Alternative splicing of apoptosis genes promotes human T cell survival
Figures
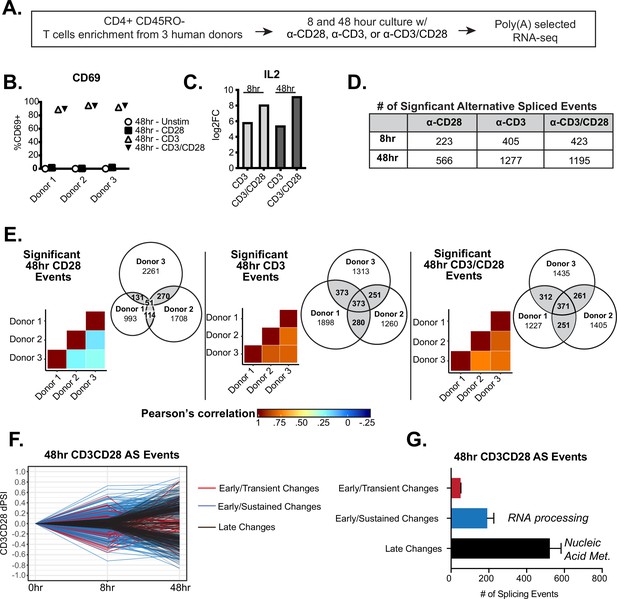
Temporal regulation of alternative splicing in primary human CD4+ T cells controls a distinct set of genes than are regulated by expression.
(A) Schematic of experimental set-up for RNA-sequencing (RNA-seq). (B) Flow cytometry analysis of CD69 cell surface expression on stimulated primary CD4+ CD45RO- T cells cultured for 48 hr. (C) Log2 fold changes (stimulated/unstimulated) of IL2 expression quantified by RNA-seq analysis. (D) Number of significant (dPSI >10%, p<0.05) splicing changes induced by either anti-CD28, anti-CD3, or anti-CD3CD28 antibodies for 8 or 48 hr of culture. dPSI is equivalent to stimulated PSI – unstimulated PSI for the corresponding timepoint (see Materials and methods). (E) Pearson’s correlation analysis of significant splicing events in the specified stimulation conditions compared between human donors. Venn diagrams display the overlap of significant splicing events induced by the specified stimulation condition compared between human donors. (F) Splicing events significantly (dPSI >10%, p<0.05) by the 48 hr-CD3CD28 condition were analyzed for differential temporal changes between 8 and 48 hr of culture. Events labeled as ‘Early/Transient Changes’ have a significant change at 8 hr but a decrease in dPSI of >2 by 48 hr, while ‘Early/Sustained Changes’ were significant at 8 hr and showed no decrease of more than twofold by 48 hr. Lastly, remaining events were labeled as ‘Late Changes’. (G) Averages of the number of significant events categorized based on their temporal patterns as seen in F, between human donors. Bar graph depicits mean +/-SEM for the 3 donors. Summary of top gene ontology (GO) categories presented by splicing events within ‘Early/Sustained Changes’ and ‘Late Changes’.
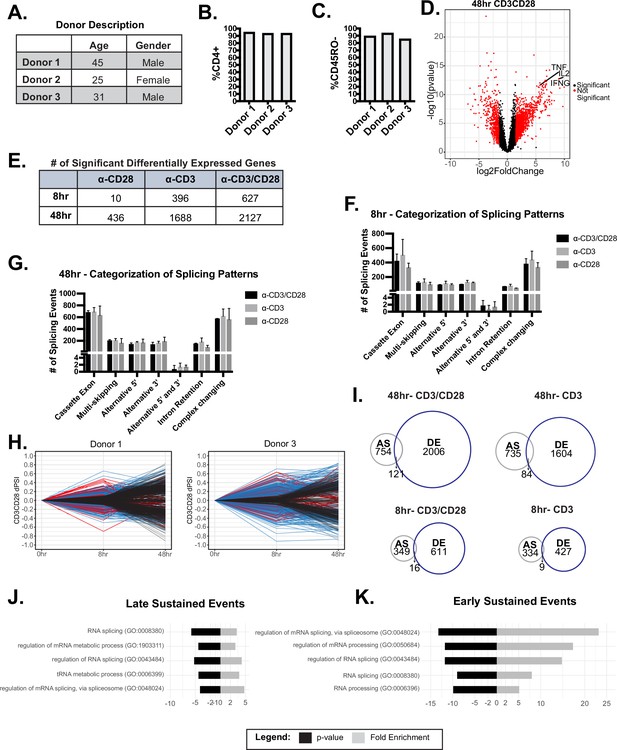
Additional information on initial RNA-sequencing (RNA-seq) analysis.
