A role for the centrosome in regulating the rate of neuronal efferocytosis by microglia in vivo
Figures
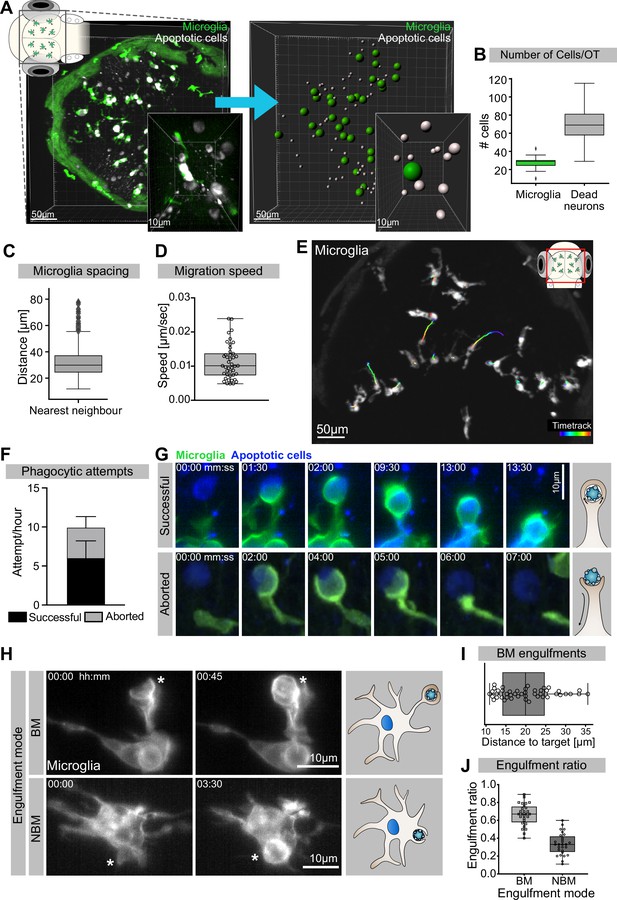
Mechanisms of neuronal efferocytosis by microglia.
(A) Left: representative image of the OT of a 3.5-day post-fertilization (dpf) embryo with microglia (green; Tg(mpeg1:eGFP-caax)) and dying neurons (grey; Tg(nbt:dLexPR-LexOP:secA5-BFP)). Right: automated spot detection of left image to determine the spatial position of microglia and apoptotic cells. (B) Number of microglia and dead neurons within the OT (N = 31). (C) Distance from each microglia to its closest neighbour (N = 31). (D) Microglia migration speed, measured by tracking microglia for 2 hr (N = 2, 20–23 microglia analysed per zebrafish). (E) A representative image of a 3-dpf zebrafish brain, showing microglia (grey; Tg(mpeg1:eGFP-caax)) and their trajectory/track over 1 hr. (F) The total number of phagocytic attempts initiated by microglia per hour; stacked barplot shows the proportion of successful and aborted phagocytic attempts (N = 4, 5–9 microglia analysed per fish). (G) Upper panel: microglia (green; Tg(mpeg1:eGFP-caax)) phagocytic cup that results in the successful formation of a phagosome around a dead neuron (blue; Tg(nbt:dLexPR-LexOP:secA5-BFP)). Lower panel: phagocytic cup formation where the phagocytic attempt is aborted. (H) Microglia (grey; Tg(mpeg1:eGFP-caax)) phagocytosis happens at two locations; upper panel: phagosome forms at the tip of a long cellular extension. Lower panel: phagosome forms directly at the cell soma. Full time lapse is found in Figure 1—video 2. (I) Length of successful phagocytic branches during branch-mediated (BM) engulfments (N = 3, n = 7, 5–16) engulfments analysed per microglia. (J) Ratio between BM and non-branch-mediated (NBM) engulfments (N = 4, 5–9 microglia analysed per fish). Bars represent mean +/- SD (F). Boxplots depict mean and 1.5x interquartile range (B, C) or mean +/- min to max values (D, F, I, J). N refers to the number of zebrafish and n to the number of microglia examined.
-
Figure 1—source data 1
Related to Figure 1D.
- https://cdn.elifesciences.org/articles/82094/elife-82094-fig1-data1-v1.xlsx
-
Figure 1—source data 2
Related to Figure 1F and J.
- https://cdn.elifesciences.org/articles/82094/elife-82094-fig1-data2-v1.xlsx
Video showing spatial position and organization of microglia and apoptotic cells.
Video of a representative zebrafish brain with microglia (green; Tg(mpeg1:eGFP-caax)) and dying neurons (grey; Tg(nbt:dLexPR-LexOP:secA5-BFP)) labelled and segmented using automatic spot detection in Imaris. Video shows how microglia are surrounded by many more apoptotic neurons.
Time-lapse showing branch-mediated (BM) and non-branch-mediated (NBM) engulfments by microglia.
Time lapses of representative microglia (grey; Tg(mpeg1:eGFP-caax)) showing how phagocytosis can happen at two locations; Left: phagosome forms at the tip of a long cellular extension (BM engulfment). Right: phagosome forms directly at the cell soma (NBM engulfment). Images were captured every 30 s for 13 min. Timescale is mm:ss.
