A pathogenic human Orai1 mutation unmasks STIM1-independent rapid inactivation of Orai1 channels
Figures
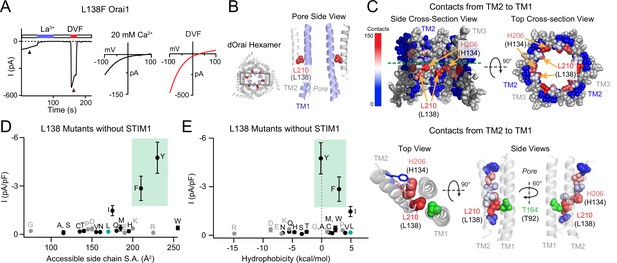
The GOF activity of L138 mutants depends on the size and shape of the introduced side chain.
(A) Time course of the constitutively active L138F Orai1 current in the absence of STIM1. The trace shows a recording from a HEK293 cell expressing L138F Orai1. At whole-cell break-in (t=0 s), a large standing inward Ca2+ current in 20 mM Ca2+ external solution is observed which decreases in amplitude over the first 50 s due to current rundown. Replacing the standard external Ringer’s solution with a divalent-free (DVF) solution reveals a large Na+ current. The current-voltage (I-V) plots from voltage ramps (–100 mV to +100 mV) are shown on the right. (B) Side-view of TM2 residue L210 (hOrai1 L138, red) in the dOrai crystal structure (PDB ID: 4HKR). For simplicity, only two TM1 helices (blue) and two TM2 helices (grey) are shown. Human Orai1 numbering is shown in parentheses. (C) Atomic packing analysis of dOrai (PDB ID: 4HKR) showing a heat map of contacts of made by TM2 towards TM1 at the TM2/3 ring-TM1 interface. TM3 is shown in grey and TM4 is hidden for clarity. Residues are colored based on the heat map scale shown on the left that indexes the number of contacts (Yeung et al., 2018). In the bottom panel, TMs 1 and 2 are shown as ribbons, with TM2 residues that face TM1 shown in spheres colored according to the number of contacts made with TM1. Residue T92 is shown in green to highlight its proximity to L138. (D–E) Current densities of L138 mutants in the absence of STIM1 co-expression plotted against amino acid side-chain surface area and hydrophobicity. L138F/Y channels are open even without STIM1 while small and flexible substitutions produce loss-of-function channels that cannot be gated by STIM1. Green shading on these plots highlight the GOF mutants L138F and L138Y. Grey dots denote amino acids (charged or Pro/Gly) which have the potential to disrupt the helical structure of TM2. N=4–7 cells. Values are mean ± S.E.M. The intracellular solution used was the standard internal solution with 8 mM BAPTA as the Ca2+ buffer (see Materials and methods).
-
Figure 1—source data 1
Mutational analysis and contact dots analysis of the Orai1 L138 locus.
- https://cdn.elifesciences.org/articles/82281/elife-82281-fig1-data1-v2.docx
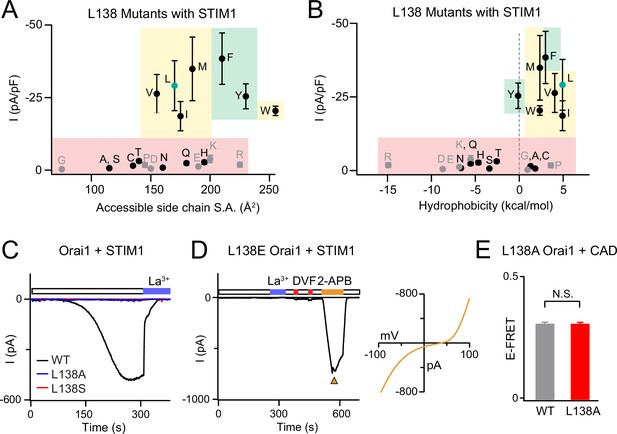
Analysis of L138 Orai1 currents in the presence of STIM1.
(A–B) Current densities of L138 mutants expressed with STIM1 are plotted against size chain size and hydrophobicity. Small and flexible substitutions produce LOF channels. Green, yellow, and red shading highlights GOF mutants, store-operated, and LOF mutants, respectively. N=4–7 cells for each point. Values are mean ± S.E.M. (C) Unlike WT Orai1, L138A and L138S mutants do are not activated by STIM1. In each case, the indicated Orai1 variant was expressed together with STIM1 and currents were recorded following dialysis of 8 mM intracellular BAPTA. (D) The L138E mutant cannot be gated by STIM1 but is strongly activated by Orai1 modulator 2-APB (50 μM). (E) There is no defect in L138A Orai1 interaction with CAD as measured by E-FRET between L138A Orai1-YFP and CFP-CAD, indicating that loss of gating in the L138A mutant is not due to lack of membrane expression or defect in interaction with STIM1. N=51–57 cells. Values are mean ± S.E.M.
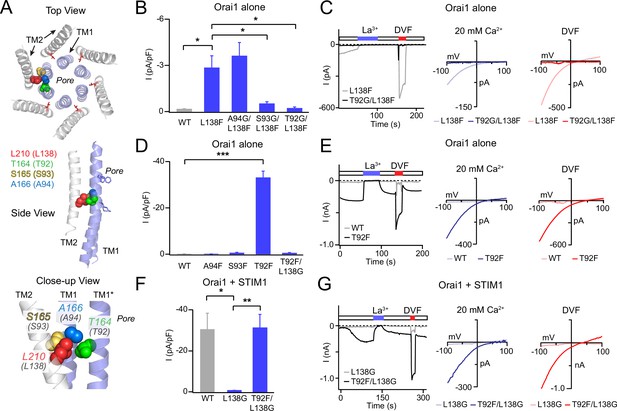
Analysis of L138-TM1 double mutants indicates that L138 interacts with T92.