(A) Description donor characteristics used for RNA-seq analysis. (B–C) Flow cytometry analysis of the percentage of CD4 and CD45RO expression on the surface of T cells enriched in each donor. (D) Volcano plot of log2FC induced with CD3/CD28 stimulation at 48 hr of culture. (E) The number of significantly (log2FC >1.5, p<0.05) differentially expressed genes in the specified conditions, quantified by RNA-seq analysis. (F–G) Significant alternative splicing changes (dPSI >10%, p<0.05) induced by anti-CD28, anti-CD3, anti-CD3CD28 were quantified by RNA-seq analysis and categorized by common splicing patterns exhibited. The number of splicing events per splicing pattern was averaged between human donors. Bar graph depicits mean +/-SEM for the 3 donors. (H) Significant (dPSI >10%, p<0.05) 48 hr-CD3CD28 splicing changes were analyzed for differential temporal changes between 8 and 48 hr of culture. Events labeled as ‘Early/Transient Changes’, red, has a ratio <0.05 between 48 and 8 hr-CD3CD28. Subsequently, events were filtered for >10% PSI at 8 hr of CD3CD28 stimulation for ‘Early/Sustained Changes’, blue. Lastly, remaining events were labeled as ‘Late Changes’, black. (I) Overlap of significant alternative splicing events and significant differential expressed genes induced by the specified conditions (J–K) Gene ontology (GO) enrichment analysis of splicing events categorized as ‘Late/Sustained Changes’ or ‘Early/Sustained Changes’.
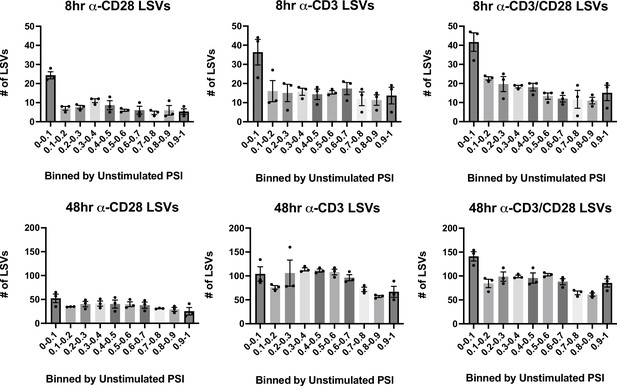
Change in splicing as binned by initial percent spliced isoform (PSI).
Significant splicing events in various stimulation conditions, described in Figure 1D, are binned by their unstimulated PSI levels in each stimulation condition. Each dot represents one human donor. Error bars represent SEM for the 3 donors.
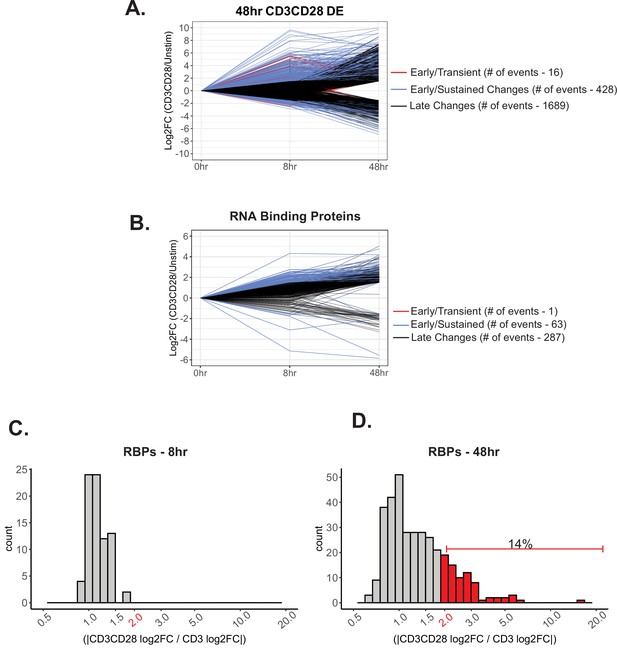
Differential gene expression induced by CD28 costimulation.
(A) Significantly differentially expressed genes (log2FC >1.5, p<0.05) induced upon CD3/CD28 stimulation for 48 hr were analyzed for differential temporal changes. Genes labeled as ‘Early/Transient Changes’, red, has a ratio <0.05 between 48 and 8 hr-CD3CD28. Subsequently, genes were filtered for >1.5 log2FC at 8 hr of CD3CD28 stimulation for ‘Early/Sustained Changes’, blue. Lastly, remaining genes were labeled as ‘Late Changes’, black. (B) RNA binding proteins that are significantly differentially expressed by induction of CD3/CD28 stimulation at 48 hr were analyzed for differential temporal changes between 8 and 48 hr of stimulation, as described above. (C–D) Histogram representing the number of significant differentially expressed genes regulated by CD28 costimulation for 8 and 48 hr of stimulation. Genes that are selected are significant (>1.5 log2FC, p<0.05) by CD3/CD28 stimulation at 48 hr. Genes that have a log2FC ratio (CD3CD28/CD3) greater than 2 are deemed regulated by CD28 costimulation.
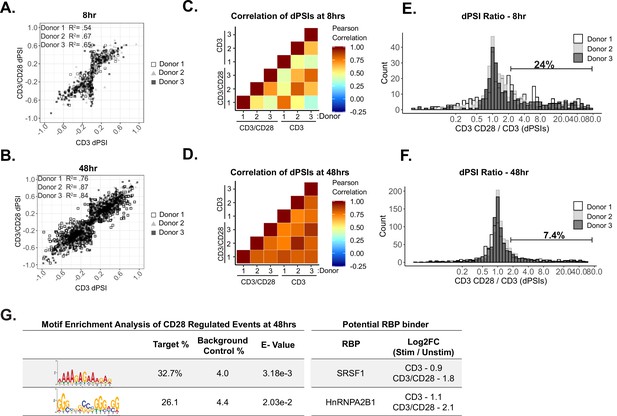
CD28 enhances a subset of alternative splicing events upon primary human CD4+ T cell activation.
