Structural insights into actin isoforms
Figures
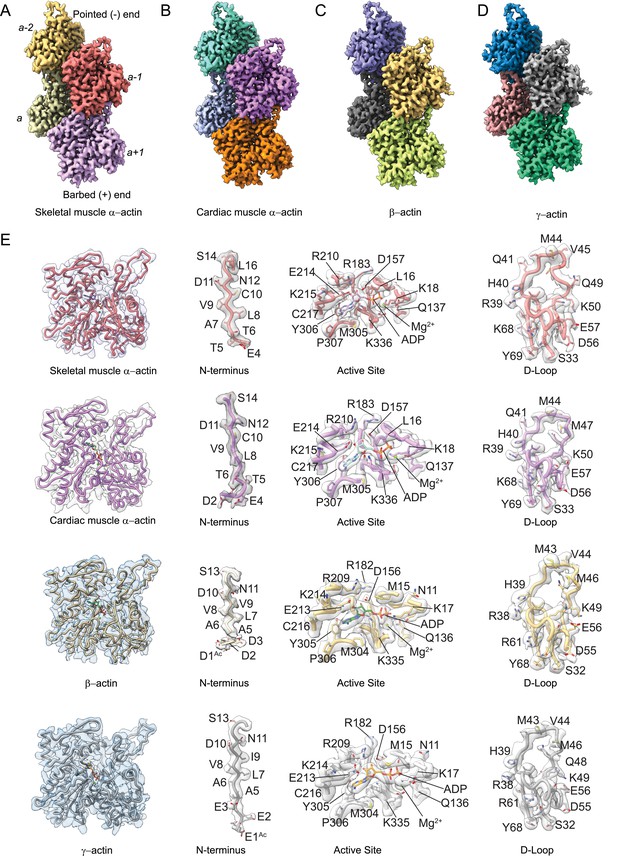
Cryo-electron microscopy (cryo-EM) filament structures of actin isoforms.
(A) Helical reconstruction of skeletal muscle α-actin, (B) cardiac muscle α-actin, (C) β-actin, and (D) γ-actin. Views in (B–D) are according to (A). Four individual actin protomers in the filament are shown and denoted with italic numbers. The pointed (−) and barbed (+) ends are indicated. (E) Representative key regions of actin isoforms with corresponding cryo-EM densities in transparent surface representation are shown. The protein backbone and amino acid side chains are shown in licorice and stick representation, respectively. Throughout this work, amino acids are numbered according to the sequence of mature actin isoforms (Figure 1—figure supplement 1C).
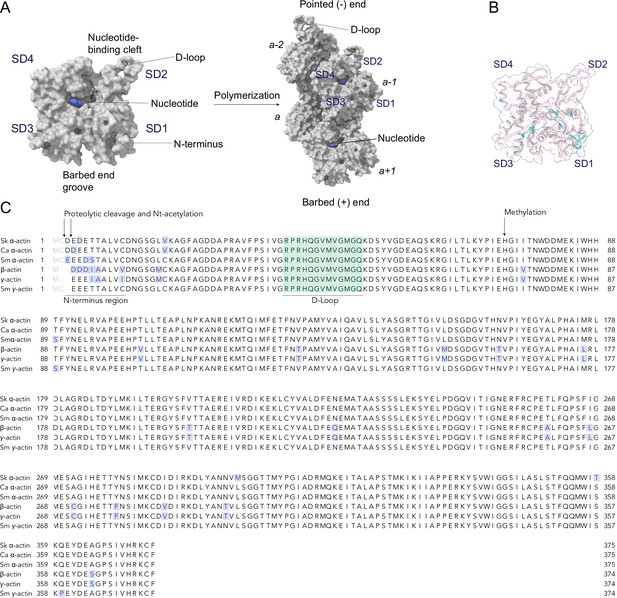
Sequence conservation in actin isoforms.
