Environmental morphing enables informed dispersal of the dandelion diaspore
Figures
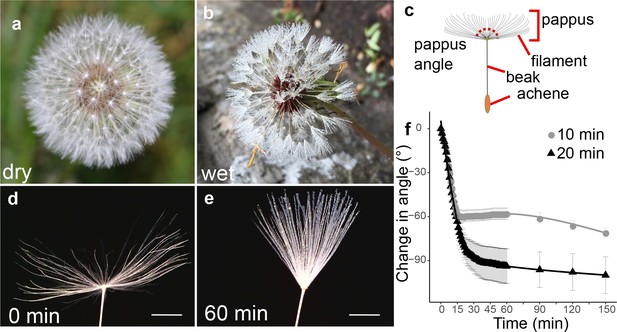
Moisture induces reversible closure of the dandelion pappus.
(a) Image of a dry dandelion infructescence, (b) image of a wet dandelion infructescence, (c) schematic of a dandelion diaspore indicating features, and (d) image of dry dandelion pappus (scale bar: 2 mm). (e) Image of dandelion pappus after 1 hr in moisture chamber with a 20 min humidifier treatment (scale bar: 2 mm). (f) Time course of change in pappus angle between outermost hairs with 10 or 20 min humidifier treatment, n = 10 and n = 12 samples for 10 and 20 min treatments, respectively; error bars are s.e.m.
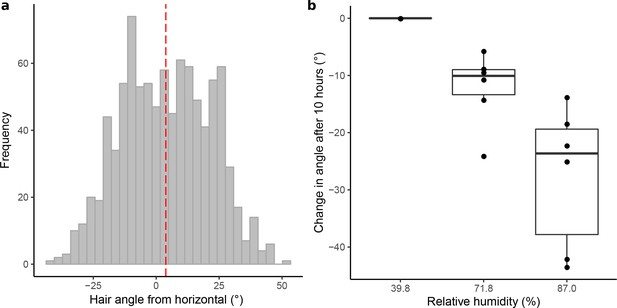
Angles of pappus hairs in dry and humid conditions.
(a) The angle of individual hairs of dandelion pappi. Angle is calculated relative to horizontal in which the dandelion diaspore beak (see Figure 1c) forms the vertical axis, n = 10 pappi, n = 932 hairs. Red dashed line indicates the median value. (b) High relative humidity partially closes the dandelion pappus. Change in pappus angle at stable relative humidity (after 10 hr in chamber with saturated salt solutions), n = 7 for 39.8% relative humidity and n = 6 for 71.8% and 87.0% treatments.
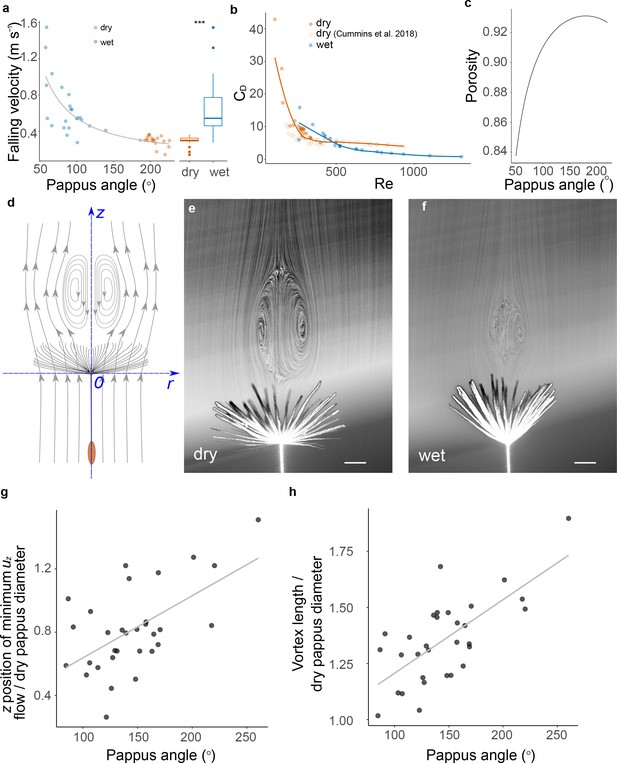
Pappus morphing alters diaspore flight and fluid dynamics.