(A–B) Dot plots displaying correlation trends of splicing changes induced by anti-CD3 and anti-CD3CD28 antibodies in stimulated human primary CD4+ CD45RO- T cells in their respective timepoints. (C–D) Pearson’s correlation analysis of splicing quantifications between induced by anti-CD3 and anti-CD3CD28 stimulation of human primary CD4+ T cells in their respective timepoints. (E–F) Histograms depict the ratio of anti-CD3CD28 percent spliced isoform (PSI) over anti-CD3 PSI to quantify the number of splicing changes enhanced by CD28 costimulation. Splicing events with a ratio >2 are defined as enhanced by CD28 costimulation. (G) (Left) Motif enrichment analysis of sequences that are enriched within splicing events regulated by CD28 costimulation at 48 hr of culture, as seen in F. (Right) Examples of RNA binding proteins that are regulated by CD28 costimulation based on RNA-seq expression analysis (Supplementary file 1) and have a consensus binding sequences similar to enriched sequence motifs.
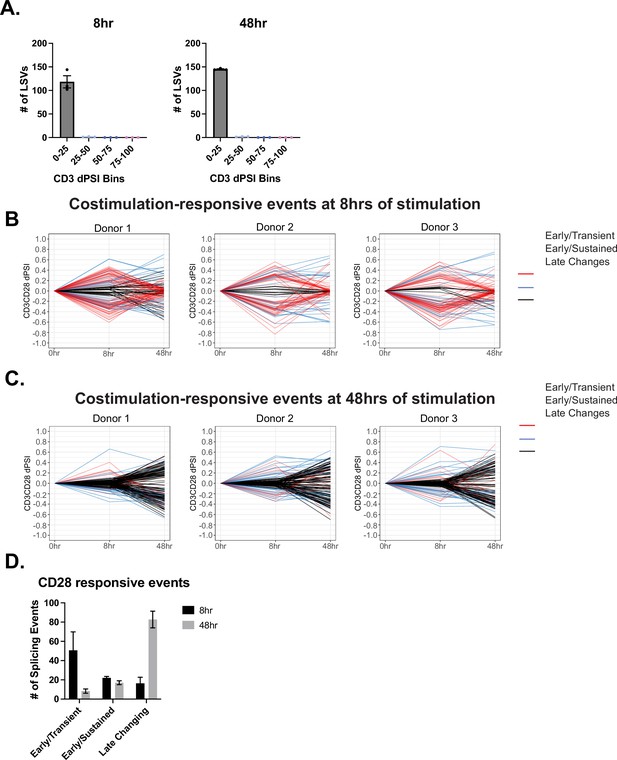
Further information on CD28 costimulation-induced events.
(A) Splicing events regulated by CD28 costimulation at 8 and 48 hr of stimulation, described in Figure 2E–F, are binned by their CD3 dPSI levels. Each dot represents one human donor. (B–C) Events regulated by CD28 costimulation are analyzed for differential temporal trends displayed between 8 and 48 hr of T cell stimulation. Events labeled as ‘Early/Transient Changes’ has a ratio <0.05 between 48 and 8 hr-CD3CD28. Subsequently, events were filtered for >10% PSI at 8 hr of CD3/CD28 stimulation. Lastly, remaining events were labeled as ‘Late Changes’. (D) Averages of the number of events regulated by CD28 costimulation that are categorized based on their differential temporal changes. Bar graphs depict mean ± SEM, n=3 biological replicates.
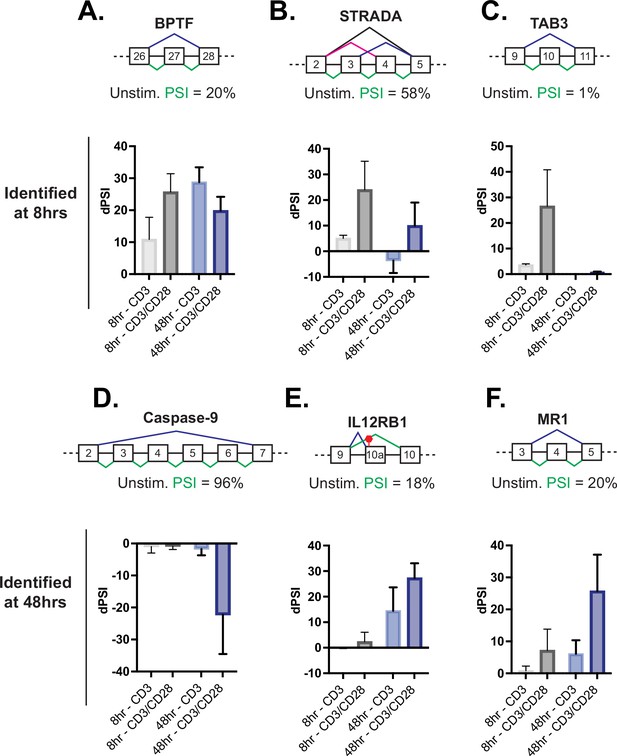
Splicing events enhanced by CD28 costimulation include those in genes involved in controlling apoptosis.