(A) Three views of L210 (hOrai1 L138, red) in the dOrai crystal structure (PDB ID: 4HKR) surrounded by the nearby TM1 residue T164 (hOrai1 T92, green) from a neighboring subunit, and S165 (hOrai1 S93, yellow) and A166 (hOrai1 A94, blue) from the same subunit. (B) The Orai1 L138F GOF phenotype is reversed by the glycine substitutions at T92 and S93, but not by a Gly substitution at A94. The bar graph plots the current density in the indicated mutants. (C) Time course and current-voltage relationships highlighting the reversal of the L138F GOF phenotype by T92G. The L138F single mutant trace and I-V plots are the same as those shown for L138F in Figure 1A. (D) Introduction of a Phe residue at T92, but not at A94 or S93 produces constitutively active GOF channels. This GOF phenotype of T92F is completely reversed by neutralizing the bulky Phe residue at 92 with a Gly substitution at L138. The bar graph shows the current densities in the indicated mutants. (E) Example traces of T92F or WT Orai1 currents expressed in the absence of STIM1. T92F evokes a constitutively active, Ca2+-selective current. (F–G) Loss of STIM1-mediated gating of L138G is reversed by a Phe substitution at T92 (L138G/T92F mutant), suggesting that the total size chain size at this locus is crucial for channel activation. N=4–6 cells. The intracellular solution used was the standard internal solution with 8 mM BAPTA as the Ca2+ buffer (see Materials and methods). In panels B, D, and F, values are mean ± S.E.M. *p<0.05 by one-way ANOVA followed by unpaired t-test between the indicated variants. **: p<0.01 by unpaired t-test, ***: p<0.001 by unpaired t-test.
-
Figure 2—source data 1
Double mutants of L138 with neighboring TM1 residues reveals an interaction with T92.
- https://cdn.elifesciences.org/articles/82281/elife-82281-fig2-data1-v2.docx
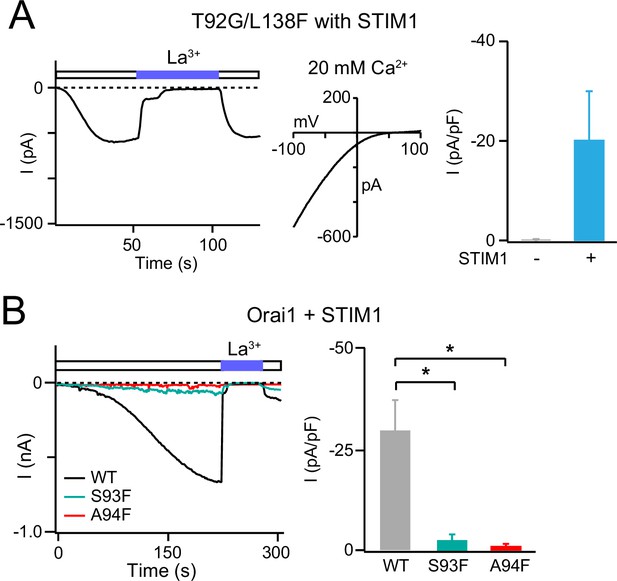
S93F and A94F mutations impede channel activation by STIM1.
(A) The T92G/L138F Orai1 double mutant can be gated and activated by STIM1. The left panel shows currents from the double mutant in the presence or absence of STIM1 co-expression, the right panel an example trace of T92G/L138F Orai1 currents in the presence of STIM1. Orai1 current is activated gradually following store depletion and shows the classic properties of Orai1 channels. N=3 cells. (B) Average current densities of S93F and A94F Orai1 channels expressed with STIM1. S93F and A94F Orai1 are non-functional even when co-expressed with STIM1. N=4 cells. Values are mean ± S.E.M. *: p<0.05 by unpaired t-test.
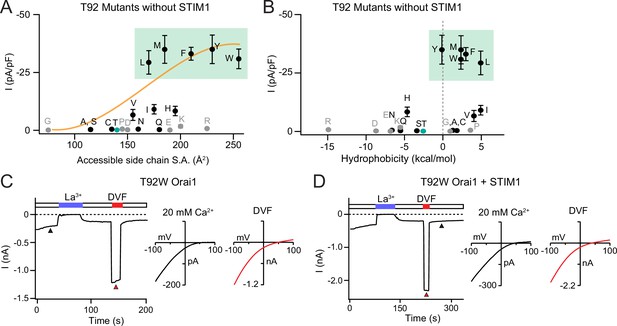
Large amino acid substitutions at T92 cause constitutive Orai1 activation.
(A–B) The current densities of T92 mutants in the absence of STIM1 plotted against side chain size and hydrophobicity, showing that bulky substitutions at T92 cause GOF channels. Plots show peak Orai1 currents recorded at –100 mV in HEK293 cells expressing the indicated Orai1 mutants. N=4–8 cells. Values are mean ± S.E.M. The green shaded areas denote residues that evoke GOF phenotypes. Grey dots denote amino acids (charged or Pro/Gly) which have the potential to disrupt the helical structure of TM2. (C) Example time course of T92W Orai1 current following whole-cell break-in at t=0 s in a cell expressing T92W Orai1 alone. The I-V graphs in 20 mM Ca2+ and DVF solutions are shown the right. T92W Orai1 alone shows inward rectifying I-V curves with positive reversal potentials, similar to STIM1-activated WT Orai1 channels. (D) Example time course and I-V plots of T92W Orai1 with STIM1 co-expression (Orai1:STIM1=1:5 cDNA transfection ratio). STIM1 does not significantly boost the current amplitude of GOF T92W Orai1 channels, suggesting that this mutant is nearly fully active at baseline. The intracellular solution used was the standard internal solution with 8 mM BAPTA as the Ca2+ buffer (see Materials and methods).
-
Figure 3—source data 1
Orai1 channel activity is correlated with side chain size at T92.