(a) Falling speed at varying pappus angles, before and after wetting for 1 hr in moisture chamber, asterisks indicate statistically significant difference at p<0.0001, n = 20; (b) relationship between Reynolds number (Re) and drag coefficient (CD) for dry and wet samples, open red circles indicate data from weighted/clipped dry diaspores from Cummins et al., 2018, n = 10 diaspores, filled circles are same data as (a), n = 20 diaspores; (c) estimated porosity at varying pappus angles; (d) schematic illustrating axes and direction of flow; (e) flow visualisation of dandelion fruit with open pappus, contrast adjusted to illustrate vortex more clearly (scale bar: 2 mm); (f) flow visualisation of dandelion fruit with a partially closed pappus, contrast adjusted to illustrate vortex more clearly (scale bar: 2 mm); (g) distance of the region of minimum uz from the pappus centre at varying pappus angle, position is nondimensionalised for each sample by the diameter of the pappus when dry, n = 12 diaspores; and (h) longitudinal length of the separated vortex ring (SVR) non-dimensionalised by the diameter of the pappus when dry, at varying pappus angles, n = 12 diaspores.
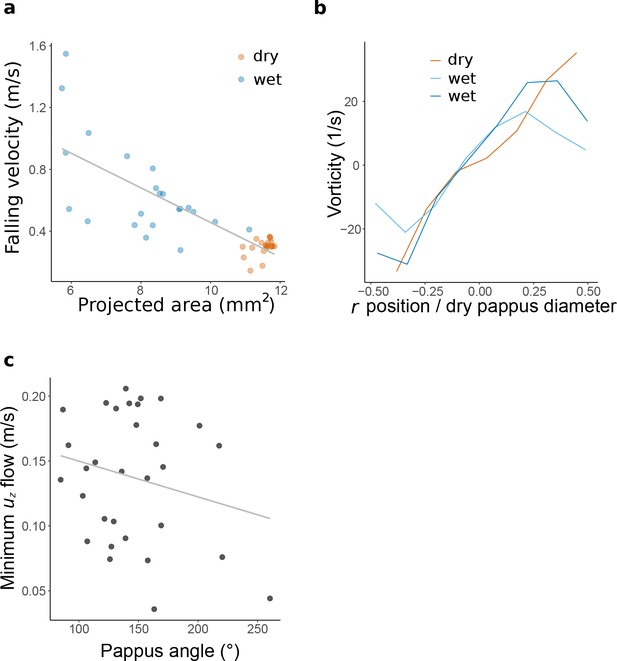
Changes to the diaspore falling velocity and separated vortex ring (SVR) as the pappus angle changes.
(a) Falling velocity reduces as projected area reduces. Projected area calculated using measured pappus angles and assuming hair lengths of 7.41 mm and diameters of 16 μm according to Cummins et al., 2018; (b) vorticity along the r axis of vortex images at calculated position of minimal uz flow. The r position is non-dimensionalised by dividing by the dry pappus diameter for each sample, with 0 indicating the centre of the pappus. Plot for a single sample at three different pappus angles, representative of n = 10 samples. (c) The minimum uz flow velocity in the separated vortex ring at varying pappus angles.
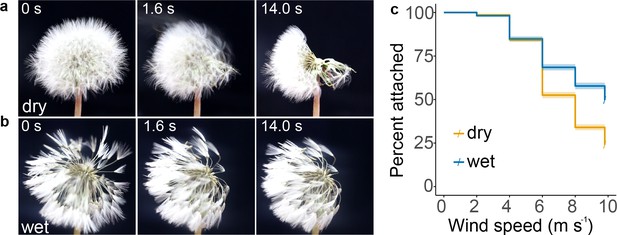
Detachment of diaspores from capitula.