(A–C) Examples of genes regulated by CD28 costimulation at 8 hr of T cell stimulation, as quantified in Figure 2E. Each schematic displays the baseline percent spliced isoform (PSI) level from unstimulated cells and the splicing pattern quantified as PSI in green. The average deltaPSI (dPSI) levels in anti-CD3 and anti-CD3/CD28 stimulation conditions across three human donors, as determined by MAJIQ analysis of RNA-seq are in the graph below. Error bars represent SEM across the 3 donors. (D–F) Same as A-C, except showing examples of genes regulated by CD28 costimulation at 48 hr of T cell stimulation, as quantified in Figure 2F.
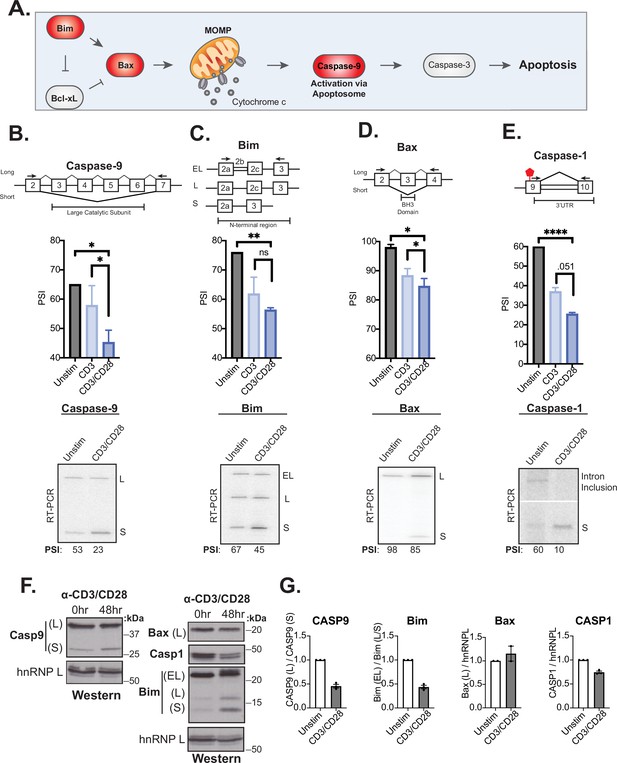
Splicing events enhanced by CD28 costimulation include those in genes involved in controlling apoptosis.
(A) Schematic of the intrinsic apoptosis signaling pathway highlights in red the role of Bim, Bax, and Caspase-9 in regulating apoptosis. (B–E) Primary human naïve (CD4+ CD45RO-) T cells from three human donors were stimulated for 48 hr with either anti-CD3 or anti-CD3CD28 antibodies. After RNA was harvested, low-cycle radioactive RT-PCR analysis of splicing changes were averaged, as shown in bar graphs (n=3). Arrows on splicing diagrams represent the proximal location of primers on the transcript which flanks sequences regulated by alternative splicing for each gene. A representative low-cycle RT-PCR gel from one donor displays splicing isoforms for each gene and associated quantified percent spliced isoform (PSI) values under each gel. Significance was assessed by Student’s t test and mean ± SEM values are plotted; p<0.05 (*), p<0.01(**), p<0.001(***). (F) Proteins were extracted from unstimulated and 48 hr-CD3CD28 stimulated primary human CD4+ T cells from one representative donor and blotted for caspase-9, Bax, caspase-1, Bim protein levels. hnRNPL is used as a loading control. (G) Bar graph quantifying average protein isoform levels with mean ± SEM values plotted. Each dot represents one human donor.
-
Figure 4—source data 1
Full gels for Figure 4B RT-PCR analysis of CASP9 splicing.
- https://cdn.elifesciences.org/articles/80953/elife-80953-fig4-data1-v2.zip
-
Figure 4—source data 2
Full gels for Figure 4C RT-PCR analysis of Bim splicing.
- https://cdn.elifesciences.org/articles/80953/elife-80953-fig4-data2-v2.zip
-
Figure 4—source data 3
Full gels for Figure 4D RT-PCR analysis of Bax splicing.
- https://cdn.elifesciences.org/articles/80953/elife-80953-fig4-data3-v2.zip
-
Figure 4—source data 4
Full gels for Figure 4E RT-PCR analysis of CASP1 splicing.
- https://cdn.elifesciences.org/articles/80953/elife-80953-fig4-data4-v2.zip
-
Figure 4—source data 5
Full gels for Figure 4F Protein expression analysis.
- https://cdn.elifesciences.org/articles/80953/elife-80953-fig4-data5-v2.zip
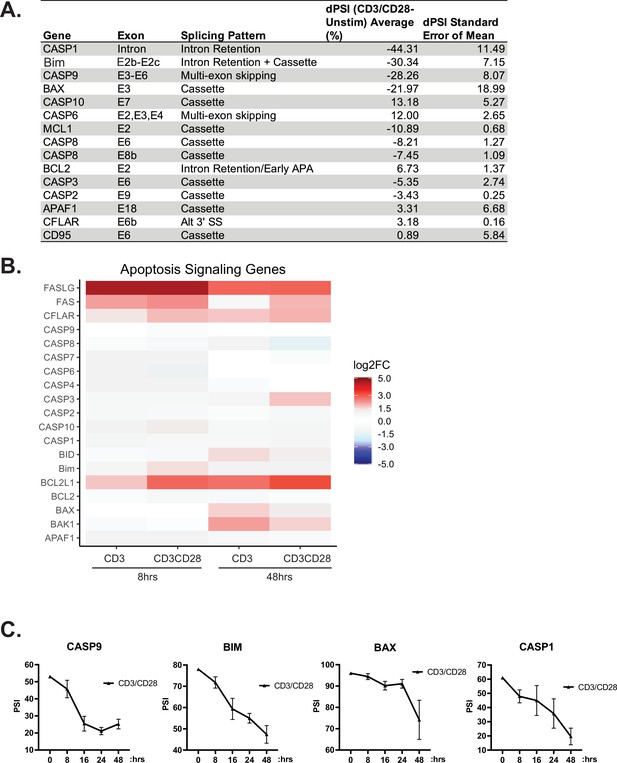
Further analysis of alternative splicing (AS) of apoptotic-related genes.