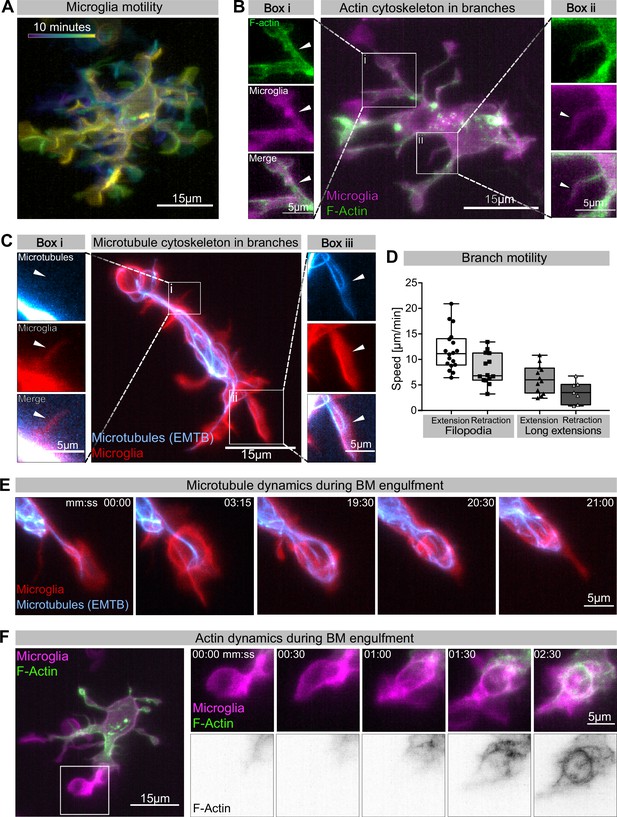
Cytoskeletal dynamics during microglia efferocytosis.
(A) Colour-coded overlay of a 10 min time lapse of microglia Tg(mpeg1:Gal4; UAS:lyn-tagRFPt) showing their branch dynamics. (B) Microglia (magenta; Tg(mpeg1:Gal4; UAS:lyn-tagRFPt)) and F-actin (green; UAS:UtrCH-mNG). Box i shows thicker extensions, and box ii shows thin filopodia, both containing F-actin. (C) Microglia (red; Tg(csf1ra:GAL4-VP16; UAS:lyn-tagRFPt)) and microtubules (cyan; Tg(UAS:EMTB-3xGFP)). Box i shows a thin filopodia, and box ii shows a thicker extension; microtubules are only observed in thicker extensions. The intensity histogram of images in boxes i and ii from (B) and (C) has been adjusted to highlight the weak fluorescent signals of the cytoskeletal components. (D) The speed at which both thick extensions and thin filopodia extend and retract, measured in μm/min (n = 2, 2–10 retractions/extensions analysed per microglia, boxplot depict mean +/- min to max values). (E) The panel shows the same branch as in (C) box ii at a later time point, forming a phagosome. (F) Microglia (magenta; Tg(mpeg1:Gal4; UAS:lyn-tagRFPt)) and F-actin (green; (UAS:UtrCH-mNG)). The panel shows how F-actin is found in the cellular extension and engages with the phagosome soon after this has formed. Full time lapse is found in Figure 2—video 1. n refers to the number of microglia examined.
-
Figure 2—source data 1
Related to Figure 2D.
- https://cdn.elifesciences.org/articles/82094/elife-82094-fig2-data1-v1.xlsx
Time-lapse showing F-actin dynamics during microglia branch-mediated engulfment.
Time lapse of a representative microglia (magenta; Tg(mpeg1:Gal4; UAS:lyn-tagRFPt)) expressing a reporter for F-actin (green; (UAS:UtrCH-mNG)). The video shows how F-actin is found in the cellular extension and engages with the phagosome soon after this has formed. Images were captured every 15 s for 10 min. Timescale is mm:ss.
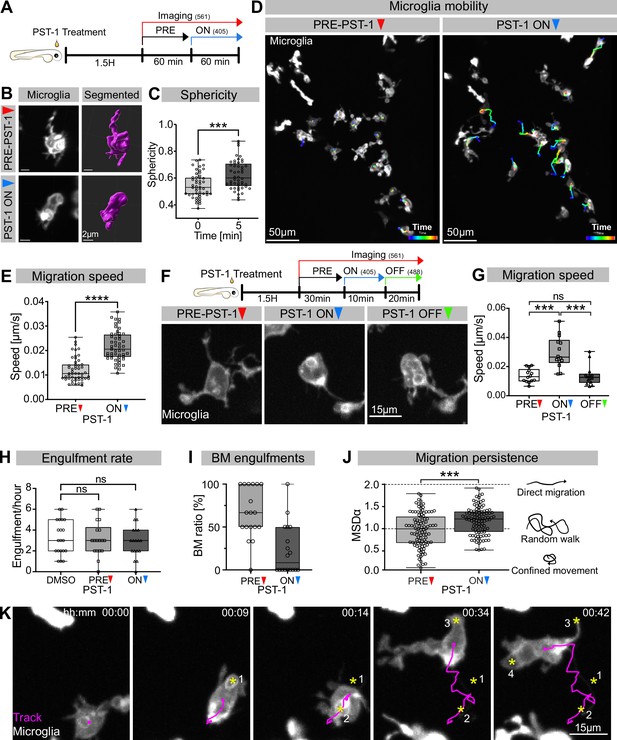
Microglia without dynamic microtubules adopt a different engulfment strategy.