(a) Snapshots of detachment dynamics at 8 m s–1 wind speed for a dry capitulum, air flow direction is from right to left of the images; (b) snapshots of detachment at 8 m s–1 wind speed for a wet capitulum; and (c) survival plot of percentage of fruits attached to capitula at varying wind speeds for 9.1% turbulent intensity flow in dry and wet conditions, tested 10–14 days after opening of capitula, n = 20 capitula per treatment corresponding to 3001 and 2654 diaspores for dry and wet conditions, respectively; shading around lines indicates s.e.m.
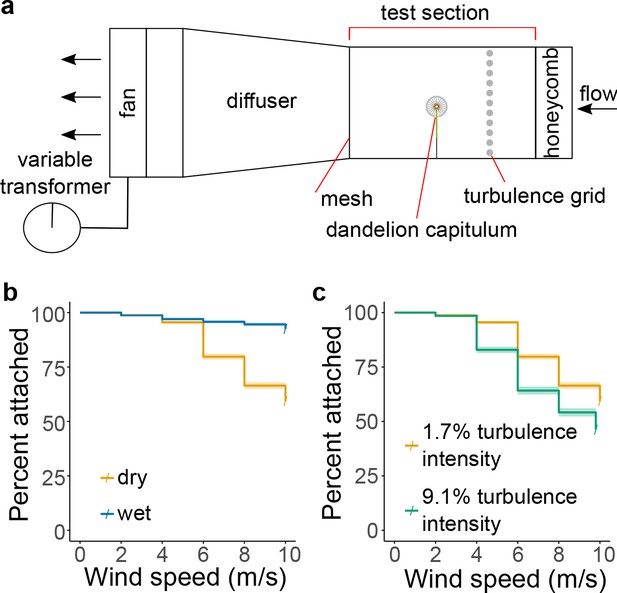
Detachment assay setup and detachment measurements for young capitula and at varying turbulence intensity.
(a) Schematic illustrating experimental setup for detachment assays; (b) survival plot of percentage of fruits attached to capitula at varying wind speeds for 1.7% turbulent intensity flow in dry and wet conditions, tested 3 days after opening of capitula, n = 21 capitula per treatment corresponding to 3736 and 3652 diaspores for dry and wet conditions, respectively, shading around lines indicates s.e.m. (c) Survival plot of percentage of fruits attached to capitula at varying wind speeds for dry conditions with turbulent intensities of 1.7% and 9.1%, tested 3 days after opening of capitula (data for 1.7% turbulent intensity are the same as for dry capitula in panel b), n = 20 capitula for the 9.1% turbulent intensity treatment corresponding to 2677 diaspores, shading around lines indicates s.e.m.
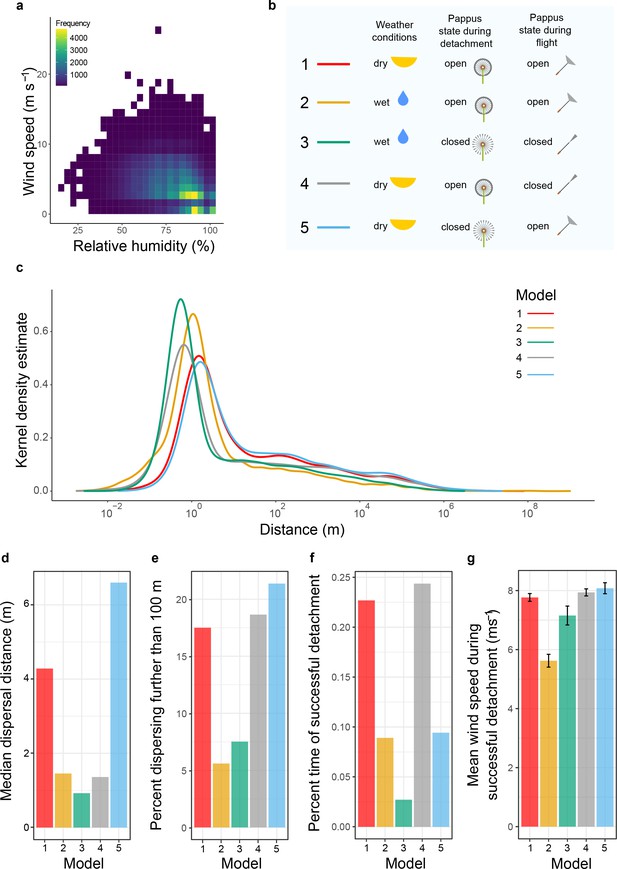
Change in pappus angle modifies dispersal according to meteorological conditions.