(A) RT-PCR analysis of AS changes within death signaling genes induced by anti-CD3/CD28 stimulation for 48 hr. (B) Differential expression analysis, quantified by RNA-seq, of death signaling. (C) Time course of splicing changes within primary human CD4+ T cells induced by anti-CD3/CD28 antibodies. Splicing changes were quantified by low-cycle radioactive RT-PCR analysis between at least three human donors. Line graphs depict mean ± SEM.
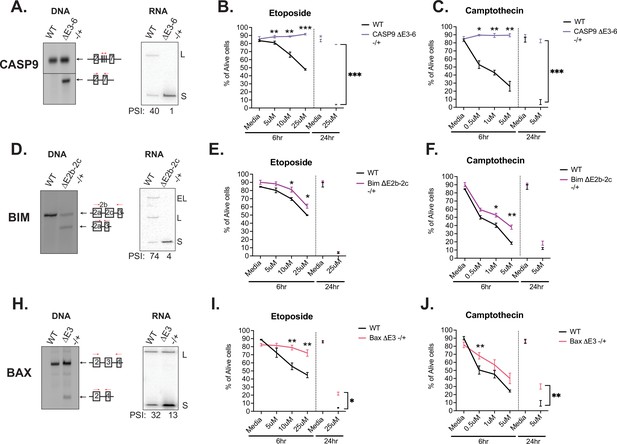
Activation-induced alternative splicing of apoptotic genes promotes T cell survival.
(A) Casp9ΔE3-6-/+ is a Jurkat derived monoclonal cell line that expresses decreased levels of the longer isoform of caspase-9 by heterozygous CRISPR/Cas9 targeting and deletion of exons 3–6. Editing was confirmed at the DNA level by genomic PCR amplification. At the RNA level, low-cycle RT-PCR confirms caspase-9 isoform expression changes. Casp9ΔE3-6-/+ cells were treated with either etoposide (B) or camptothecin (C) to induce apoptosis at the specified concentrations and timepoints. The percentage of alive cells were quantified through flow cytometry analysis by Annexin V and PI staining. Values differing from wildtype control across three independent experiments are noted. Significance was measured by Student’s t test with mean ± SEM values depicted; p<0.05 (*), p<0.01(**), p<0.001(***). (D–J) The validation and measurement of survival rates of BimΔE2b-2c-/+ and BaxΔE3-/+ cell lines are similar as described in A-C. Data from independent heterozygous clones is shown in Figure 5—figure supplement 1.
-
Figure 5—source data 1
Full gels for Figure 5A PCR and RT-PCR analysis of CASP9 CRISPR cell line.
- https://cdn.elifesciences.org/articles/80953/elife-80953-fig5-data1-v2.zip
-
Figure 5—source data 2
Full gels for Figure 5D PCR and RT-PCR analysis of Bim CRISPR cell line.
- https://cdn.elifesciences.org/articles/80953/elife-80953-fig5-data2-v2.zip
-
Figure 5—source data 3
Full gels for Figure 5H PCR and RT-PCR analysis of Bax CRISPR cell line.
- https://cdn.elifesciences.org/articles/80953/elife-80953-fig5-data3-v2.zip
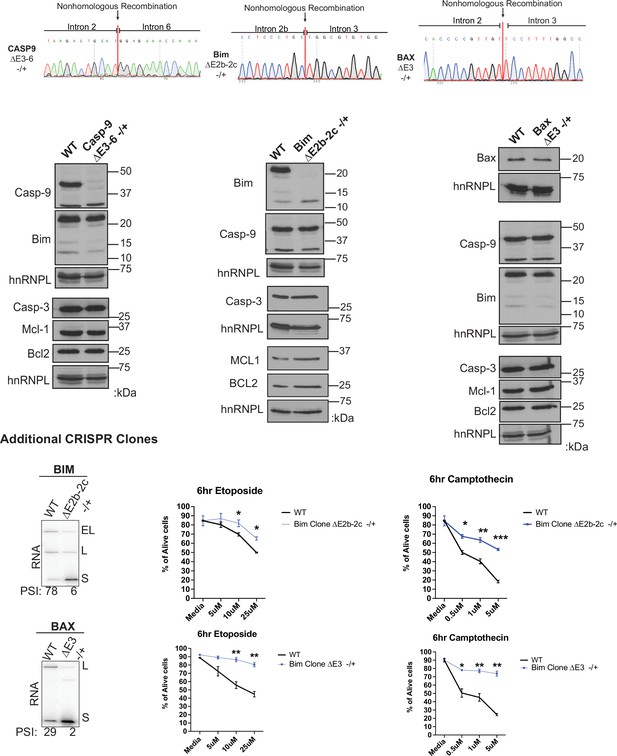
Further data validating CRISPR clones.