- https://cdn.elifesciences.org/articles/82281/elife-82281-fig3-data1-v2.docx
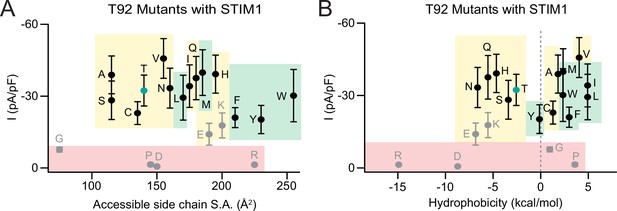
Analysis of T92 Orai1 mutant currents in the presence of STIM1.
(A–B) The current densities of T92 mutants with STIM1 co-expression plotted against size-chain size and hydrophobicity. Green, yellow, and red shading highlights GOF mutants, store-operated, and LOF mutants, respectively. Grey dots denote amino acids (charged or Pro/Gly) which have the potential to disrupt the helical structure of TM2. N=4–8 cells for each point. Values are mean ± S.E.M. N=5–8 cells. Values are mean ± S.E.M. ***: p<0.001 by unpaired t-test.
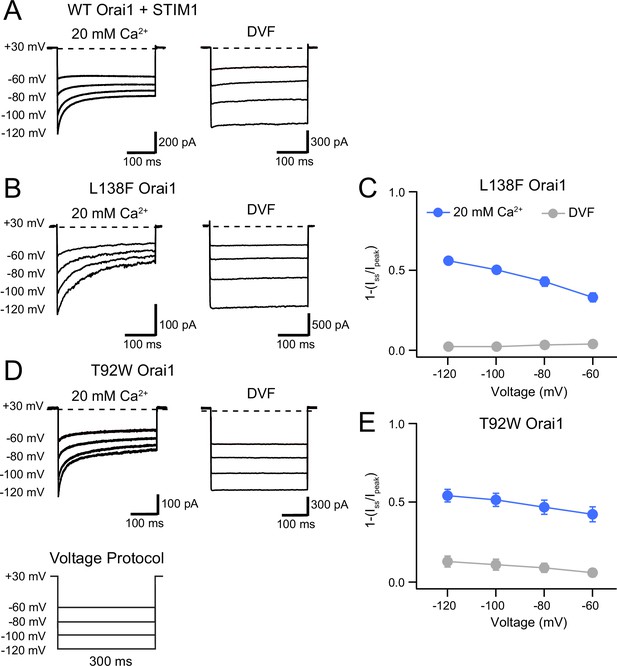
L138F and T92W Orai1 mutants show CDI.
(A) Currents in response to hyperpolarizing voltage steps in STIM1-gated WT Orai1 channels in the presence of 10 mM EGTA in the internal solution. Hyperpolarizing voltage steps from –120 to –60 mV lasting 300ms were applied from a holding potential of + 30 mV. Replacing the extracellular 20 mM Ca2+ Ringer’s solution with a DVF solution eliminates CDI, consistent with the known calcium dependence of CDI (Zweifach and Lewis, 1995). (B) Representative traces showing CDI of L138F Orai1 currents in a cell expressing L138F Orai1 alone. The intracellular solution contained 8 mM BAPTA. (C) Quantification of extent of inactivation (1−Iss/Ipeak) of L138F GOF mutant channel without STIM1 with 8 mM BAPTA internal solution. (D) Representative traces of CDI of T92W Orai1 without STIM1 with an 8 mM BAPTA internal solution. (E) Quantification of extent of inactivation (1−Iss/Ipeak) of T92W Orai1 in the absence of STIM1. In both mutants, inactivation seen in 20 mM Ca2+ is abolished in the presence of divalent-free (DVF) solution indicating that the inactivation requires conducting Ca2+ ions. N=5–17 cells. Values are mean ± S.E.M.
-
Figure 4—source data 1
Analysis of STIM1-independent inactivation in L138 and T92 mutants.
- https://cdn.elifesciences.org/articles/82281/elife-82281-fig4-data1-v2.docx
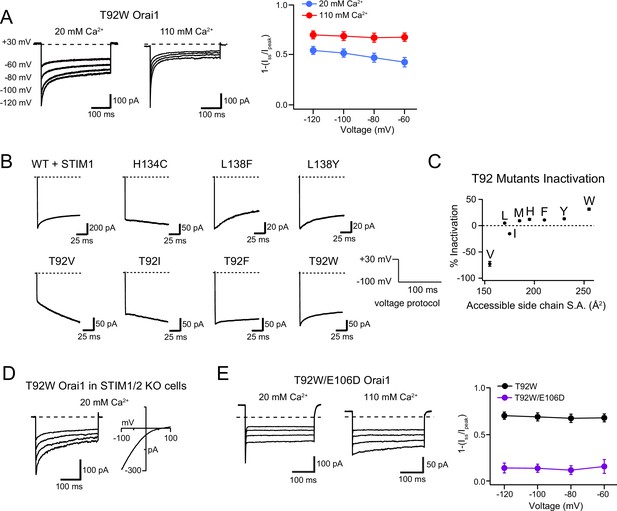
Analysis of T92 mutant CDI.
(A) Inactivation of the T92W Orai1 mutant is enhanced by raising extracellular Ca2+ to 110 mM. The left panels show T92W Orai1 currents in response to hyperpolarizing voltage steps from –120 to –60 mV from a holding potential of +30 mV. The right graph summarizes the extent of CDI of T92W Orai1 in 20 and 110 mM external solutions. (B) Example traces showing inactivation seen in STIM1-gated WT and L138 or T92 mutant channels during a 100ms hyperpolarizing step from +30 mV to -100 mV. GOF mutants such as H134C typically do not inactivate without STIM1, but L138F and L138Y mutants show inactivation during the 100ms hyperpolarizing step with kinetics comparable to CDI of WT Orai1 channels. (C) Inactivation of T92 mutants is positively correlated with the side-chain surface area (S.A.) at this position. T92V shows slight potentiation similar other previously described GOF mutants, whereas T92W inactivates ~30% over the 100ms hyperpolarization step to –100 mV with kinetics similar to STIM1-gated channels. (D) T92W Orai1 is constitutively active and displays inactivation in STIM1/2 double knock-out HEK cells, indicating that the activation and inactivation of this mutant is not dependent on STIM. (E) As previously shown for the E106D Orai1 single mutant gated by STIM1 (Yamashita et al., 2007), T92W/E106D Orai1 shows Ca2+-dependent block of Na+ current in 20 mM external Ca2+ and minimal inactivation in 110 mM Ca2+ solution. Inactivation of the T92W mutant in 110 mM Ca2+ solution with and without E106D. N=5–17 cells. Values are mean ± S.E.M. The intracellular solution used in all experiments here was the standard internal solution with 8 mM BAPTA as the Ca2+ buffer (see Materials and methods).