(a) Relationship between relative humidity and wind speed in Edinburgh, UK. (b) Model descriptions indicating whether dry (relative humidity < 90%), or wet (relative humidity ≥ 90%) weather conditions were used, whether the pappus was simulated to be open or closed during detachment, and whether the simulated diaspore falling velocity during flight was based on an open or closed pappus. (c) Modelled kernel density estimate of predicted dispersal distances for diaspores with open or closed pappi and for different weather conditions (see b for model details). (d) Median dispersal distance for each model. (e) Percent of 10,0000 dispersal simulation events that travelled further than 100 m. (f) Percent of time in which diaspores successfully detached after 172,800 simulations (representing the number of seconds in a 48 hr period). (g) Mean wind speed for time points (172,800 simulations) when detachment occurred.
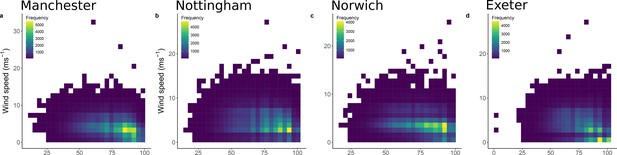
The relationship between relative humidity and wind speed in four additional locations.
(a) Manchester, (b) Nottingham, (c) Norwich, and (d) Exeter.
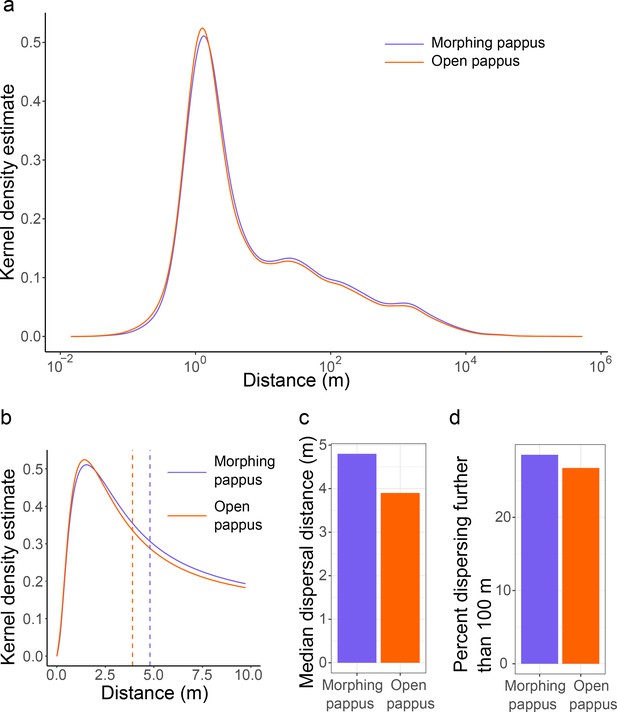
Modelled kernel density estimate of predicted dispersal distances for diaspores with a morphing or constantly open pappi in all weather conditions.
(a) Full dispersal kernel with distance plotted on a log10 scale. (b) Dispersal kernel portion from 0 to 10 m dispersal distance, dashed vertical lines indicate medians for the entire dispersal kernel. (c) Median dispersal distance. (d) Percent seeds dispersing further than 100 m.