Validation assays of Jurkat cell lines using the CRISPR/Cas9 targeting system to delete genomic coordinates that encode for regulated alternative exons within caspase-9, Bim, and Bax genes. (Top) Sanger sequencing of genomic DNA isolated from specified CRISPR cell lines. Sanger sequencing peaks represent the deletion of the alternative exons within cell clones. Sequence of unmodified allele for each of the cell lines is shown in Figure 5—figure supplement 2. (Middle) Western blots demonstrate the protein expression changes of CRIPSR/Cas9 targeted genes, in addition to proteins that are not targeted by CRISPR/Cas9 that display consistent expression levels compared to wildtype Jurkat cells. (Bottom) Additional BimΔE2b-2c-/+ and BaxΔE3-/+ cell lines with RT-PCR validation analysis and Etoposide and Camptothecin-induced cell death analysis as described in Figure 5.
-
Figure 5—figure supplement 1—source data 1
Full gels for Figure 5—figure supplement 1 protein expression analysis of CRISPR cell lines.
- https://cdn.elifesciences.org/articles/80953/elife-80953-fig5-figsupp1-data1-v2.zip
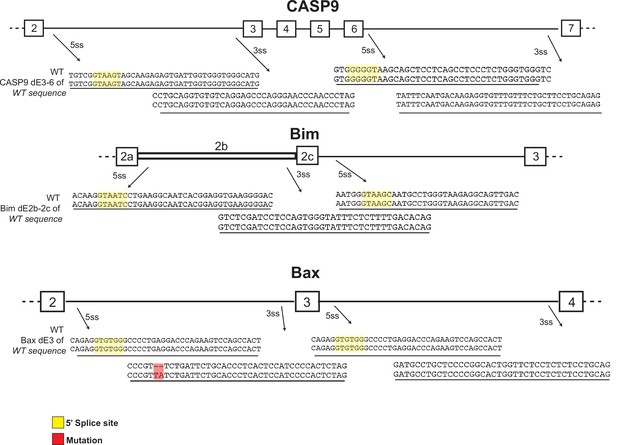
Sequence of wildtype allele in CRISPR heterozygous clones.
Sequences around splice sites of caspase-9, Bim, and Bax from Ref-Seq compared to that obtained from the wildtype allele in the heterozygous clones used in the main manuscript.
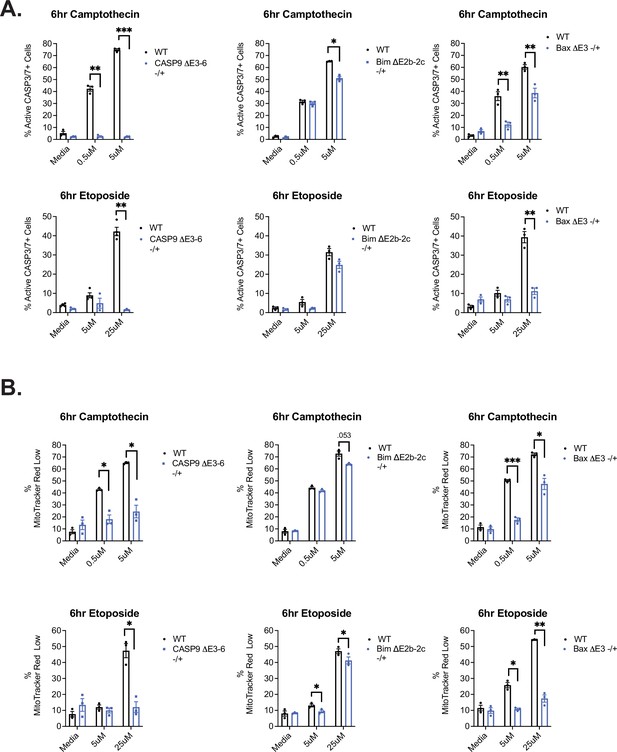
Assessment of additional apoptotic markers in CRISPR clones.
(A) CRISPR cell clones were incubated with camptothecin or etoposide for apoptosis induction for 6 hr and subsequently harvested to quantify the percentage of cells with active caspase-3/7 activity by utilizing caspase-3/7 FAM-FLICA probes. (B) CRISPR cell clones were incubated with camptothecin or etoposide for apoptosis induction for 6 hr and subsequently harvested to measure mitochondrial membrane permeabilization events via MitoTracker Red CMXRos staining by flow cytometry. Significance was measured by Student’s t test with mean ± SEM values reported; p<0.05 (*), p<0.01(**), p<0.001(***). n=3, independent experiments.
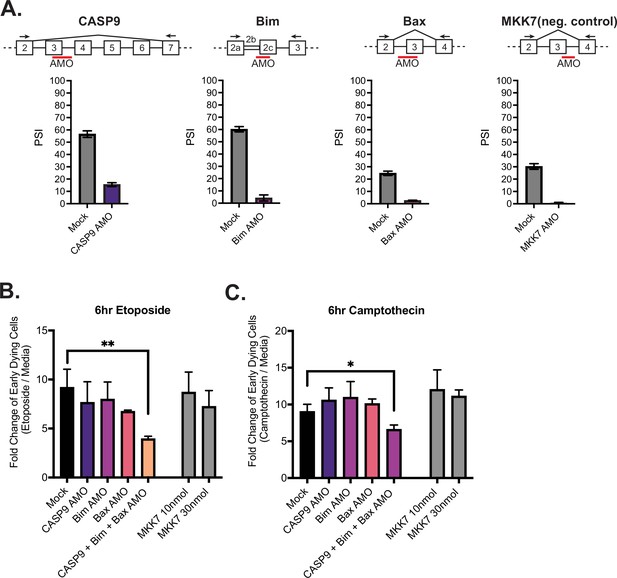
Activation-induced alternative splicing of apoptotic genes promotes T cell survival.