(A) Schematic showing the experimental setup: Embryos were treated for 1.5 hr with PST-1, then imaged for 1 hr with 561 nm light (PRE-PST-1) and then imaged for another hour with 561 nm and 405 nm light to activate PST-1 (PST-1 ON). (B) Left: microglia (grey; Tg(mpeg1:Gal4; UAS:lyn-tagRFPt)) before (upper) and after (lower) PST-1 activation. Right: 3D segmentation of the cells in the left panel. (C) Microglia sphericity before and 5 min after PST-1 activation. Segmentation in (B) was used to extract surface area and volume to calculate sphericity (N = 4, 6–15 microglia analysed per fish, ***p=0.0005). (D) 3D tracking of microglia for 1 hr before (left) and after (right) PST-1 activation, displayed as colour-coded tracks overlayed on top of microscopy images of the microglia. Full time lapse is found in Figure 3—video 1. (E) Microglia migration speed measured in μm/s (N = 4, 6–18 microglia analysed per fish, ****p<0.0001). (F) Upper: schematic of the ON-OFF experiment. Lower: microglia (grey; Tg(mpeg1:Gal4; UAS:lyn-tagRFPt)) shown before PST-1 activation (PRE-PST-1) and when the drug is turned ON with 405 nm light (PST-1-ON) and OFF again using 488 nm light (PST-1-OFF). A paired, non-parametric Friedman test with Dunn’s correction was used to compare the three groups (***p=0.0002). (G) Microglia migration speed during the ON-OFF experiment, measured in μm/s (N = 1, n = 14). (H) Microglia engulfment rate after DMSO (N = 3, 5–8 microglia analysed per fish) or PST-1 treatment (N = 3, 6 microglia analysed per fish). (I) Percentage of branch-mediated (BM) engulfments before (left) and after (right) activation of PST-1 (N = 3, 6 microglia analysed per fish). (J) Left: microglia migration persistence measured with the mean square displacement alpha (MSDα) before and after PST-1 activation (N = 4, 6–18 microglia analysed per fish, ***p=0.0001) Right: schematic of what the MSDα values imply. (K) Representative time lapse of a microglia (grey) treated with activated PST-1 and tracked (magenta), showing how microglia migrate towards apoptotic targets (yellow asterisk). Boxplots depict mean +/- min to max values. Groups were compared using a two-tailed, nonparametric Mann-Whitney U-test was used (C, E, H, J). When comparing reapeated measurements from the same group, a nonparametric Friedmann test with a Dunn's correction was used (G). N refers to the number of zebrafish and n to the number of microglia examined.
-
Figure 3—source data 1
Related to Figure 3C.
- https://cdn.elifesciences.org/articles/82094/elife-82094-fig3-data1-v1.xlsx
-
Figure 3—source data 2
Related to Figure 3E.
- https://cdn.elifesciences.org/articles/82094/elife-82094-fig3-data2-v1.xlsx
-
Figure 3—source data 3
Related to Figure 3G.
- https://cdn.elifesciences.org/articles/82094/elife-82094-fig3-data3-v1.xlsx
-
Figure 3—source data 4
Related to Figure 3H and I.
- https://cdn.elifesciences.org/articles/82094/elife-82094-fig3-data4-v1.xlsx
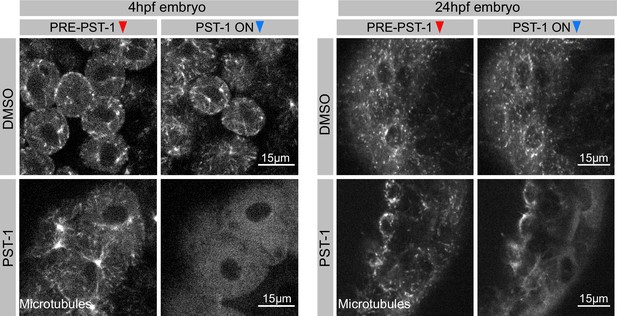
Validation of PST-1 functionality in vivo.
Zebrafish embryo’s injected with EB3-mScarlett-I mRNA, treated with 1%. DMSO (top panel) or 1 mM PST-1 (bottom panel) at two different developmental stages. Growing tips of microtubules are visualized as grey comets. Left panel: 4-hr-post-fertilization (hpf) embryos before and after 405 nm light illumination. Right panel: 24-hpf embryos before and after 405 nm light activation. Images were captured using spinning-disc microscopy.

Nocodazole and PST-1-treated microglia behave similarly.
(A) Microglia (grey; mpeg1:Gal4; UAS:lyn-tagRFPt) treated with DMSO (left) or 10 mM nocodazole for 2 hr. Engulfment rate (B) and mode (C) of microglia treated with either DMSO (N = 2, n = 7) or nocodazole (N = 1, n = 9). Boxplots depict mean +/- min to max values. Groups were compared using a two-tailed, nonparametric Mann-Whitney U-test. N refers to the number of zebrafish and n to the number of microglia examined.
-
Figure 3—figure supplement 2—source data 1
Related to Figure 3—figure supplement 2B and C.
- https://cdn.elifesciences.org/articles/82094/elife-82094-fig3-figsupp2-data1-v1.xlsx
Microglia without dynamic microtubules become more mobile.
Time lapse of a representative zebrafish brain showing microglia (grey; mpeg1:Gal4; UAS:lyn-tagRFPt) treated with PST-1 before (left; Pre-PST-1) or after (right; PST-1-ON) activation with 405 nm light. 3D tracking of microglia is displayed as colour-coded tracks overlayed on top of the microscopy images. Images were captured every minute for 2 hr total using spinning-disc microscopy. Timescale is hh:mm.