(A) The classical view of the monomer structure of actin. Key regions are indicated. Monomers self-assemble into helical, polarized filaments. The arrangement of individual actin protomers in a filament is shown, and the relative position is denoted with italic numbers. The pointed (−) and barbed (+) end, the nucleotide-binding cleft, and the barbed end groove are indicated. Monomer and filament structure not drawn to scale. (B) ConSurf analysis shows the conservation of amino acid positions in actin isoforms. Variations are shown in cyan color. The protein backbone is shown in licorice representation. (C) Sequence alignment of actin isoforms: rabbit skeletal muscle α-actin (Skα-actin; UniProt ID: P68135), bovine cardiac muscle α-actin (Caα-actin; UniProt ID: Q3ZC07), human smooth muscle α-actin (Smα-actin; UniProt ID: P62736), human β-actin (UniProt ID: P60709), human γ-actin (UniProt ID: P63261), and human smooth muscle γ-actin (Smγ-actin; UniProt ID: Q3ZC07). Conserved amino acids are shown in black, and variable amino acids are highlighted in blue. Amino acids that are absent in mature actin isoforms are shown in gray. Key structural regions and resolved post-translational modifications (PTMs) in our cryo-electron microscopy (cryo-EM) structures are indicated.
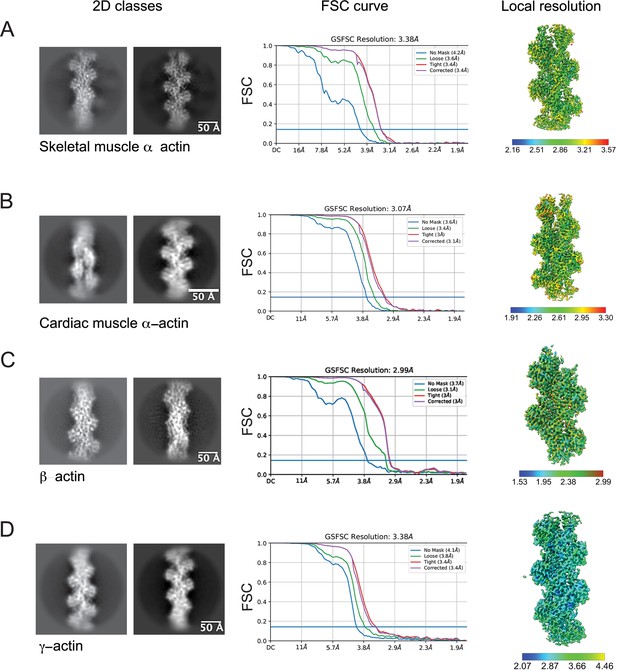
Image processing summary for actin isoforms.
(A–D) 2D classes, global map-model Fourier Shell Correlation (FSC) curves, and the local resolution estimated of the experimental maps are shown for skeletal muscle α-actin (A), cardiac muscle α-actin (B), β-actin (C), and γ-actin (D). The gold standard, FSC 0.143 criterion was used to estimate the global resolution of actin isoforms. The local resolution gradient is in Angstrom (Å).
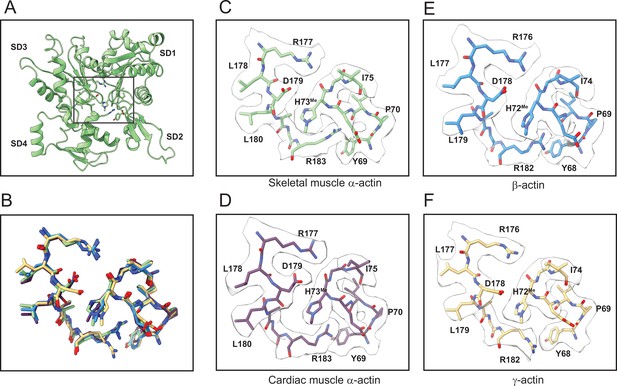
Methylation of H72/H73 in actin isoforms.
(A) The methylated histidine region in the actin protomer is indicated with a rectangle. (B) Superimposition of methylated histidine regions in actin isoform structures. Colors are according to panels C–F. (C) H73Me and neighboring residues in skeletal muscle α-actin. (D) H73Me and neighboring residues in cardiac muscle α-actin. (E) H72Me and neighboring residues in β-actin. (F) H72Me and neighboring residues inγ-actin. Cryo-electron microscopy (Cryo-EM) densities, contoured at ≥2σ, are shown in surface representation in C–F. Panel A is shown in cartoon representation, and panels B–F are shown in stick representation.