(A) Jurkats were individually transfected with antisense morpholino oligos (AMOs) that targeted splicing events within caspase-9, Bim, Bax, and MKK7 (negative control) genes, and rested for 48 hr, then subjected to low-cycle RT-PCR analysis to quantify splicing changes within each gene. Splicing diagrams represent relative placement of AMO along transcripts of each gene. n=3 independent experiments. As shown previously (Goehe et al., 2010), blocking of caspase-9 exon 3 is sufficient to cause skipping of the whole exon 3–6 cassette. (B) Forty-eight hr after AMO transfection, cells were incubated with either etoposide or camptothecin for 6 hr and cell survival was quantified through staining of Annexin V and PI by flow cytometry. Early dying cells are characterized as Annexin V+ and PI- cells. Significance was measured by Student’s t test across n=3 independent experiments with mean ± SEM values reported; p<0.05 (*), p<0.01(**). No differences between mock and single AMO treatments are statistically significant.
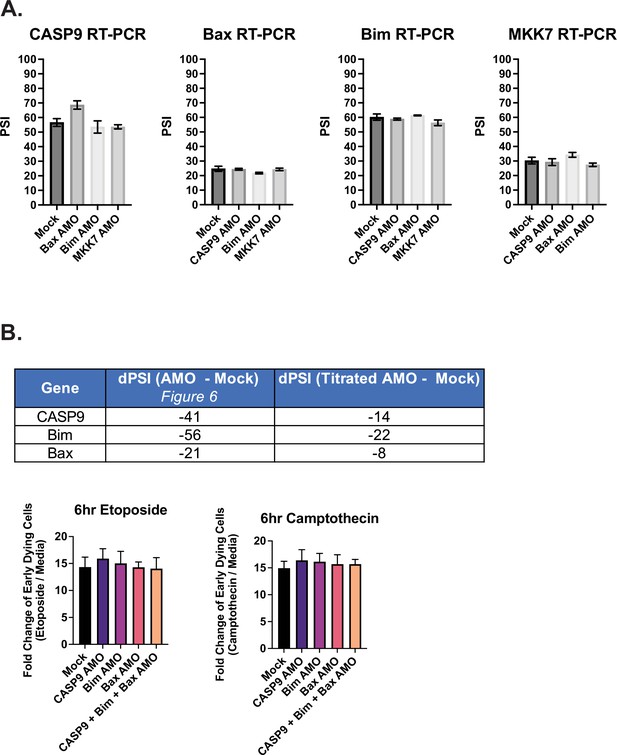
Additional analysis of antisense morpholino oligo (AMO)-treated Jurkat cells.
(A) As described in Figure 6, Jurkat cells were transfected with AMOs to induce splicing changes for subsequent apoptosis analysis. Here, low-cycle radioactive RT-PCR analysis quantified the rate of off-target effects of AMOs to influence splicing changes of non-targeted genes. (B) Jurkat cells were transfected with a titrated amount AMOs against CASP9, Bim, and Bax splicing events. The splicing analysis was quantified by low-cycle RT-PCR analysis and measured by dPSI (Titrated AMO PSI - Mock PSI) as seen in the table (Top). (Bottom) Forty-eight hr after AMO transfection, cells were incubated with either etoposide or camptothecin for 6 hr and cell survival was quantified through staining of Annexin V and PI by flow cytometry. Early dying cells are characterized as Annexin V+ and PI- cells.
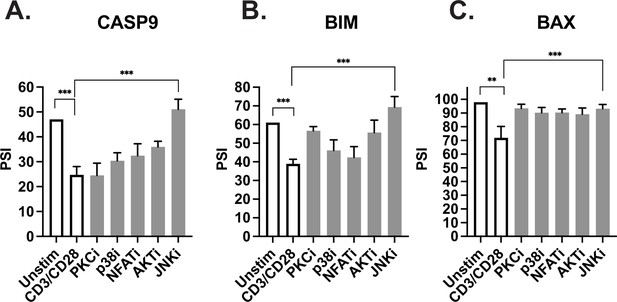
JNK signaling coordinates regulation of caspase-9, Bim, and Bax.
(A–C) Primary CD4+ CD45RO- T cells isolated from three human donors were individually incubated with inhibitors for 1 hr and then stimulated with anti-CD3CD28 antibodies for 48 hr. The effect of each signal transduction target was averaged between at least two different inhibitors (see Materials and methods). Bar graphs represent low-cycle radioactive RT-PCR analysis of splicing changes within casapse-9, Bim, and Bax genes, as described in Figure 4. Significance was measured by Student’s t test with mean ± SEM values reported; p<0.05 (*), p<0.01(**), p<0.001(***). n=3, independent experiments.