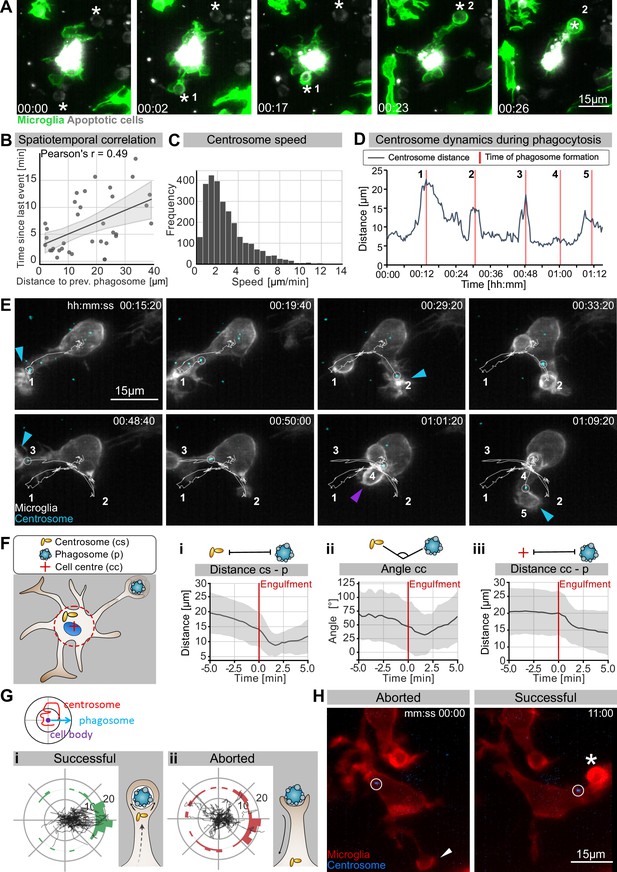
The microglia centrosome is dynamic and moves into successful phagocytic branches.
(A) Sequential uptake of two apoptotic neurons (grey; Tg(nbt:secA5-BPF)) by microglia (green; Tg(mpeg1:Gal4; UAS:lyn-tagRFPt)). Timescale is hh:mm. (B) Correlation of spatiotemporal distances between phagocytic events (N = 3, n = 7, 5–16 engulfments analysed per microglia, 95% confidence interval is depicted). (C) Speed of centrosomal movements, relative to the cell centre (N = 3, 2–3 microglia analysed per fish). (D) Movement of the centrosome, relative to the cell centre of the microglia in (E). The track shows the distance of the centrosome from the cell centre, and the red lines indicate the time of phagosome formation. (E) Time lapse of a microglia (grey; Tg(csf1ra:GAL4-VP16; UAS:lyn-tagRFPt)) and the centrosome (cyan; Tg(UAS:miRFP670-cetn4)). The microglial centrosome is encircled and tracked for 1 hr 15 min; phagocytic events are numbered and labelled; branch-mediated (BM) engulfments are indicated with a blue arrow and non-branch-mediated (NBM) engulfments are indicated with a purple arrow. Full time lapse is found in Video 5. (F) Schematic of how the centrosome (cs), cell centre (cc), and location of newly formed phagosomes (p) were tracked across many samples. (i) cs distance from cb, (ii) angle between cs and p, and (iii) distance of p from cc for 5 min before and after phagosome formation (N = 7, n = 7, 5–16 engulfments analysed per microglia, the mean +/- SD is depicted). (G) Top: schematic of how the centrosome, cell centre, and location of a newly formed phagosomes are oriented in the polar plots. Bottom: individual tracks of the centrosome relative to the cell body and the phagosome during (i) successful and (ii) aborted phagocytic attempts (N = 3, n = 7, 5–16 successful and 2–12 aborted attempts analysed per microglia). (H) Microglia (grey) and the centrosome (cyan) where the centrosome is encircled during an aborted (left, arrowhead) and a successful event (right, asterisk). Original image was processed with a Gaussian Blur filter with sigma(radius) = 1. N refers to the number of zebrafish and n to the number of microglia examined.
-
Figure 4—source data 1
Related to Figure 4D.
- https://cdn.elifesciences.org/articles/82094/elife-82094-fig4-data1-v1.xlsx
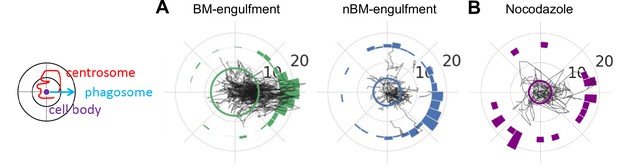
The microglia centrosome only moves towards branch-mediated (BM) phagosomes.
Left: schematic of how the centrosome, cell centre, and location of newly formed phagosomes are oriented in the polar plots. (A–, B) Individual tracks of the centrosome relative to the cell body and the phagosome during BM (left) and non-branch-mediated (NBM) (right) engulfments in DMSO-treated embryos (N = 4, 4–9 microglia analysed per fish) (A) and all engulfments in embryos treated with 10 mM nocodazole (N = 3, 2–3 microglia analysed per fish) for 2 hr (B). N refers to the number of zebrafish examined.
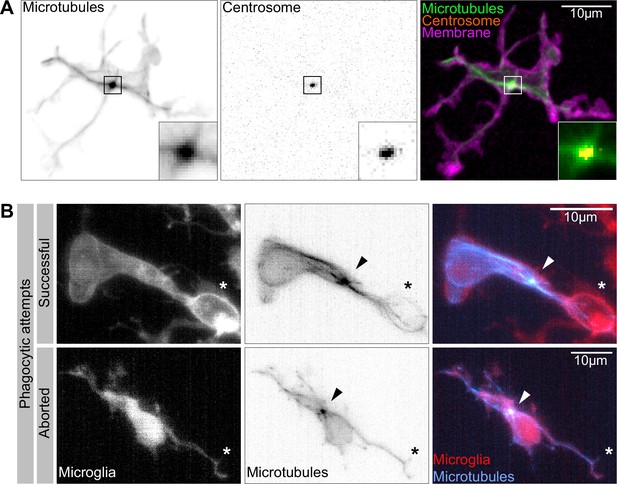
The microglia centrosome is a microtubule organization centre (MTOC) and moves into successful phagocytic branches.