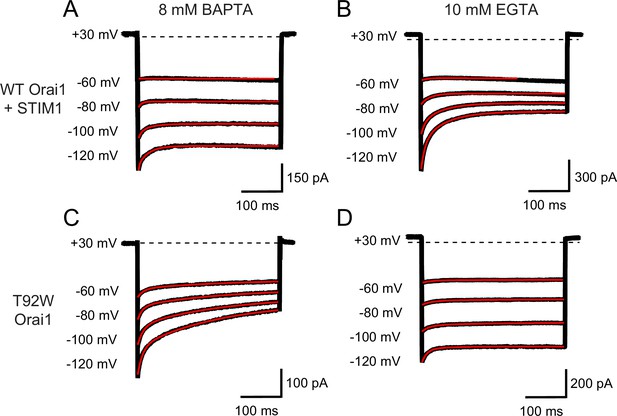
Kinetics of CDI in WT and T92W Orai1 channels.
(A–B) Examples of double-exponential fits of WT Orai1 voltage family traces. In cells with 10 mM EGTA as the internal solution, τfast = 10 ± 1 ms and τslow = 60 ± 8 ms at –100 mV (n=10 cells). (C–D) Examples of double-exponential fits of T92W Orai1 voltage family traces. In cells with 8 mM BAPTA as the internal solution, the inactivation time constants for T92W are: τfast = 11 ± 1 ms and τslow = 134 ± 16 ms (n=10 cells).
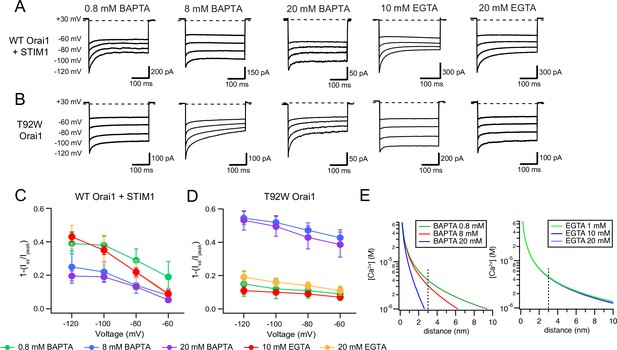
CDI of T92W Orai1 shows enhanced Ca2+ sensitivity compared to WT Orai1.
(A–B) Representative inactivation traces of WT Orai1 +STIM1 and constitutively active T92W Orai1 mutant channels during voltage steps with either a slow (EGTA) or fast (BAPTA) Ca2+ chelator in the internal solution. (A) In cells expressing WT Orai1, raising the intracellular BAPTA concentration from 0.8 to 8 or 20 mM diminishes CDI. Further, replacing intracellular BAPTA with EGTA at any concentration strongly enhances CDI. (B) However, in T92W Orai1, increasing the BAPTA concentration from 0.8 to 8 or 20 mM enhances CDI, and replacing BAPTA with EGTA strongly diminishes CDI. (C–D) The extent of inactivation (1−Iss/Ipeak) plotted against membrane voltage in the indicated buffer solutions. The extracellular Ringer’s solution contained 20 mM Ca2+ in all cases. N=4–17 cells. Values are mean ± S.E.M. Note that the inactivation quantification plot for T92W in 8 mM BAPTA is also shown in Figure 4E. (E) [Ca2+]i profiles in the presence of different concentrations of EGTA or BAPTA. [Ca2+] was estimated from equation 1 (see Results). The unitary Ca2+ current amplitude was assumed to be 5 fA. At distances beyond 2 nm, [Ca2+]i is significantly buffered by BAPTA but not EGTA.
-
Figure 5—source data 1
Dependence of T92W inactivation on internal local Ca2+ concentration compared to WT Orai1 channels.
- https://cdn.elifesciences.org/articles/82281/elife-82281-fig5-data1-v2.docx
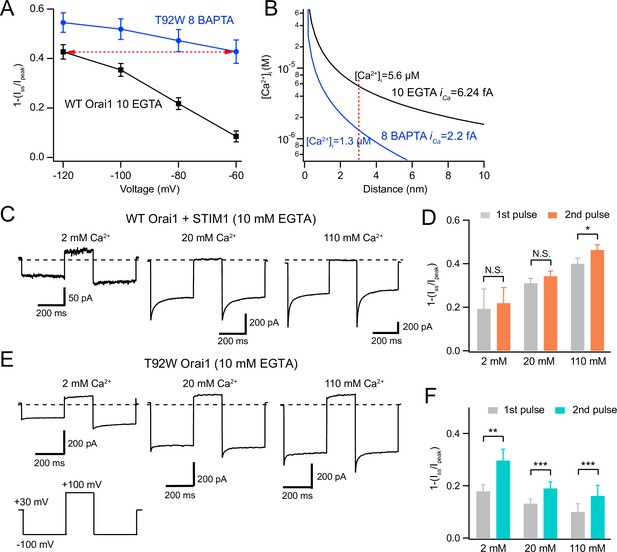
Depolarizing the cell promotes recovery from CDI in T92W Orai1 channels.