Structure of a skeletal muscle α-actin protomer with the corresponding cryo-electron microscopy (cryo-EM) density.
Structure of a skeletal muscle α-actin protomer in ribbon representation with the corresponding cryo-EM density shown as gray surface contoured at ≥2σ. Amino acid side chains are shown in stick representation. ADP and Mg2+ are shown in stick and sphere representation, respectively.
Structure of a cardiac muscle α-actin protomer with the corresponding cryo-electron microscopy (cryo-EM) density.
Structure of a cardiac muscle α-actin protomer in ribbon representation with the corresponding cryo-EM density shown as gray surface contoured at ≥2σ. Amino acid side chains are shown in stick representation. ADP and Mg2+ are shown in stick and sphere representation, respectively.
Structure of a β-actin protomer with the corresponding cryo-electron microscopy (cryo-EM) density.
Structure of a skeletal muscle α-actin protomer in ribbon representation with the corresponding cryo-EM density shown as gray surface contoured at ≥1.8σ. Amino acid side chains are shown in stick representation. ADP and Mg2+ are shown in stick and sphere representation, respectively.
Structure of a γ-actin protomer with the corresponding cryo-electron microscopy (cryo-EM) density.
Structure of a skeletal muscle α-actin protomer in ribbon representation with the corresponding cryo-EM density shown as gray surface contoured at ≥2σ. Amino acid side chains are shown in stick representation. ADP and Mg2+ are shown in stick and sphere representation, respectively.
Structures of the N-termini of actin isoforms with corresponding cryo-electron microscopy (cryo-EM) densities.
Structures of the N-termini of skeletal muscle α-actin, cardiac muscle α-actin, β-actin, and γ-actin. The corresponding cryo-EM densities are shown as surface and mesh representation contoured at ≥2σ. The protein backbone and side chains are shown in licorice and stick representation, respectively.
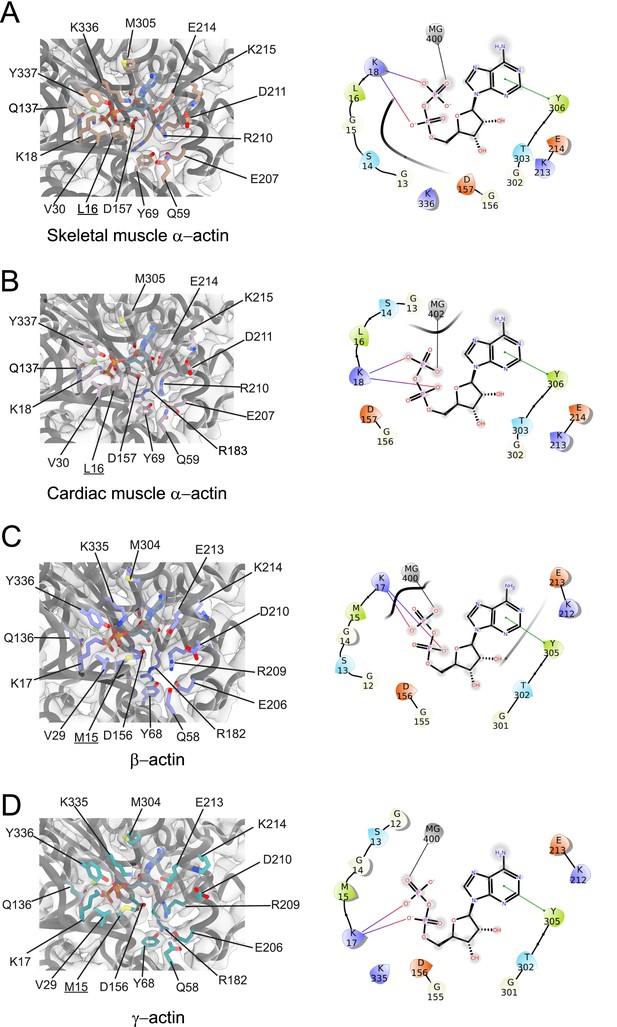
Conserved nucleotide-binding cleft active site in actin isoforms.