(A) Macrophage (magenta; Tg(csf1ra:GAL4-VP16; UAS:lyn-tagRFPt)) with the centrosome (UAS:miRFP670-cetn4) and microtubules (green; Tg(UAS:EB3-GFP)) labelled. The centrosome can be seen right in the centre of the MTOC (box). (B) Microglia (grey; Tg(csf1ra:GAL4-VP16; UAS:lyn-tagRFPt)) with microtubules (cyan; Tg(UAS:EB3-GFP)) labelled. The MTOC can be seen as a bright spot of growing microtubules (arrow). Top: a successful phagocytic event, full time lapse in Figure 5—video 2. The newly formed phagosome is marked with an asterisk. Bottom: an aborted attempt marked with an asterisk.
The microglia microtubule organization centre (MTOC) visualized as a dense cluster of EB3 comets.
Time lapse of a representative microglia expressing the EB3 plus-end microtubule reporter (black; Tg(csf1ra:Gal4-VP16; UAS:EB3-GFP)) showing the MTOC as a dense cluster of growing microtubule tips. Images were captured every 15 s for 10 min using single-plane illumination microscopy (SPIM). Timescale is mm:ss.
The microglia microtubule organization centre (MTOC) moves into successful phagocytic branches.
Time lapse of a representative microglia (grey; Tg(csf1ra:GAL4-VP16; UAS:lyn-tagRFPt)), expressing the EB3 plus-end microtubule reporter (cyan; Tg(UAS:EB3-GFP)). The MTOC can be seen as a bright spot of growing microtubules that moves into the phagocytic branch during phagocytosis. Images were captured every 20 s for 35 min. Timescale is hh:mm:ss.
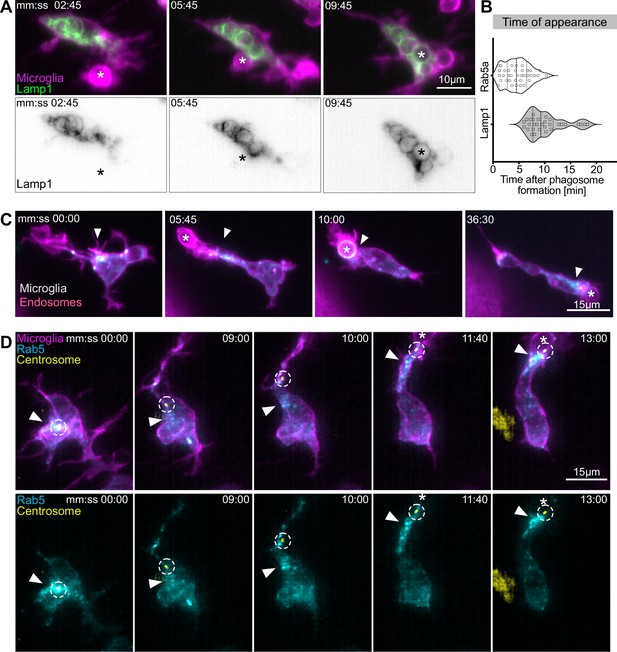
Movement of the centrosome correlates with targeted vesicular trafficking towards phagosomes.
(A) Microglia (magenta; Tg(csf1ra:GAL4-VP16; UAS:lyn-tagRFPt)) and Lamp1 labelled vesicles (green; Tg(UAS:Lamp1-mGFP)) localized within the cell soma and not moving into branches. (B) Time of Rab5 (n = 3, 8–10 phagocytic events analysed per microglia) and Lamp1 (n = 3, 9–11 phagocytic events analysed per microglia) appearance at phagosome, after its formation. Violin plots have mean and quartiles depicted. (C) Microglia (magenta; Tg(csf1ra:GAL4-VP16; UAS:lyn-tagRFPt)) and Rab5-positive endosomes (cyan; UAS:mNeonGreen-Rab5a), full time lapse in Figure 6—video 1. Endosomes form a cloud (arrowhead) that moves into phagocytic branches towards forming phagosomes (asterisk). Original images were deconvolved using Huygens deconvolution. (D) Representative microglia (magenta; Tg(csf1ra:GAL4-VP16; UAS:lyn-tagRFPt)), Rab5 endosomes (cyan; UAS:mNeonGreen-Rab5a), and centrosome (yellow; UAS:miRFP670-centrin4). The centrosome (circle) moves into phagocytic branches (star) and is followed by the cloud of Rab5 endosomes (arrowhead). Original images were deconvolved using Huygens deconvolution. n refers to the number of microglia examined.
-
Figure 6—source data 1
Related to Figure 6B.
- https://cdn.elifesciences.org/articles/82094/elife-82094-fig6-data1-v1.xlsx
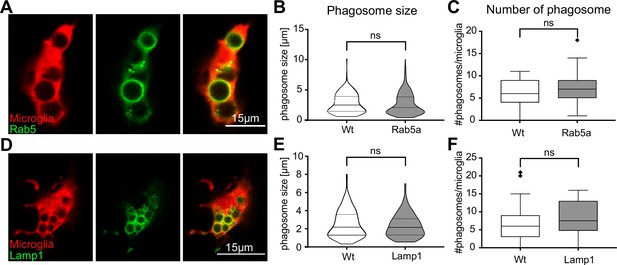
Vesicular markers do not affect microglia phagocytosis.
(A) A single-image plane showing microglia (red) expressing Rab5 (green) on newly formed phagosomes (fms:Gal4-VP16; UAS:nfsB-mCherry; UAS:mNeonGreen-Rab5a). Size (B) and number (C) of phagosomes from same brain (N = 11) microglia, with (n = 62) or without (n = 29) Rab5 expression (1–18 phagosomes analysed per microglia). (D) A single-image plane showing microglia (red) expressing Lamp1 (green) on vesicles (fms:Gal4-VP16; UAS:nfsB-mCherry; UAS:Lamp1-mGFP). Size (E) and number (F) of phagosomes from same brain (N = 10) microglia, with (n = 17) or without (n = 48) Lamp1 expression (1–21 phagosomes analysed per microglia). N refers to the number of zebrafish and n to the number of microglia examined. Violin plots show mean and quartiles, boxplots depict mean +/- 95% confidence intervals. Groups were compared using a two-tailed, nonparametric Mann-Whitney U-test.