(A) Comparison of extent of CDI in constitutively active T92W Orai1 (in 8 mM internal BAPTA) and WT Orai1 +STIM1 currents (in 10 mM internal EGTA). The extent of CDI at –60 mV in T92W is identical to CDI in WT Orai1 channels at –120 mV (dashed red arrow). The standard external solution contained 20 mM Ca2+ (see Methods). (B) [Ca2+]i profiles in the two conditions that elicit similar levels of CDI in the experimental conditions in A. The unitary Ca2+ current at each potential was estimated by scaling the estimated iCa at –100 mV with the ratio of the peak whole-cell current at the different voltages (see Methods). The profiles indicate that although CDI is similar, the [Ca2+]i mediating CDI in T92W Orai1 at –60 mV is substantively lower than the [Ca2+]i in WT Orai1 at –120 mV. (C–F) Analysis of recovery from inactivation in response to termination of Ca2+ influx with depolarizing voltage steps. (C) After a –100 mV hyperpolarizing voltage step to induce CDI, the cell was depolarized to +100 mV to abruptly terminate Ca2+ influx and lower submembrane [Ca2+]i. Following the recovery interval at +100 mV (200 ms), a second pulse to –100 mV was applied to evoke CRAC current. In WT Orai1 channels, the second pulse evokes Orai1 current with similar amplitude and inactivation as the first pulse. Holding potential is +30 mV. (E) Recovery from inactivation in T92W Orai1. Following a recovery step to +100 mV to terminate Ca2+ influx, a second hyperpolarizing pulse to –100 mV reveals significantly larger peak current and re-appearance of CDI indicating that abruptly terminating Ca2+ influx partly restores inactivation of T92W Orai1 channels in 10 mM EGTA. (D and F) The extent of inactivation (1 − Iss/Ipeak) in the first and second pulses at different extracellular Ca2+ concentrations. For T92W channels, CDI was enhanced by pre-pulse with +100 mV in 2 mM, 20 mM, and 110 mM external Ca2+ solutions. N=6–8 cells. Values are mean ± S.E.M. *:p<0.05, **p<0.01, ***: p<0.001 by paired t-test.
-
Figure 6—source data 1
Paired pulse experiments reveals recovery of T92W inactivation through depolarizing steps.
- https://cdn.elifesciences.org/articles/82281/elife-82281-fig6-data1-v2.docx
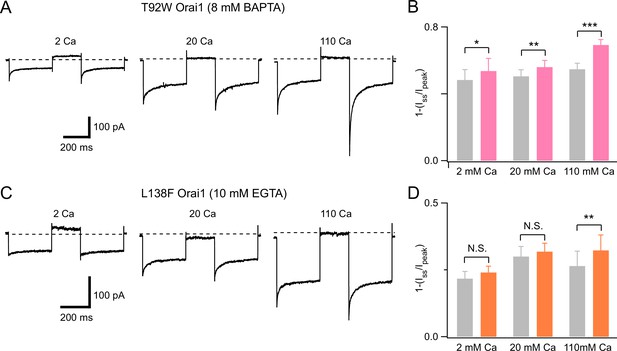
Recovery of inactivation of T92W and L138F Orai1 channels is promoted by depolarizing steps to +100 mV.
(A–B) T92W Orai1 CDI in paired pulses separated by a recovery step to +100 mV to reduce [Ca2+]i and promote recovery from CDI. The second pulse shows greater CDI relative to the first pulse indicating that abruptly terminating Ca2+ influx enhances channel recovery from inactivated states and manifests greater CDI during the second hyperpolarizing step. Intracellular chelator: 8 mM BAPTA. N=4–6 cells. (C–D) Recovery from inactivation of L138F Orai1. Recovery was examined using the same paired-pulse protocol in the presence of 10 mM intracellular EGTA. L138F recovery pattern resembles the pattern seen in WT Orai1 channels likely due to minimal accumulation of CDI at the holding potential due to the weak activity of the mutant channels. N=5–6 cells. Values are mean ± S.E.M. *:p<0.05, **p<0.01, ***: p<0.001 by paired t-test.
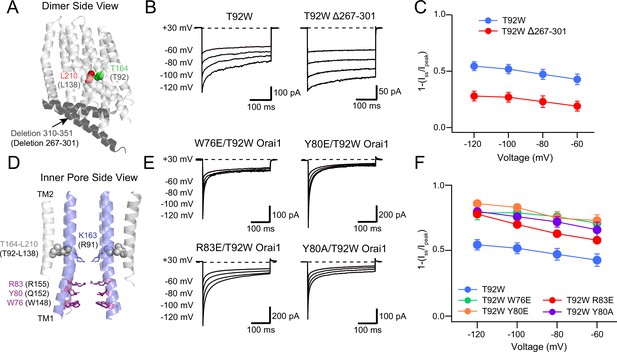
The Orai1 C-terminus contributes to T92W Orai1 CDI.
(A) Side view of dOrai dimer (PDB ID: 4HKR; hOrai1 numbering in parentheses) showing location of T92 and L138 residues relative to the deleted C-terminal residues 267–301. The Orai1 C-terminus is shown in dark grey. (B–C) Deletion of the Orai1 C-terminus residues attenuates inactivation of T92W Orai1. Example traces showing CDI of T92W Orai1 and T92W Δ267–301 Orai1 mutant (B) The extent of inactivation is summarized in panel C. (D) Location of N-terminal residues (W76, Y80 and R83) previously implicated in regulating CDI in Orai1 channels. (E) Mutations of W76, Y80 and R83 in T92W Orai1 all strongly accelerated CDI compared to T92W Orai1 alone. (F) The extent of CDI plotted against membrane voltage in the indicated mutants. The inactivation quantification plot for T92W in C and F is the same as the data for this mutant in Figure 4E. N=4–17 cells. Values are mean ± S.E.M. The intracellular solution used was the standard internal solution with 8 mM BAPTA as the Ca2+ buffer and the standard external solution contained 20 mM Ca2+ (see Materials and methods).
-
Figure 7—source data 1
Effects of N- and C-terminal mutations on T92W CDI.