(A–D) Coordination of Mg2+·ADP in the nucleotide-binding cleft of skeletal muscle α-actin (A), cardiac muscle α-actin (B), β-actin (C), and γ-actin (D). Underlines indicate locations of amino acid substitutions between actin isoforms. The protein backbone and side chains are shown in licorice and stick representation, respectively. ADP is shown in cyan-colored stick representation. Electron densities for key amino acids in the nucleotide-binding cleft active site of actin isoforms are shown. Schematic representations of key interactions in the nucleotide-binding cleft active sites of the respective actin isoforms are shown in the right panel. The schematics are not drawn to scale.
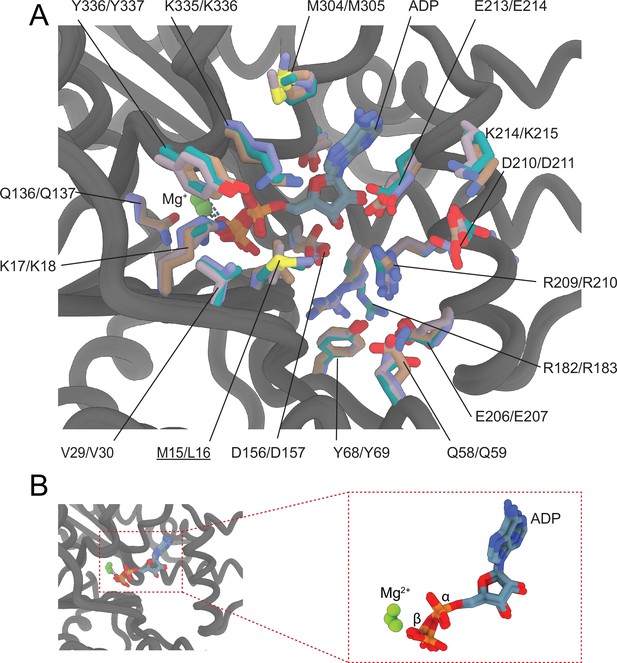
Coordination of Mg2+·ADP in the nucleotide-binding cleft of actin isoforms.
(A) Superimposition of the nucleotide-binding cleft active site of actin isoforms. Key amino acids are indicated. ADP is shown in cyan-colored stick representation, and Mg2+ is shown as green sphere. Underlines indicate locations of amino acid substitutions between actin isoforms. The protein backbone and side chains are shown in licorice and stick representation, respectively. (B) Comparison of the position of ADP and Mg2+ in the nucleotide-binding cleft active site of actin isoforms. Colors are according to (A).
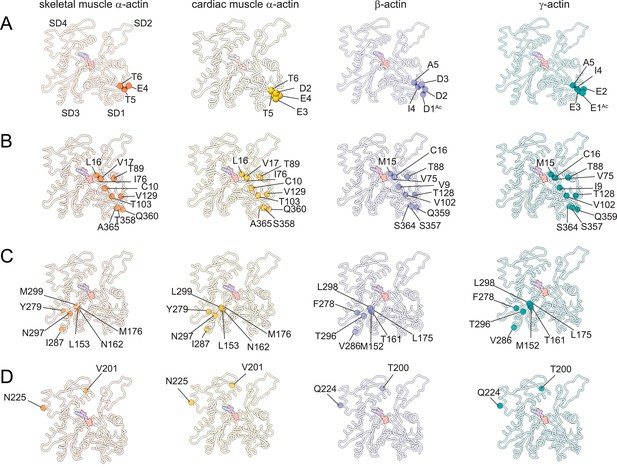
Similarities and differences between actin isoforms.
(A) Sequence variations at the N-terminus located in SD1 of actin isoforms. (B) Sequence variations in SD1 of actin isoforms. (C) Sequence variations in SD3 of actin isoforms. (D) Sequence variations in SD4 of actin isoforms. SD2 is conserved between actin isoforms. The identical and nonidentical amino acids at sites of substitutions within the actin protomer across isoforms are shown for skeletal muscle α-actin (orange), cardiac muscle α-actin (yellow), β-actin (purple), and γ-actin (teal) as spheres. Note that the first three amino acids of skeletal muscle α-actin and the first amino acid of cardiac muscle α-actin are unresolved in our structures. The protein backbone is shown in licorice representation, and the substituted amino acids are shown in spheres representation.