Tables
Reagent type (species) or resource | Designation | Source or reference | Identifiers | Additional information |
---|---|---|---|---|
Gene (Homo sapiens) | CASP9 | GenBank | #842 | |
Gene (Homo sapiens) | BCL2L11 | GenBank | #10018 | |
Gene (Homo sapiens) | BAX | GenBank | #581 | |
Gene (Homo sapiens) | CASP1 | GenBank | #834 | |
Cell line (Homo sapiens) | Wildetype Jurkat T cells | Lynch and Weiss, 2000 | JSL1 | Clonal population of Jurkat T cells |
Cell line (Homo sapiens) | Casp9ΔE3-6 (-/+) | This paper | Cell line generated by CRISPR/CAS9 system | |
Cell line (Homo sapiens) | BimΔE2b-2c (-/+) | This paper | Cell line generated by CRISPR/CAS9 system | |
Cell line (Homo sapiens) | and BaxΔE3 (-/+) | This paper | Cell line generated by CRISPR/CAS9 system | |
Transfected construct (Homo sapiens) | pSpCas9(BB)–2A-GFP (PX458) | Addgene | Plasmid #48138 | Construct to transfect cells with Crispr guide RNAs |
Biological sample (Homo sapiens) | Primary Human CD4+ T cells | Human Immunology core at the University of Pennsylvania | CD4+ T cells enriched from apheresis product | |
Antibody | Anti-human CD69-PE (Mouse monoclonal) | Biolegend | Cat # 310905 | 1:100 |
Antibody | Anti-human CD28 L293 (Mouse monoclonal) | BD | Cat # 348040 | 2.5 µg/mL of soluble antibody |
Antibody | Anti-human CD3 NA/LE (Mouse monoclonal) | BD | Cat # 555336 | 2.5 µg/mL of plate bound antibody |
Antibody | Anti-human Caspase-9 (Rabbit polyclonal) | Cell Signaling | Cat # 9502 | 1:1000 |
Antibody | Anti-human Bim (Rabbit monoclonal) | Abcam | Cat # ab32158 | 1:1000 |
Antibody | Anti-human Bax (Rabbit monoclonal) | Abcam | Cat # ab325034 | 1:1000 |
Antibody | Anti-human Caspase-1 (Rabbit monoclonal) | Abcam | Cat # ab179515 | 1:1000 |
Peptide, recombinant protein | Annexin V- Pacific Blue | Biolegend | Cat # 640917 | |
Peptide, recombinant protein | IL-2 | Miltenyi | Cat # 130-097-742 | |
Commercial assay or kit | RNA STAT-60 | Tel-Test Inc | ||
Commercial assay or kit | Anti-human CD45RO human microbeads | Biolegend | Cat # 130-046-001 | |
Commercial assay or kit | Caspase-3/7 FAM-FLICA assay | Immunochemistry Technologies | Cat # 93 | |
Chemical compound, drug | Camptothecin | Millipore Sigma | Cat # 208925 | |
Chemical compound, drug | Etoposide | Sigma-Aldrich | Cat # E1383 | |
Chemical compound, drug | Propidium Iodide | Thermo Fisher | Cat # c34557 | |
Chemical compound, drug | MitoTracker Red CMXRos | Thermo Fisher | Cat # M7512 | |
Chemical compound, drug | R0-31-8220 | Selleckchem | S7207 | |
Chemical compound, drug | Go6983 | Selleckchem | S2911 | |
Chemical compound, drug | SB20350 | Selleckchem | S1076 | |
Chemical compound, drug | Skepinone-L | Selleckchem | S7214 | |
Chemical compound, drug | Cyclosporin A | Selleckchem | S2286 | |
Chemical compound, drug | FK506 | Selleckchem | S5003 | |
Chemical compound, drug | MK-2206 | Selleckchem | S1078 | |
Chemical compound, drug | SP600215 | Selleckchem | S1460 | |
Chemical compound, drug | JNK-IN-8 | Selleckchem | S4901 | |
Chemical compound, drug | Tanzisertib | Selleckchem | S8490 | |
Software, algorithm | PANTHER Classification System | PANTHER Classification System | Version 17.0 | |
Software, algorithm | STREME algorithm | MEME suite software | Version 5.4.1 | |
Software, algorithm | Flowjo | Flowjo | Version 10.7.1 | |
Software, algorithm | GraphPad Prism | GraphPad Software | Version 9.4.0 | |
Software, algorithm | RStudio | The R foundation | Version 1.1.463 |
Additional files
-
MDAR checklist
- https://cdn.elifesciences.org/articles/80953/elife-80953-mdarchecklist1-v2.pdf
-
Supplementary file 1
Differential gene expression changes upon CD3 and/or CD28 stimulation.
- https://cdn.elifesciences.org/articles/80953/elife-80953-supp1-v2.xlsx
-
Supplementary file 2
Alternative splicing events which are changed significantly upon CD3 and/or CD28 stimulation.
- https://cdn.elifesciences.org/articles/80953/elife-80953-supp2-v2.xlsx
-
Supplementary file 3
Changes in alternative splicing over time following CD3 and CD28 stimulation.
- https://cdn.elifesciences.org/articles/80953/elife-80953-supp3-v2.xlsx
-
Supplementary file 4
Motifs enriched around regulated alternative splicing events.
- https://cdn.elifesciences.org/articles/80953/elife-80953-supp4-v2.xlsx