- https://cdn.elifesciences.org/articles/82281/elife-82281-fig7-data1-v2.docx
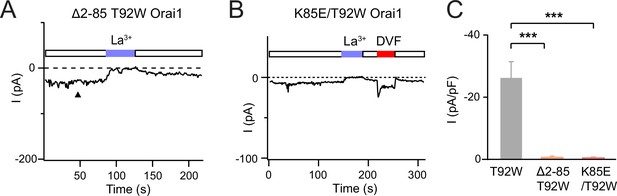
Deletion of the Orai1 N-terminus or introduction of a K85E mutation abrogates the constitutive T92W Orai1 current.
(A–B) Example traces of Δ2–85 T92W and K85E/T92W Orai1 mutants. The current density of these mutants is summarized in panel C. N=5–8 cells. Values are mean ± S.E.M. ***: p<0.001 by paired t-test.
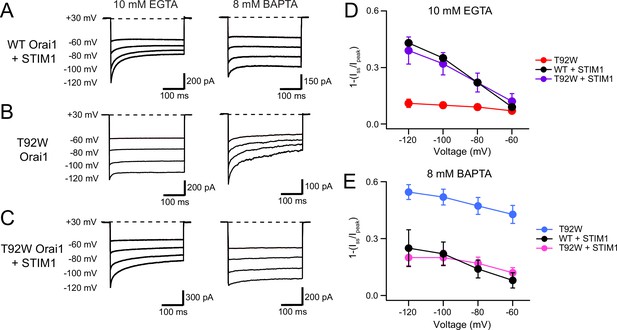
STIM1 modulates the Ca2+ sensitivity of T92W Orai1 CDI.
(A–B) Representative traces of CDI in WT Orai1 +STIM1 (A) and T92W Orai1 (B) currents in the presence of EGTA (10 mM) or BAPTA (8 mM). (C) The addition of STIM1 normalizes the aberrant intracellular buffer dependence of T92W Orai1 CDI. In the presence of STIM1, CDI of T92 Orai1 is strongly enhanced in 10 mM EGTA compared to T92W currents in the absence of STIM1. Conversely, CDI in STIM1-expressing T92W Orai1 cells in 8 mM BAPTA is reduced relative to cells expressing T92W Orai1 alone (compare right traces in T92W in panels B and C), analogous to the behavior seen in WT Orai1 (A). (D–E) Summary plots showing the extent of CDI in the indicated conditions. The inactivation quantification plots for WT and T92W Orai1 are also shown in Figure 5C–D. All recordings were conducted in the standard external solution containing 20 mM Ca2+. N=4–17 cells. Values are mean ± S.E.M.
-
Figure 8—source data 1
STIM1 restores the Ca2+ sensitivity of T92W CDI to that of WT Orai1.
- https://cdn.elifesciences.org/articles/82281/elife-82281-fig8-data1-v2.docx
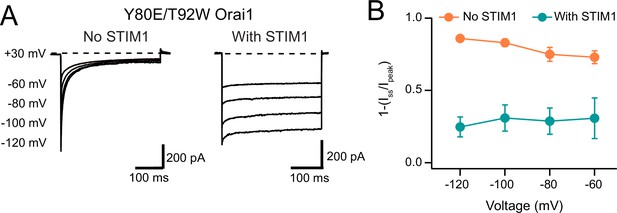
STIM1 modulates CDI of Y80E/T92W Orai1.
(A) Example traces of Y80E/T92W voltage family steps without and with STIM1 co-expression. (B) Summary data of Y80E/T92W Orai1 inactivation modulation by STIM1. The example trace and quantification shown without STIM1 is the same data as in Figure 7E–F. N=4 cells. Values are mean ± S.E.M.
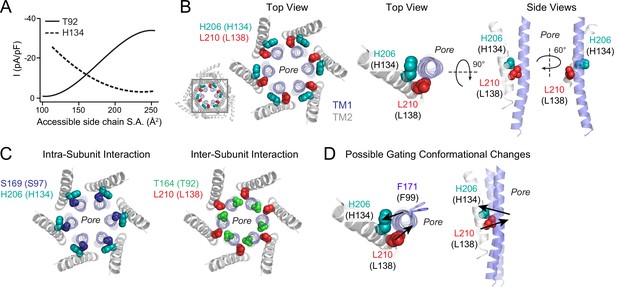
Schematic of the ‘brace’ formed by the H134 and L138 residues around TM1.
(A) Polynomial fits of T92 and H134 mutant channel activity plotted against side chain surface area shows opposite dependence of these two positions on size. For both fits, charged residues (D/E/K/R) and G/P were excluded from this analysis because of their propensity to break α-helical secondary structure. (B) Left: Top view of the dOrai hexameric channel with the relative positions at the TM1-TM2/3 ring interface with H134 (teal) and L138 (red) shown. Middle and right: Top and side views of residues H134 (teal spheres) and L138 (red spheres) at the TM1 (blue) and TM2 (grey) interface. The two amino acids are on either side of the pore helix, approximately one turn apart. One TM2 and TM1 helix from each subunit are displayed for simplicity. (C) Intra-subunit interaction of S97 (navy) with H134 (teal) and inter-subunit interaction of T92 (green) with L138 (red) are shown to highlight the different interfaces of these pairs of interactions. (D) Proposed gating conformational change of L138 and H134 associated with channel opening. H134 and L138 are positioned one helical turn away from each other on opposite sides of the TM1 pore helix. Amino acid substitutions at L138 that cause TM1 to be pushed inwards, or H134 to be drawn outwards, cause channel activation via TM1 pore helix twisting and pore dilation to open the channel gate.

Recovery from CDI of T92W Orai1 is not improved by prolonging the interpulse interval.
(A) The traces show inactivation in response to -100 mV pulses separated by a 3 s depolarizing step to +30 mV to promote recovery from CDI. Extracellular ca2+ was 20 mM and the intracellular solution contained 10 mM EGTA. (B) Recovery time course of CDI of T92W Orai1 channels. The interpulse interval was varied from 40 ms to 200 ms. The recovery time course is shown on the right plot. Recovery reaches steady-state by 200 ms.