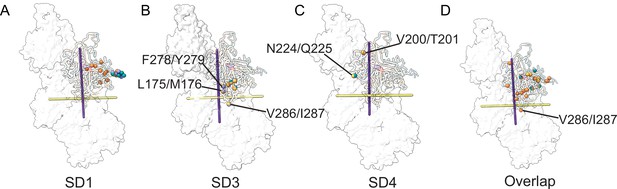
Amino acid variations along the longitudinal and transverse axis of actin isoforms.
(A–D) Variable amino acid distributions along the longitudinal and transverse axis of actin isoforms. Nonconserved amino acids are shown along with the longitudinal (purple axis) and transverse axis (yellow) for actin subdomains SD1 (A), SD3 (B), and SD4 (C). (D) Distribution of all variable amino acids in SD1, SD3, and SD4 for all actin isoforms. For clarity, only amino acids close to the transverse and longitudinal axis are labeled.
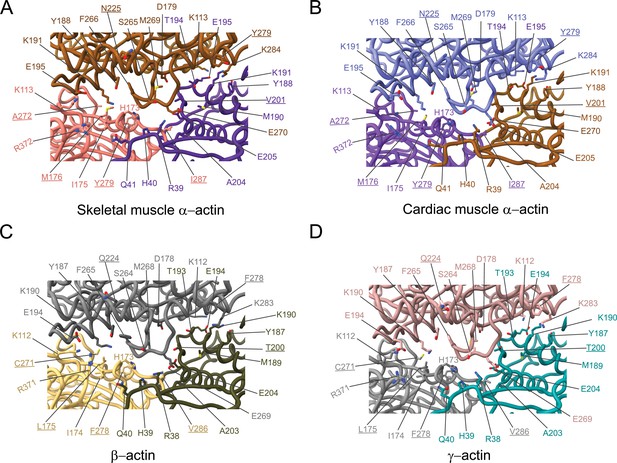
Comparative structural analysis of the longitudinal interprotomer interface.
(A–D) Key residues at the interprotomer interface of skeletal muscle α-actin (A), cardiac muscle α-actin (B), β-actin (C), and γ-actin (D). Individual protomers in actin isoforms are oriented according to Figure 4—figure supplement 1A. Underlines indicate locations of amino acid substitutions between actin isoforms. The protein backbone and side chains are shown in licorice and stick representation, respectively.
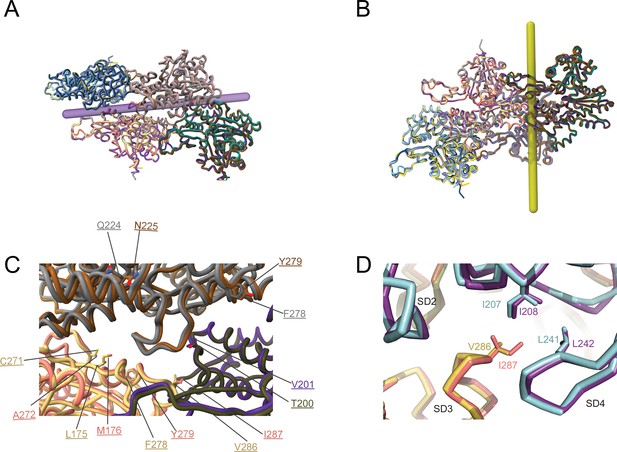
Transverse and longitudinal interprotomer interfaces in actin isoforms.
(A) Analyzed transverse interprotomer interface (yellow rod) in actin isoforms. (B) Analyzed longitudinal interprotomer interface (lilac rod) in actin isoforms. (C) Substituted amino acids in the longitudinal interprotomer interface of skeletal muscle α-actin and β-actin. Colors of the protomers are according to the Figure 4. (D) Substituted amino acid V286/I287 in the transverse interprotomer interface of skeletal muscle α-actin and β-actin. The protein backbone and amino acid side chains are shown in licorice and stick representation.
