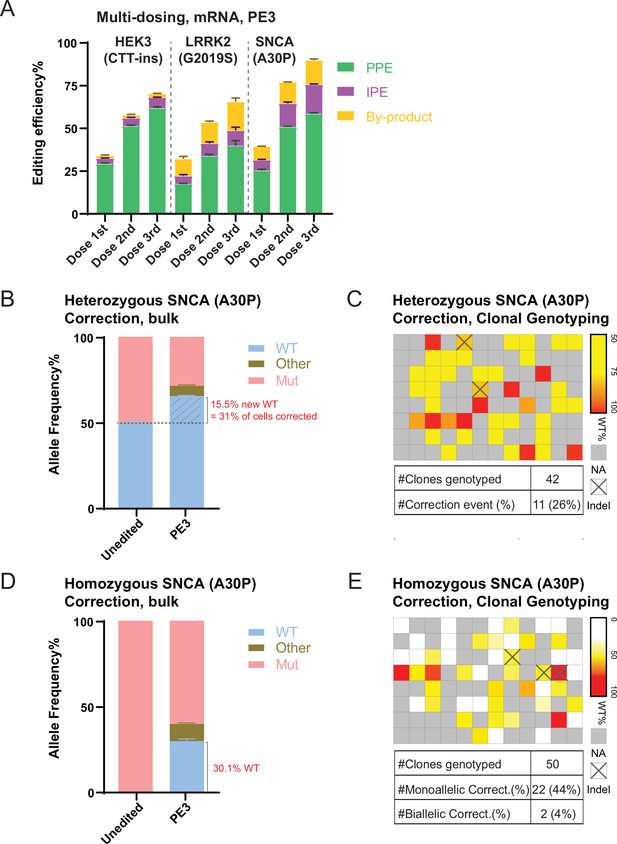
Repeated prime editing (PE) and reversion of an α-Synuclein (SNCA; A30P) mutation in human embryonic stem cells (hESCs).
(A) Comparison of bulk PE outcomes in a multi-dosing strategy using mRNA-based delivery on the three indicated mutations in hESCs. N=3. (B) Bulk next generation sequencing (NGS) analysis indicating allele spectrum before (unedited) and after mRNA-based PE (PE3, single dosing) to correct the heterozygous SNCA (A30P) mutation in hESCs. N=3. The dashed line indicates the 50% allele frequency. The portion of the wild-type (WT) allele converted from the mutant allele is hatched. (C) Heatmap and summary of clonal genotyping from an SNCA (A30P) heterozygous mutation correction experiment in a 96-well format. The wells with edited clones containing more than 5% insertion or deletion (indel) reads were labeled as Indel. NA indicates wells without hESCs. (D) Bulk NGS analysis indicating allele spectrum before (unedited) and after mRNA-based PE (PE3, single dosing) to correct the homozygous SNCA (A30P) mutation in hESCs. N=3. (E) Heatmap and summary of clonal genotyping from an SNCA (A30P) homozygous mutation correction experiment in a 96-well format. The wells with edited clones containing more than 5% indel reads were labeled as Indel. NA indicates wells without hESCs. (Error bars indicate the SD, N=number of biological replicates).
Additional files
-
Supplementary file 1
Table providing DNA Oligonucleotides and gBlocks gene fragment sequences.
- https://cdn.elifesciences.org/articles/79208/elife-79208-supp1-v2.docx
-
Supplementary file 2
Table providing list of generated plasmids and synthetic gRNA/ngRNA/pegRNA sequences.
- https://cdn.elifesciences.org/articles/79208/elife-79208-supp2-v2.docx
-
MDAR checklist
- https://cdn.elifesciences.org/articles/79208/elife-79208-mdarchecklist1-v2.pdf