Intracellular [Ca2+] profiles from a point source of Ca2+ entry.
The left plots show [Ca2+] profiles in varying concentrations of EGTA and the right profiles in BAPTA. Whereas local Ca2+ is largely unaffected by variations in [EGTA], they are profoundly reduced by increasing [BAPTA] from 0.8 mM to 8 mM or more. The dotted line shows the estimated distance of the putative Ca2+ binding site for CDI (Zweifach and Lewis, 1995. JGP).

Intracellular [Ca2+] in HEK293 cells expressing T92W Orai1, L138F Orai1, WT Orai1 + STIM1, or WT Orai1 alone.
Intracellular [Ca2+] was measured using fura-2. Stores were depleted as indicated using thapsigargin and extracellular Ca2+ added back to assess SOCE.
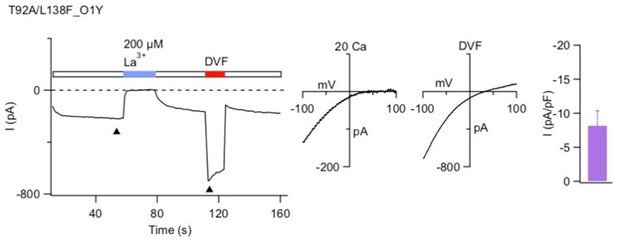
Introduction of an Ala residue at T92 causes constitutive activation of T92A/L138F Orai1.
T92A/L138F Orai1 currents were measured in the absence of STIM1 using standard methods. The left plot shows the peak current at -100 mV plotted over time and the right graph shows the current-voltage relationship of the ca2+ and monovalent currents as indicated.
Tables
Reagent type (species) or resource | Designation | Source or reference | Identifiers | Additional information |
---|---|---|---|---|
Cell line (Homo-sapiens) | HEK293-H | Thermo Fisher Scientific | 11631017 | RRID:CVCL_6643 |
Commercial assay or kit | QuikChange II XL Site-Directed Mutagenesis Kit | Agilent | 200522 | |
Transfected construct (human) | Orai1-YFP | Clontech | Navarro-Borelly et al., 2008 | |
Transfected construct (human) | mCherry-STIM1 | Richard Lewis (Stanford) | ||
Transfected construct (human) | CFP-CAD | Richard Lewis (Stanford) | ||
Chemical compound, drug | Lipofectamine 2000 | Thermo Fisher Scientific | 11668019 | |
Chemical compound, drug | EGTA | Sigma Aldrich | E3889 | |
Chemical compound, drug | BAPTA | Thermo Fisher Scientific | B1212 | |
Sequence-based reagent | mutagenesis primers for Orai1 L138A | IDT | This paper | gcaggtgctgatcatggccgcaaacaggtgcaca tgtgcacctgtttgcggccatgatcagcacctgc |
Sequence-based reagent | mutagenesis primers for Orai1 L138C | IDT | Yeung et al., 2018 | tgcaggtgctgatcatgcacgcaaacaggtgcacag ctgtgcacctgtttgcgtgcatgatcagcacctgca |
Sequence-based reagent | mutagenesis primers for Orai1 L138D | IDT | This paper | gcaggtgctgatcatgtccgcaaacaggtgcaca tgtgcacctgtttgcggacatgatcagcacctgc |
Sequence-based reagent | mutagenesis primers for Orai1 L138E | IDT | This paper | gatgcaggtgctgatcatctccgcaaacaggtgcacagc gctgtgcacctgtttgcggagatgatcagcacctgcatc |
Sequence-based reagent | mutagenesis primers for Orai1 L138F | IDT | This paper | caggtgctgatcatgaacgcaaacaggtgcaca tgtgcacctgtttgcgttcatgatcagcacctg |
Sequence-based reagent | mutagenesis primers for Orai1 L138G | IDT | This paper | gcaggtgctgatcatgcccgcaaacaggtgcaca tgtgcacctgtttgcgggcatgatcagcacctgc |
Sequence-based reagent | mutagenesis primers for Orai1 L138H | IDT | This paper | caggtgctgatcatgtgcgcaaacaggtgca tgcacctgtttgcgcacatgatcagcacctg |
Sequence-based reagent | mutagenesis primers for Orai1 L138I | IDT | This paper | caggtgctgatcatgatcgcaaacaggtgcaca tgtgcacctgtttgcgatcatgatcagcacctg |
Sequence-based reagent | mutagenesis primers for Orai1 L138K | IDT | This paper | gatgcaggtgctgatcatcttcgcaaacaggtgcacagc gctgtgcacctgtttgcgaagatgatcagcacctgcatc |
Sequence-based reagent | mutagenesis primers for Orai1 L138M | IDT | This paper | atgcaggtgctgatcatcatcgcaaacaggtgcacag ctgtgcacctgtttgcgatgatgatcagcacctgcat |
Sequence-based reagent | mutagenesis primers for Orai1 L138N | IDT | This paper | tgcaggtgctgatcatgttcgcaaacaggtgcacag ctgtgcacctgtttgcgaacatgatcagcacctgca |
Sequence-based reagent | mutagenesis primers for Orai1 L138P | IDT | This paper | caggtgctgatcatgggcgcaaacaggtgca tgcacctgtttgcgcccatgatcagcacctg |
Sequence-based reagent | mutagenesis primers for Orai1 L138Q | IDT | This paper | tgcaggtgctgatcatctgcgcaaacaggtgcac gtgcacctgtttgcgcagatgatcagcacctgca |
Sequence-based reagent | mutagenesis primers for Orai1 L138R | IDT | This paper | caggtgctgatcatgcgcgcaaacaggtgca tgcacctgtttgcgcgcatgatcagcacctg |
Sequence-based reagent | mutagenesis primers for Orai1 L138S | IDT | This paper | tgcaggtgctgatcatgctcgcaaacaggtgcacag ctgtgcacctgtttgcgagcatgatcagcacctgca |
Sequence-based reagent | mutagenesis primers for Orai1 L138T | IDT | This paper | tgcaggtgctgatcatggtcgcaaacaggtgcacag ctgtgcacctgtttgcgaccatgatcagcacctgca |