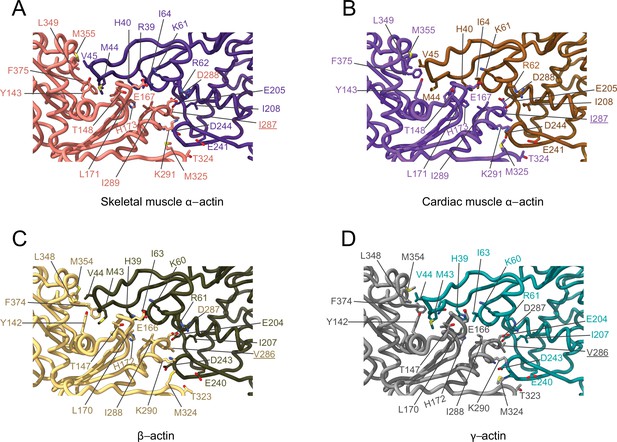
Comparative structural analysis of the transverse interprotomer interface.
(A–D) Key residues at the interprotomer interface of skeletal muscle α-actin (A), cardiac muscle α-actin (B), β-actin (C), and γ-actin (D). Individual protomers in actin isoforms are oriented according to Figure 4—figure supplement 1B. Underlines indicate locations of amino acid substitutions between actin isoforms. The protein backbone and amino acid side chains are shown in licorice and stick representation, respectively.
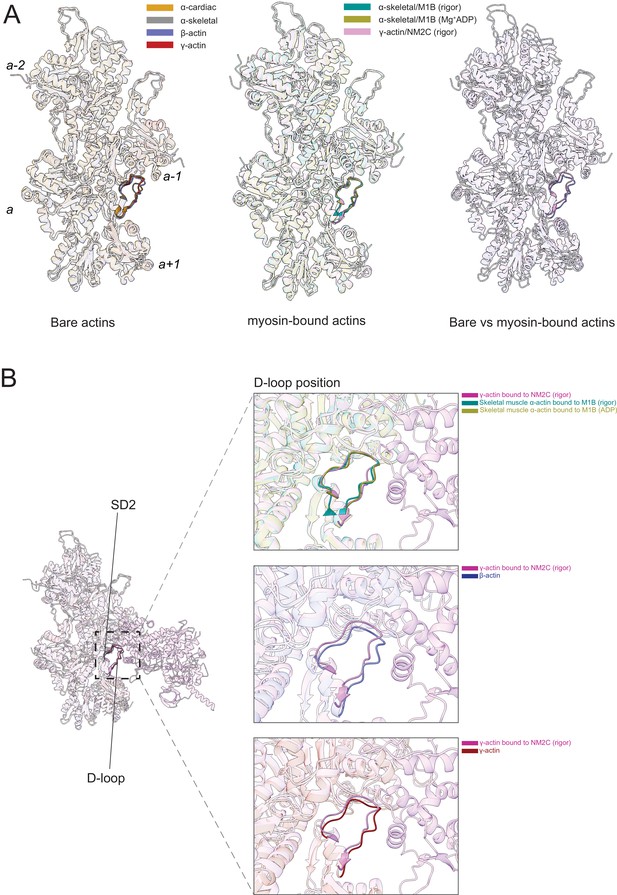
The actomyosin interface.
(A) Superimposition of bare actin isoform structures in the Mg2+·ADP state (left), superimposition of myosin-bound actin isoforms structures (middle), and overlap of bare versus myosin-bound actin structures (right) are shown. (B) Zoomed-in view of the actomyosin interface at the D-loop region. For clarity, only D-loops involved in the binding of myosins are highlighted in the respective dark colors. The offset between the structures in the lower two panels is caused by a conformational change of SD2 in myosin-bound compared to bare actin structures.
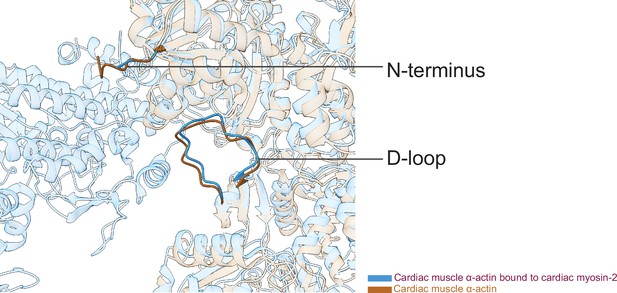
The actomyosin interface of the cardiac thin filament compared to bare cardiac muscle α-actin.
