Machine learning-assisted fluoroscopy of bladder function in awake mice
Figures
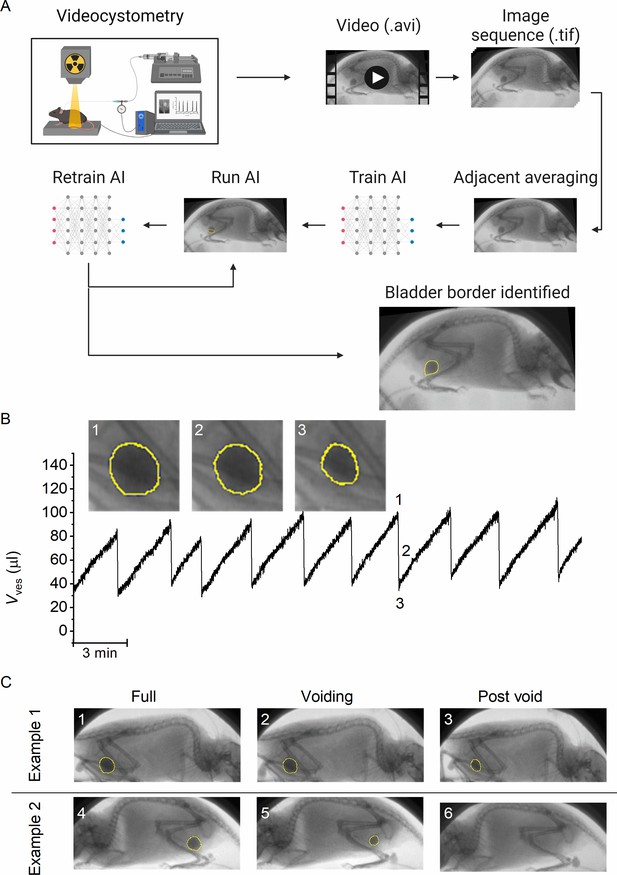
A machine-learning protocol for the automated annotation of the bladder in fluoroscopic images.
(A) Image analysis protocol using artificial intelligence-based automatic annotation of the bladder border. (B) Representative bladder volume trace from an anesthetized animal (1.2 g/kg urethane) based on artificial assisted automated annotation of the bladder. Annotated bladder images (blue line), corresponding to different filling states of the bladder, are shown. (C) Examples showing annotated bladders before, during, and after voiding in different positions. Example 1 represents the same animal as in panel B, whereas Example 2 represents an awake animal.
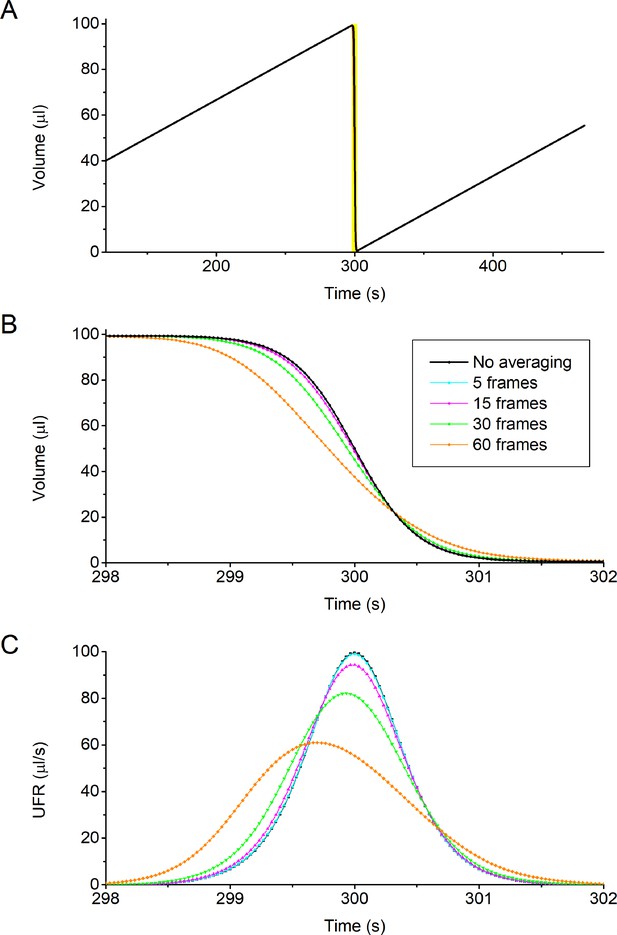
Effect of rolling averaging on voiding parameters.