Sequence-based reagent | mutagenesis primers for Orai1 L138V | IDT | This paper | aggtgctgatcatgaccgcaaacaggtgcac gtgcacctgtttgcggtcatgatcagcacct |
Sequence-based reagent | mutagenesis primers for Orai1 L138W | IDT | This paper | gatgcaggtgctgatcatccacgcaaacaggtgcacagc gctgtgcacctgtttgcgtggatgatcagcacctgcatc |
Sequence-based reagent | mutagenesis primers for Orai1 L138Y | IDT | This paper | ggatgcaggtgctgatcatatacgcaaacaggtgcacagcc ggctgtgcacctgtttgcgtatatgatcagcacctgcatcc |
Sequence-based reagent | mutagenesis primers for Orai1 T92A | IDT | This paper | cagagccgaggcccggctggagg cctccagccgggcctcggctctg |
Sequence-based reagent | mutagenesis primers for Orai1 T92C | IDT | Yeung et al., 2018 | gagcagagccgagcaccggctggaggct agcctccagccggtgctcggctctgctc |
Sequence-based reagent | mutagenesis primers for Orai1 T92D | IDT | This paper | gagcagagccgagtcccggctggaggct agcctccagccgggactcggctctgctc |
Sequence-based reagent | mutagenesis primers for Orai1 T92E | IDT | This paper | ggagagcagagccgactcccggctggaggcttt aaagcctccagccgggagtcggctctgctctcc |
Sequence-based reagent | mutagenesis primers for Orai1 T92F | IDT | This paper | gagcagagccgagaaccggctggaggct agcctccagccggttctcggctctgctc |
Sequence-based reagent | mutagenesis primers for Orai1 T92G | IDT | This paper | gagcagagccgagccccggctggaggct agcctccagccggggctcggctctgctc |
Sequence-based reagent | mutagenesis primers for Orai1 T92H | IDT | This paper | gagcagagccgagtgccggctggaggct agcctccagccggcactcggctctgctc |
Sequence-based reagent | mutagenesis primers for Orai1 T92I | IDT | This paper | agcagagccgagatccggctggagg cctccagccggatctcggctctgct |
Sequence-based reagent | mutagenesis primers for Orai1 T92K | IDT | This paper | agcagagccgacttccggctggaggctttaagc gcttaaagcctccagccggaagtcggctctgct |
Sequence-based reagent | mutagenesis primers for Orai1 T92L | IDT | This paper | cggagagcagagccgatagccggctggaggcttta taaagcctccagccggctatcggctctgctctccg |
Sequence-based reagent | mutagenesis primers for Orai1 T92M | IDT | This paper | agcagagccgacatccggctggaggctttaagc gcttaaagcctccagccggatgtcggctctgct |
Sequence-based reagent | mutagenesis primers for Orai1 T92N | IDT | This paper | agcagagccgagttccggctggagg cctccagccggaactcggctctgct |
Sequence-based reagent | mutagenesis primers for Orai1 T92P | IDT | This paper | cagagccgagggccggctggagg cctccagccggccctcggctctg |
Sequence-based reagent | mutagenesis primers for Orai1 T92Q | IDT | This paper | ggagagcagagccgactgccggctggaggcttt aaagcctccagccggcagtcggctctgctctcc |
Sequence-based reagent | mutagenesis primers for Orai1 T92R | IDT | This paper | gcagagccgacctccggctggaggctttaa ttaaagcctccagccggaggtcggctctgc |
Sequence-based reagent | mutagenesis primers for Orai1 T92S | IDT | This paper | gcagagccgagctccggctggag ctccagccggagctcggctctgc |
Sequence-based reagent | mutagenesis primers for Orai1 T92V | IDT | This paper | gagcagagccgagacccggctggaggct agcctccagccgggtctcggctctgctc |
Sequence-based reagent | mutagenesis primers for Orai1 T92W | IDT | This paper | ggagagcagagccgaccaccggctggaggcttt aaagcctccagccggtggtcggctctgctctcc |
Sequence-based reagent | mutagenesis primers for Orai1 T92Y | IDT | This paper | cggagagcagagccgaataccggctggaggcttta taaagcctccagccggtattcggctctgctctccg |
Sequence-based reagent | mutagenesis primers for Orai1 K85E | IDT | McNally et al., 2013 | ggaggctttaagctcggcgcggctcaagt acttgagccgcgccgagcttaaagcctcc |
Sequence-based reagent | mutagenesis primers for Orai1 W76E | IDT | Mullins et al., 2016b | caagtagagcttgcgctcggacagcgcctgcatg catgcaggcgctgtccgagcgcaagctctacttg |
Sequence-based reagent | mutagenesis primers for Orai1 Y80A | IDT | Mullins et al., 2016b | ggcgcggctcaaggcgagcttgcgccag ctggcgcaagctcgccttgagccgcgcc |
Sequence-based reagent | mutagenesis primers for Orai1 Y80E | IDT | Mullins et al., 2016b | tggcgcggctcaactcgagcttgcgccag ctggcgcaagctcgagttgagccgcgcca |
Sequence-based reagent | mutagenesis primers for Orai1 R83E | IDT | Mullins et al., 2016b | ggaggctttaagcttggcctcgctcaagtagagcttgcg cgcaagctctacttgagcgaggccaagcttaaagcctcc |
Sequence-based reagent | mutagenesis primers for Orai1 del267-301 | IDT | Yamashita et al., 2007 | gcgtccagctgcacatccaccattgccac gtggcaatggtggatgtgcagctggacgc |
Sequence-based reagent | mutagenesis primers for Orai1 del267-301 | IDT | This paper | ggcgaccggtggatcggtcttatggctaac gttagccataagaccgatccaccggtcgcc |