Superimposition of the structures of cardiac myosin-2 bound to the thin filament and bare cardiac muscle α-actin.
Conformational changes of the D-loop in bare and myosin-bound actin.
Superimposition of the structures of bare β-actin (blue) and bare γ-actin (red) with the structure of NM2C bound to γ-actin (pink). To show the extent of conformational changes between bare and myosin-bound actin structures, a morph (gray) is drawn between the structures of bare actin isoforms and the structure of NM2C bound to γ-actin. The D-loop of actin is highlighted in dark color. The NM2C motor domain is not shown.
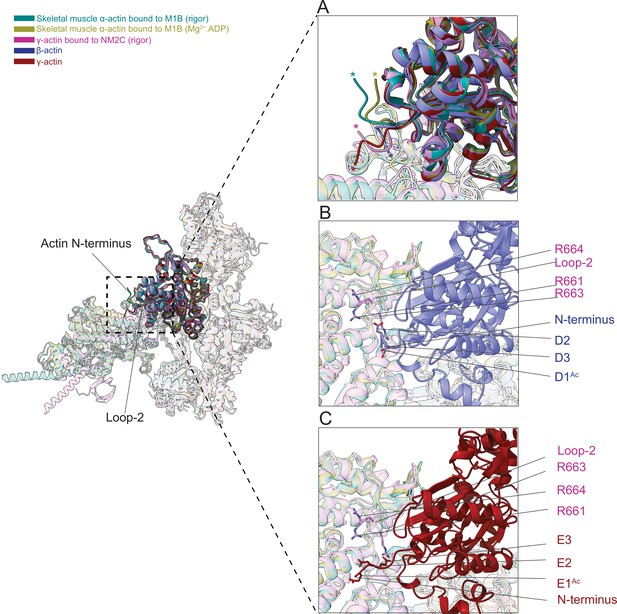
The N-terminus of actin interacts with loop-2 of myosins.
(A) Close-up view of the N-termini of bare β-actin (blue), bare γ-actin (red), myosin-bound actin isoforms M1B (green, lime), and NM2C (pink). The N-termini are indicated with an asterisk. (B) A cluster of positively charged amino acids in the loop-2 of myosins is in close proximity to amino acids D1Ac to D3 in the N-terminus of bare β-actin (blue) and, (C) a cluster of positively charged amino acids in the loop-2 of myosins is in close proximity to amino acids E1Ac to E3 in the N-terminus of bare γ-actin (red).
Conformational changes at the actomyosin interface.
Superimposition of the structures of bare β-actin (blue) and bare γ-actin (red) with the structure of NM2C bound to γ-actin (pink). To show the extent of conformational changes between bare and myosin-bound actin structures, a morph (gray) is drawn between the structures of bare actin isoforms and the structure of NM2C bound to γ-actin. The N-terminus and D-loop of actin are highlighted and shown with respect to the NM2C motor domain.
Tables
Data collection, image processing, and structure characteristics summary.