-
Figure 6—figure supplement 1—source data 1
Related to Figure 6—figure supplement 1B-C and E-F.
- https://cdn.elifesciences.org/articles/82094/elife-82094-fig6-figsupp1-data1-v1.xlsx
Rab5-positive endosomal cloud is highly dynamic and moves into phagocytic branches.
Time lapse of a representative microglia (grey; Tg(csf1ra:GAL4-VP16; UAS:lyn-tagRFPt)) and Rab5-positive endosomes (magma; UAS:mNeonGreen-Rab5a) showing how endosomes form a cloud that moves into phagocytic branches towards forming phagosomes. Original images were deconvolved using Huygens deconvolution. Images were captured every 15 s for 1 hr. Timescale is hh:mm:ss.
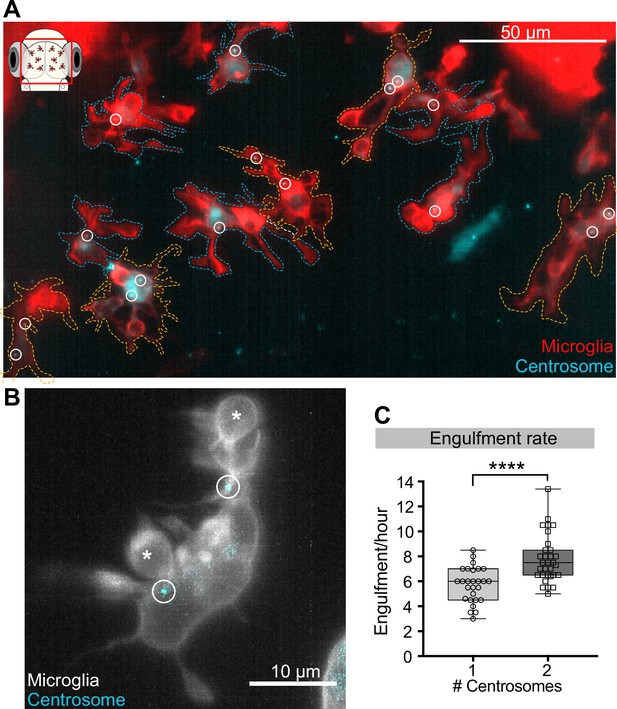
Microglia with two centrosomes are more efficient phagocytes.
(A) Microglia (red; Tg(csf1ra:GAL4-VP16; UAS:lyn-tagRFPt)) overexpressing the centrosome marker (cyan; Tg(UAS:miRFP670-cetn4)). Due to mosaicism in the overexpression of UAS:miRFP670-cetn4, there are microglia (dashed outlines) with two centrosomes (orange cells) and matching controls with only one (blue cells) within the same brain. Centrosomes are labelled with circles. (B) Microglia (grey) overexpressing the centrosome marker (cyan) resulting in this cell having two centrosomes (circle). (C) Microglia with two centrosomes engulf more neurons (N = 8, 2–9 microglia analysed per fish). Boxplots depict mean +/- min and max values. Groups were compared using a two-tailed, nonparametric Mann-Whitney U test, ****p<0.0001. N refers to the number of zebrafish analysed.
-
Figure 7—source data 1
Related to Figure 7C.
- https://cdn.elifesciences.org/articles/82094/elife-82094-fig7-data1-v1.xlsx
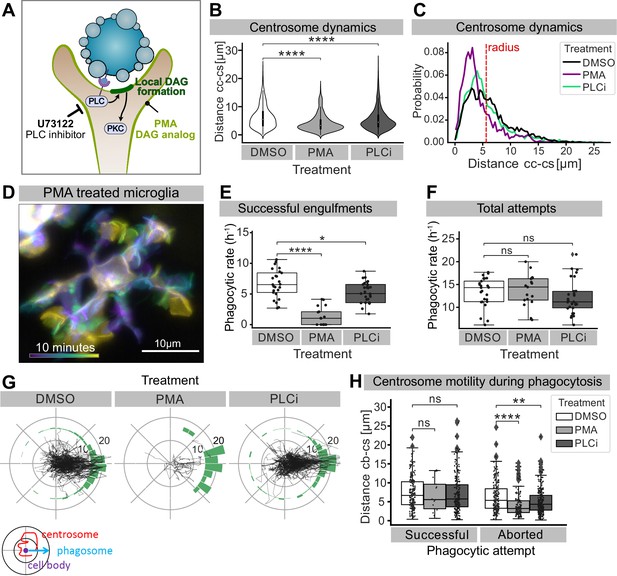
Perturbing DAG signalling affects centrosomal motility and phagocytosis.