(A, B) Simulated time course of a bladder, filled at 20 μl/min, that fully voids at time point 300 s (black trace; no averaging). Panel B zooms in on the 4 s surrounding the void, showing individual time points in a 30 Hz recording, similar to the fluoroscopy imaging used in this study. Also shown in are the time courses of bladder volume that would be calculated when using a rolling average with the indicated number of frames (N). These time courses were determined based on the assumption that, when averaging N images, the derived length of the long and short radii of the detected bladder circumference of the averaged image are equal to the mean value of the long and short radii of the N images. (C) Simulated time courses of the urethral flow rate (UFR) during a void, determined as minus the time derivative of the volume traces in (A, B). A rolling average of 15 frames, as used in this work, leads to a small (<5%) decrease in maximal UFR, with little effect on the time course of the void. As expected, further increasing the number of images for averaging (30 or 60 frames) leads to a gradual broadening of the void, associated with a shift and decrease of the voiding peak. Note that this simulation was done for a void with a short duration (t20–80 = 1.2 s), comparable to the results obtained in catheterized mice. The effect a 15-image rolling average will be even smaller for the fluoroscopic volumetry experiments in noncatheterized animals, where void durations were significantly longer (t20–80 = 2–5 s).
Video showing fluoroscopic imaging of a void in an awake mouse.
Bladder circumference detected by artificial intelligence-assisted automated annotation procedure is overlaid.
Video showing fluoroscopic imaging of a void in a urethane anesthetized mouse.
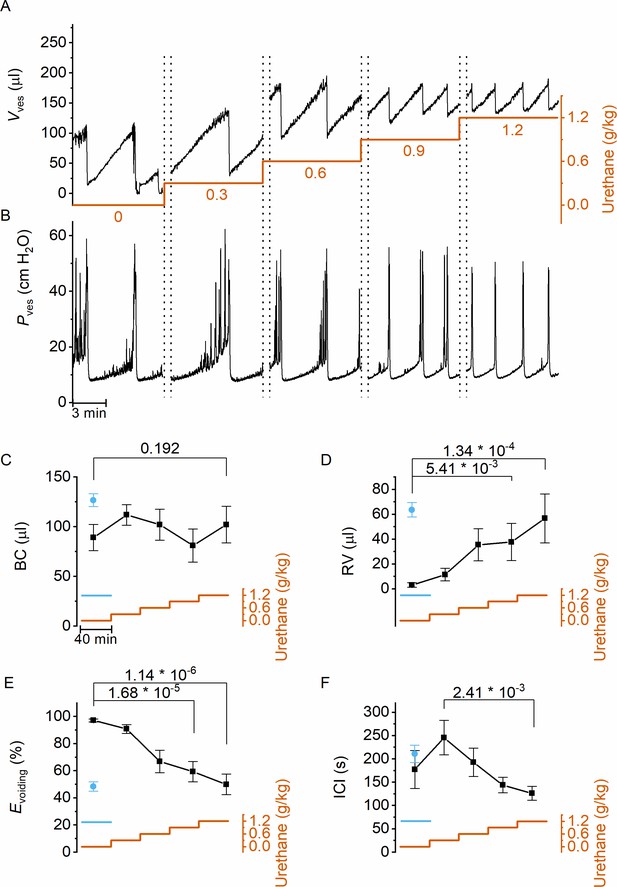
Dose-dependent effects of urethane on bladder volume and voiding efficiency.
(A, B) Short excerpts of simultaneous bladder volume and intravesical pressure recordings traces at increasing cumulative doses of urethane in catheterized mice. (C–F) Average bladder capacity, residual volume, voiding efficiency, and intercontractile interval at increasing doses of urethane (mean ± standard error of the mean [SEM]). Animals were assayed for 40 min at each dose, after which the dose as increased as indicated by the orange trace. For comparison, data from mice that immediately received a single dose of 1.2 g/kg at the start of the recording (Franken et al., 2021) are also included, shown by the blue symbols and blue line. One-way repeated measures analysis of variance (ANOVA) was used to test for statistically significant differences with the 0.0 g/kg condition.
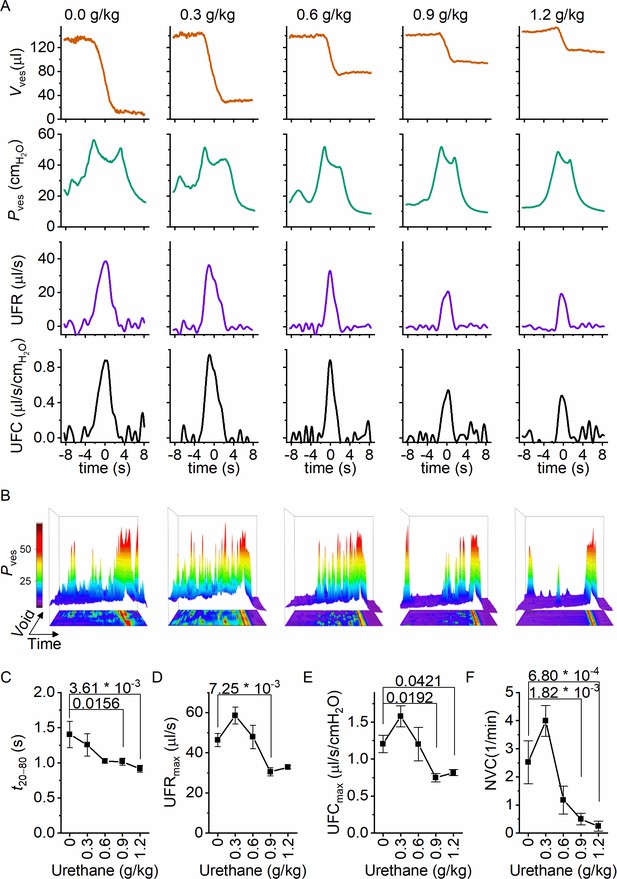
Dose-dependent effects of urethane on the voiding process.