Map | Skeletal muscle α-actin | Cardiac muscle α-actin | β-actin | γ-actin |
---|---|---|---|---|
Data collection | ||||
Microscope | FEI Titan Krios G3i | FEI Titan Krios G3i | FEI Titan Krios G3i | FEI Titan Krios G3i |
Voltage (kV) | 300 | 300 | 300 | 300 |
Detector | Gatan K3 | Gatan K3 | Gatan K3 | Gatan K3 |
Automation software | EPU | EPU | EPU | EPU |
Energy filter slit width (eV) | 20 | 20 | 20 | 20 |
Recording mode | Super-resolution | Super-resolution | Super-resolution | Super-resolution |
Magnification (nominal) | 81,000 | 81,000 | 81,000 | 81,000 |
Movie micrograph pixel size (Å) | 0.891 | 0.891 | 0.891 | 0.891 |
Total Dose rate (e−/Å2) | 65 | 60 | 50 | 65 |
Defocus range (µm) | –0.5 to –2.5 | –0.5 to –2.5 | –0.5 to –2.5 | –0.5 to –2.5 |
Spherical aberration (mm) | 0.01 | 0.01 | 0.01 | 0.01 |
Movies | 2046 | 1444 | 1352 | 2952 |
Total extracted particles | 261,195 | 657,300 | 279,120 | 1,249,379 |
Total # of refined particles | 185,406 | 657,041 | 263,911 | 1,009,372 |
Reconstruction | ||||
EMDB code | EMD-27548 | EMD-27549 | EMD-27572 | EMD-27565 |
Box size | 350 | 256 | 256 | 256 |
Symmetry | helical | helical | C1 | C1 |
Map sharpening B-factor (Å2) | –90 | –149 | –81 | –201 |
Resolution (global) (Å) | 3.37 | 3.07 | 2.99 | 3.38 |
Structure building and validation | ||||
PDB ID | 8 DMX | 8DMY | 8DNH | 8DNF |
Model building | Coot | Coot | Coot | Coot |
Refinement program | Phenix | Phenix | Phenix | Phenix |
Refinement target | Real-space | Real-space | Real-space | Real-space |
RMSD from ideal values | ||||
Bond length (Å) | 0.02 | 0.02 | 0.04 | 0.03 |
Bond Angles (0) | 0.493 | 0.494 | 0.761 | 0.711 |
Ramachandran favored (%) | 97.61 | 96.68 | 96.21 | 96.20 |
Ramachandran allowed (%) | 2.39 | 3.32 | 3.79 | 3.46 |
Ramachandran outliers (%) | 0 | 0 | 0 | 0.34 |
MolProbity Score | 1.42 | 1.42 | 1.72 | 1.72 |
Structures Characteristics | ||||
Species | Rabbit | Bovine | Human | Human |
Amino acid resolved | 4–375 | 2–375 | 1–374 | 1–374 |
PTMs resolved | H73 | H73 | D1/H72 | E1/H72 |
Reagent type (species) or resource | Designation | Source or reference | Identifiers | Additional information |
---|---|---|---|---|
Strain, strain background (P. pastoris) | P. pastoris transformants | https://journals.biologists.com/jcs/article/131/8/jcs213827/57192/Rapid-production-of-pure-recombinant-actin | Hatano et al., 2018; P. pastoris transformants used to prepare recombinant b-actin (H. sapiens) and g-actin (H. sapiens) | |
Biological sample (O. cuniculus) | Skeletal muscle | Pel-Freez | 41995 | Muscle acetone powder used for the preparation of native skeletal muscle a-actin |
Biological sample (B. taurus) | heart | Local butcher | Left ventricle used for the preparation of native cardiac muscle a-actin | |
Other (O. cuniculus) | skeletal muscle a-actin | This paper | UniProt ID: P68135 | Peptide, protein reagent, prepared from muscle acetone powder |
Other (B. taurus) | cardiac muscle a-actin | This paper | UniProt ID: Q3ZC07 | Peptide, protein reagent, prepared from bovine heart |
Peptide, recombinant protein (H. sapiens) | b-actin | This paper | UniProt ID: P60709 | Prepared from P. pastoris transformants |
Peptide, recombinant protein (H. sapiens) | g-actin | This paper | UniProt ID: P63261 | Prepared from P. pastoris transformants |
Other | Amicon 30 kDa MWCO centrifugal filters | Millipore Sigma | UFC903008 | Protein concentrators |
Other | C-flat Au 1.2/1.3 grids | Electron Microscopy Sciences | CF313-50-Au | Electron microscopy grids |
Software, algorithm | EPU | Thermo Fisher Scientific | Software for cryo-EM data acquisition | |
Software, algorithm | cryoSPARC | https://doi.org/10.1038/nmeth.4169 | Punjani et al., 2017 | |
Software, algorithm | MotionCor2 | https://doi.org/10.1038/nmeth.4193 | Zheng et al., 2017 | |
Software, algorithm | Coot | https://doi.org/10.1107/S0907444910007493 | Emsley et al., 2010 | |
Software, algorithm | PHENIX | https://doi.org/10.1107/S0907444909052925 | Adams et al., 2010 | |
Software, algorithm | Chimera | https://doi.org/10.1002/jcc.20084 | Pettersen et al., 2004 | |
Software, algorithm | MolProbity | https://doi.org/10.1002/pro.3330 | Williams et al., 2018 |