(A) Schematic showing the effect of phorbol 12-myristate 13-acetate (PMA) and pan-inhibitor of PLC (PLCi) on DAG-mediated signalling. (B) Distance of the centrosome from the cell centre in microglia after 1% DMSO (N = 4, n = 26), 2.5 μM PMA (N = 3, n = 17) or 1 μM U73122 (N = 4, n = 29) treatment. (C) Probability map of the radial location of the centrosome, relative to the cell centre, after DMSO, PMA, or PLCi treatment. (D) A colour-coded overlay of a 10 min time lapse of a representative microglia (Tg(mpeg1:Gal4; UAS:lyn-tagRFPt)) treated with 2.5 μM PMA, to show branch dynamics. (E, F) Phagocytic attempts by microglia treated with 1% DMSO (white), 2.5 μM PMA (light grey) or 1 μM U73122 (dark grey). Plots show (E) successful and (F) total phagocytic attempts (*p<0.05, ****p<0.0001). (G) Tracks of the centrosome relative to successful phagocytic events, after DMSO, PMA, or PLCi treatment. Histograms summarize the position of the centrosome at the time of phagosome formation. (H) Centrosome distance from cell centre at the time of successful or aborted phagocytic attempts, after DMSO, PMA, or PLCi treatment (*p<0.05, **p<0.01, ***p<0.001, ****p<0.0001). Violin and boxplots depict mean and 1.5x interquartile range. Groups were compared using an unpaired, nonparametric Kruskal-Wallis with Bonferroni correction. N refers to the number of zebrafish and n to the total number of microglia analysed.

The radius of microglia cell soma.
Left: schematic of how the radius of a microglia soma was determined. Right: a boxplot showing the radius of individual microglia treated with DMSO (N = 2, 7–10 microglia analysed per fish), Pan-inhibitor of PLC (PLCi) (N = 2, 5–8 microglia analysed per fish) or phorbol 12-myristate 13-acetate (PMA) (N = 2, 5–8 microglia analysed per fish). Boxplots depict the mean +/- min and max values. N refers to the number of zebrafish analysed.
-
Figure 8—figure supplement 1—source data 1
Related to Figure 8—figure supplement 1.
- https://cdn.elifesciences.org/articles/82094/elife-82094-fig8-figsupp1-data1-v1.xlsx
Videos
Microglia phagocytic attempts can be successful or aborted.
Representative microglia (green; Tg(csf1ra:GAL4-VP16; UAS:lyn-tagRFPt)) making successful (left) and aborted (right) phagocytic attempts. Apoptotic neurons are in blue (Tg(nbt:dLexPR-LexOP:secA5-BFP)). Images were acquired every 30 s using single-plane illumination microscopy (SPIM). Timescale is mm:ss.
Microtubule dynamics during microglia branch-mediated engulfment.
Representative microglia (red; Tg(csf1ra:GAL4-VP16; UAS:lyn-tagRFPt)) expressing a microtubule reporter (cyan; Tg(UAS:EMTB-3xGFP)). Images were captured every 15 s for 32 min using single-plane illumination microscopy (SPIM). Timescale is mm:ss.
Without dynamic microtubules, microglia become amoeboid and mobile.
Representative time lapse of microglia (black; Tg(mpeg1:Gal4; UAS:lyn-tagRFPt)) treated with PST-1, before (Pre-PST-1; first hour), and after (PST-1-ON; second hour) PST-1 activation using 405 nm light. Images were captured every minute for 2 hr using spinning-disc microscopy. Timescale is hh:mm.
Microglia engulf dead neurons sequentially.
Time lapse of a representative microglia (grey; Tg(csf1ra:GAL4-VP16; UAS:lyn-tagRFPt)) capturing apoptotic neurons (not labelled) sequentially. Phagocytic events are marked with an asterisk. Images were captured every 30 s for 1,5 hr using single-plane illumination microscopy (SPIM). The original time lapse was deconvolved using Huygens deconvolution. Time scale is hh:mm:ss.
The microglia centrosome is motile and moves into phagocytic branches.
Representative microglia (red; Tg(csf1ra:GAL4-VP16; UAS:lyn-tagRFPt)) expressing the centrosome reporter (cyan; Tg(UAS:miRFP670-cetn4)). The microglial centrosome is marked with a circle as centrosomes from surrounding cells can also be seen in the projection. Images were captured every 20 s for 1 hr 40 min using single-plane illumination microscopy (SPIM). The original time lapse was deconvolved using Huygens deconvolution. Time scale is hh:mm:ss.
Rab5-positive early endosomes form a cloud that follows the centrosome into phagocytic branches.
Right: representative microglia (magenta; Tg(csf1ra:GAL4-VP16; UAS:lyn-tagRFPt)), expressing the Rab5 endosome (cyan; UAS:mNeonGreen-Rab5a) and centrosome (yellow; UAS:miRFP670-centrin4) reporters. Left: image segmentation showing tracking of the centrosome (red dot) and a binary threshold view of the Rab5 signal (black pixels) to show cloud dynamics. Images were captured every 15 s for 30 min using single-plane illumination microscopy (SPIM). Timescale is hh:mm:ss.
Microglia with two centrosomes.
Representative microglia (red; Tg(csf1ra:GAL4-VP16; UAS:lyn-tagRFPt)) overexpressing the centrosome reporter (cyan; Tg(UAS:miRFP670-cetn4)) resulting in two centrosomes. Images were captured every 20 s for 40 min using single-plane illumination microscopy (SPIM). Timescale is hh:mm:ss.
Microglia centrosome is retained within the cell soma after phorbol 12-myristate 13-acetate (PMA) and pan-inhibitor of PLC (PLCi) treatment.
Representative microglia treated with DMSO, PMA, and PLCi (grey; Tg(csf1ra:GAL4-VP16; UAS:lyn-tagRFPt)) expressing the centrosome reporter (cyan; Tg(UAS:miRFP670-cetn4)). Images were captured every 30 s for 1 hr using single-plane illumination microscopy (SPIM). Original images were processed with a Gaussian Blur filter with sigma(radius) = 1. Timescale is mm:ss.