(A) Zoomed-in examples of average bladder volume, bladder pressure, urethral flow rate, and urethral flow conductance at increasing doses of urethane in catheterized mice. (B) Combined 2D/3D pressure plots showing 100 s of intravesical pressure changes aligned to a voiding contraction at time point 80 s from a single animal at the indicated dose. Each row represents the pressure during one individual void. The color scale on the right represents the intravesical pressure (in cmH2O). (C–F) Assessment of the effect of urethane on void duration, urethral flow rate, urethral flow conductance, and nonvoiding contractions (mean ± standard error of the mean [SEM]). One-way repeated measures analysis of variance (ANOVA) was used to test for statistically significant differences with the 0.0 g/kg condition.
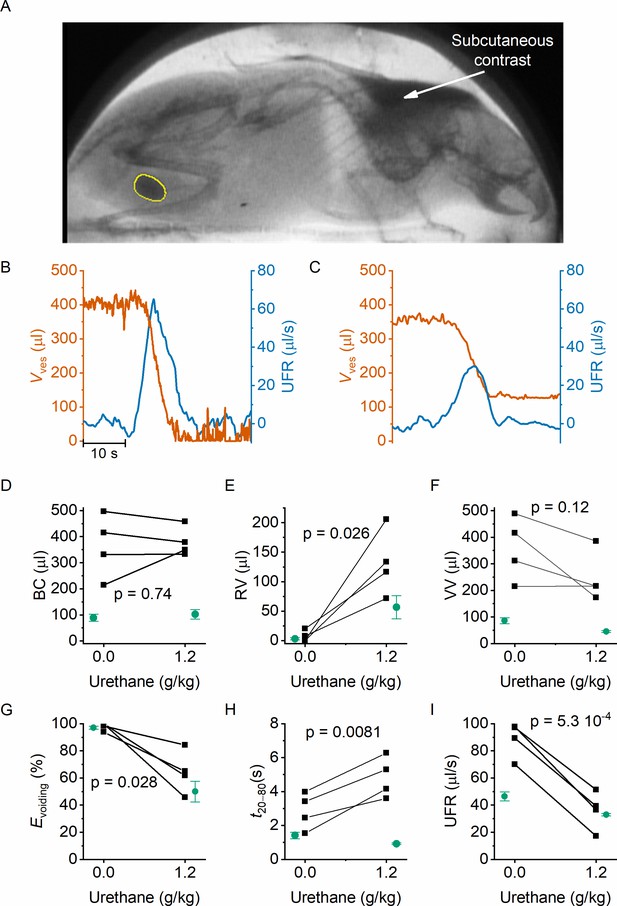
Noninvasive fluoroscopic volumetry.
(A) Fluoroscopic image showing the presence of a contrast agent in the scruff (arrow) and in the bladder of an awake and nonrestrained mouse, as well as the automatic annotation of the bladder border. (B–C) Zoomed-in example of bladder volume and urethral flow rate in an animal that did not receive any urethane anesthesia and in the same animal after dosing at 1.2 g/kg. Note that, due to the complete bladder emptying and slow filling in the absence of urethane, the void is followed by a period of low-accuracy volume measurement. (D–I) Comparison of the indicated voiding parameters in animals without an implanted catheter (black), before and after urethane dosing. In green, we show the corresponding values from catheterized animals reproduced from Figures 2 and 3. Paired t-tests comparing the values in the noncatheterized animals before and after urethane dosing yielded the indicated p values.
Tables
Reagent type (species) or resource | Designation | Source or reference | Identifiers | Additional information |
---|---|---|---|---|
Strain, strain background (Mus musculus) | Wild-type mice | Janvier | C57BL/6J | 12- to 16-week-old females |
Chemical compound, drug | Isoflurane | Dechra veterinary products | Iso-vet | Anesthesia |
Chemical compound, drug | Carprofen | Zoetis | Rimadyl | Analgesia |
Chemical compound, drug | Surgical skin glue | Vygon | ||
Chemical compound, drug | Iomeprol | Bracco Imaging Europe | Iomeron 250 | Contrast agent |
Chemical compound, drug | Iodixanol | GE Healthcare | Visipaque 320 mg I/ml | Contrast agent |
Chemical compound, drug | Urethane | Sigma-Aldrich | Anesthesia | |
Chemical compound, drug | Furosemide | Sanofi | Lasix | Diuretic |
Software, algorithm | ImageJ/FIJI | NIH | RRID:SCR_002865 | Image processing |
Software, algorithm | Igor Pro | Wavemetrics | RRID:SCR_000325 | Data processing |
Software, algorithm | Origin 9.0 | Originlab | RRID:SCR_014212 | Data processing |
Software, algorithm | NIS-Elements; NIS.ai | Nikon | RRID:SCR_014329 | Image processing |