Tables
Reagent type (species) or resource | Designation | Source or reference | Identifiers | Additional information |
---|---|---|---|---|
Genetic reagent (Danio rerio) | Tg(mpeg1.1:EGFP-caax) | Villani et al., 2019 | ZFIN ID: ZDB- TGCONSTRCT-191211-1 | Macrophage membrane labelling |
Genetic reagent (D. rerio) | Tg(mpeg1.1:Gal4-VP16) | Ellett et al., 2011 | ZFIN ID: ZDB-ALT-120117-3 | Macrophage Gal4 driver line |
Genetic reagent (D. rerio) | TgBAC(csf1ra:Gal4-VP16)i186 | Gray et al., 2011 | ZFIN ID: ZDB-ALT-110707-2 | Macrophage Gal4 driver line |
Genetic reagent (D. rerio) | Tg(Xla.Tubb:LEXPR-SEC-Hsa.ANXA5-TagBFP) | Mazaheri et al., 2014 | ZFIN ID: ZDB-ALT-170110-1 | Reporter line for apoptotic neurons |
Genetic reagent (D. rerio) | Tg(UAS:EMTB-3xGFP) | Revenu et al., 2014 | UAS-driven microtubule reporter | |
Genetic reagent (D. rerio) | Tg(UAS:EB3-GFP) | Fassier et al., 2018 | UAS-driven microtubule reporter | |
Genetic reagent (D. rerio) | Tg(UAS:mNeonGreen-UtrCH) | Shkarina et al., 2021 | UAS-driven actin reporter | |
Genetic reagent (D. rerio) | Tg(UAS:lyn-tagRFPT) | This paper | UAS-driven red membrane labelling | |
Genetic reagent (D. rerio) | Tg(UAS:mNeonGreen-Rab5a) | This paper | UAS-driven early endosomal reporter | |
Genetic reagent (D. rerio) | Tg(UAS:miRFP670-cetn4) | This paper | UAS-driven centrosomal reporter | |
Genetic reagent (D. rerio) | Tg(UAS:Lamp1-mGFP) | This paper | UAS-driven lysosomal reporter | |
Genetic reagent (D. rerio) | Tg(UAS:EB3-mScarlet-I) | This paper | UAS-driven microtubule reporter | |
Recombinant DNA reagent | UAS:EB3-mScarlet-I (plasmid) | This paper | RRID:Addgene_192358 | Made with tol2kit, see section ‘Cloning of plasmids’ |
Sequence-based reagent | Sp6-EB3-mScarlet_Fw | This paper | PCR primers | ATTTAGGTGACACTATAGAAGATGGCCGTCAATGTGTAC |
Sequence-based reagent | Sp6-EB3-mScarlet_Rev | This paper | PCR primers | CAACTATGTATAATAAAGTTG |
Commercial assay or kit | MultiSite Gateway Pro | Invitrogen | #12537 | |
Commercial assay or kit | Gibson Assembly Master Mix | New England Biolabs | #E2611 | |
Commercial assay or kit | pENTR/D-TOPO Cloning Kit | Invitrogen | # K240020SP | |
Commercial assay or kit | mMESSAGE mMACHINE SP6 Transcription Kit | Invitrogen | #AM1340 | |
Commercial assay or kit | MinElute Gel extraction kit | QIAGEN | #28604 | |
Commercial assay or kit | GeneJet RNA Cleanup and Concentration kit | Thermo Fisher Scientific | #K0841 | |
Chemical compound, drug | N-Phenylthiourea (PTU) | Sigma-Aldrich | #P7629 | |
Chemical compound, drug | Tricaine (Mesab) | Sigma-Aldrich | #A5040 | |
Chemical compound, drug | DMSO | Sigma-Aldrich | #D8418 | |
Chemical compound, drug | Nocodazole | Sigma-Aldrich | #M1404 | |
Chemical compound, drug | Photostatin-1 (PST-1) | Borowiak et al., 2015 | ||
Chemical compound, drug | Phorbol 12-myristate 13-acetate (PMA) | Sigma-Aldrich | #P8139 | |
Chemical compound, drug | U73122 (PLC inhibitor) | Tocris | #U73122 | |
Software, algorithm | Prism 9 | GraphPad | RRID:SCR_002798 | |
Software, algorithm | Python 3.7 | Python Software Foundation | RRID:SCR_008394 | https://www.python.org/psf/ |
Software, algorithm | Matplotlib 3.4.1 | Hunter, 2007 | RRID:SCR_008624 | |
Software, algorithm | NumPy 1.20 | Harris et al., 2020 | RRID:SCR_008633 | |
Software, algorithm | SciPy 1.4 | Virtanen et al., 2020 | RRID:SCR_008058 | |
Software, algorithm | Pandas 1.2.4 | McKinney, 2010 | RRID:SCR_018214 | |
Software, algorithm | Seaborn 0.11.1 | Waskom, 2021 | RRID:SCR_018132 | |
Software, algorithm | Imaris 9.5 | Bitplane | RRID:SCR_007370 | https://imaris.oxinst.com/ |
Software, algorithm | Fiji | Schindelin et al., 2012 | RRID:SCR_002285 | imagej.net/Fiji |
Software, algorithm | BigDataProcessor2 (ImageJ plugin) | Tischer et al., 2021 | https://github.com/bigdataprocessor/bigdataprocessor2 | |
Software, algorithm | MtrackJ (ImageJ plugin) | Meijering et al., 2012 | https://github.com/imagescience/MTrackJ/ | |
Software, algorithm | HyperStackReg (ImageJ plugin) | Sharma, 2018 | https://github.com/ved-sharma/HyperStackReg/tree/v5.6 | |
Software, algorithm | Z-stackDepthColorCode (ImageJ plugin) | https://sites.imagej.net/Ekatrukha/ | ||
Software, algorithm | Analysis code | Max Brambach | https://github.com/max-brambach/microglia_centrosome |