Improved ANAP incorporation and VCF analysis reveal details of P2X7 current facilitation and a limited conformational interplay between ATP binding and the intracellular ballast domain
Figures
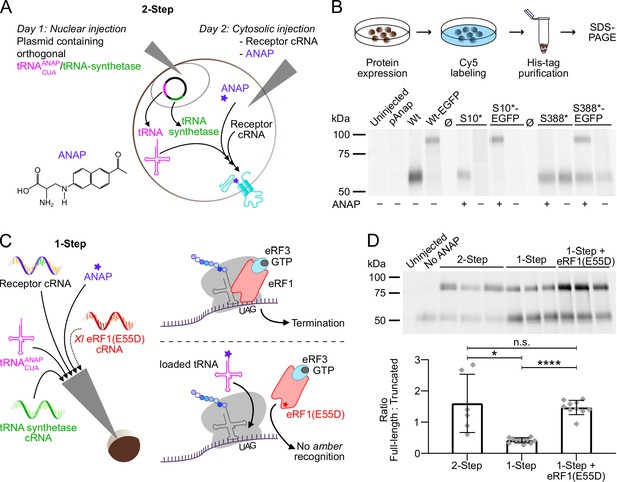
Optimization of fluorescent unnatural amino acid (fUAA) incorporation into Xenopus laevis oocyte-expressed P2X1 receptor.
(A) Molecular structure of L-3-(6-acetylnaphthalen-2-ylamino)–2-aminopropanoic acid (ANAP) and schematic representation of the 2-step injection method for site-specific ANAP incorporation using the amber stop-codon (UAG) and a plasmid containing the orthogonal tRNA/tRNA-synthetase pair. (B) Representative SDS-PAGE analysis of plasma membrane-expressed ANAP-labeled (S10* or S388*) rat P2X1Rs (46 kDa without glycosylation). A C-terminal EGFP-tag (27 kDa) was added as indicated to enable detection of premature termination at position 388. Oocytes were injected as shown in A and labeled with membrane impermeable Cy5-NHS ester. His-tagged P2X1Rs were extracted in 0.5% n-dodecyl-β-D-maltoside, purified via Ni2+-NTA agarose, and separated by SDS-PAGE (8%). Noninjected oocytes and oocytes injected only with the plasmid pANAP, P2X1 cRNA without the amber stop codon (Wt), or without ANAP (as indicated) served as controls. Note, that twice the amount of protein was loaded for P2X1(S10*). Ø indicates empty lanes. Two to three independent experiments were performed. (C) Representation of the 1-step injection method and all components required for UAA-labeling plus optional X. laevis eRF1(E55D) cRNA (left) and (right) scheme of protein translation termination by eRF1 (upper panel) and how overexpression of the mutated form of eRF1 favors amber-encoded fUAA incorporation by outcompeting endogenous eRF1 (lower panel). (D) Comparison of Cy5-labeled membrane-expressed full-length and truncated His-rP2X1-EGFP(388*) ratios upon expression by the 2-step and 1-step injection method with or without eRF1(E55D) co-expression. A representative SDS-PAGE gel (prepared as in B) and statistical analysis of data from 6 to 11 experiments including oocytes from 4 to 6 different X. laevis frogs per group are shown. Data are represented as mean ± S.D., and significance was determined by a two-tailed unpaired Welch’s t-test and is indicated as *p<0.05 and ****p<0.0001.
-
Figure 1—source data 1
Original gel, Figure 1B.
- https://cdn.elifesciences.org/articles/82479/elife-82479-fig1-data1-v2.zip
-
Figure 1—source data 2
Original gel, Figure 1D.
- https://cdn.elifesciences.org/articles/82479/elife-82479-fig1-data2-v2.zip
-
Figure 1—source data 3
Original gels for bar graph in Figure 1D: Gel2, Gel3, Gel4, and Gel5.
- https://cdn.elifesciences.org/articles/82479/elife-82479-fig1-data3-v2.zip
-
Figure 1—source data 4
Original gels for bar graph in Figure 1D: GelA, GelB, and GelC.
- https://cdn.elifesciences.org/articles/82479/elife-82479-fig1-data4-v2.zip
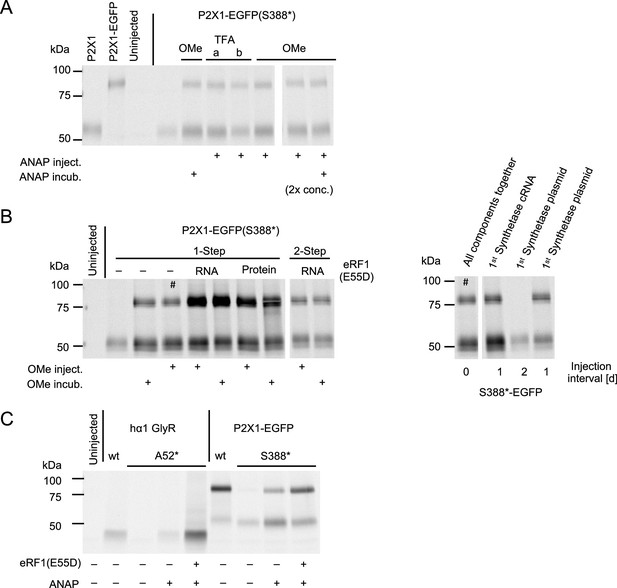
Variation of experimental conditions to optimize L-3-(6-acetylnaphthalen-2-ylamino)–2-aminopropanoic acid (ANAP) incorporation into oocyte-expressed ion channels.
(A) Comparison of ANAP-trifluoroacetic salt (TFA, a and b indicate two different batches) and membrane-permeable ANAP methyl ester (OMe) and application forms (injection and/or incubation in 2 mM solution). In the last two lanes, a twofold higher concentration (500 mM) was used for injection. Lanes shown in the same figure are from the same gel but rearranged for clarity. (B) Left: Comparison of ANAP-OMe application forms (injection and incubation as above) and effect of co-injected X. laevis eRF1(E55D) (as purified protein or as cRNA, as indicated) for the 1-step and the 2-step injection method. Right: Effect of different injection intervals. tRNA synthetase was expressed first, either from the pANAP plasmid (injected into the nucleus) or from the in vitro synthesized cRNA (injected into the cytoplasm) as indicated. All other components were then injected into the cytoplasm after the shown intervals. Note that the gel was rearranged for clearer presentation and that lanes marked with a hashtag are shown twice. (C) The optimized 1-step injection protocol with and without co-injection of eRF1(E55D) applied to hα1 GlyR(A52*) and P2X1(S388*)-EGFP.
-
Figure 1—figure supplement 1—source data 1
Original gel, Figure 1—figure supplement 1A.
- https://cdn.elifesciences.org/articles/82479/elife-82479-fig1-figsupp1-data1-v2.zip
-
Figure 1—figure supplement 1—source data 2
Original gel, Figure 1—figure supplement 1B.
- https://cdn.elifesciences.org/articles/82479/elife-82479-fig1-figsupp1-data2-v2.zip
-
Figure 1—figure supplement 1—source data 3
Original gel, Figure 1—figure supplement 1C.
- https://cdn.elifesciences.org/articles/82479/elife-82479-fig1-figsupp1-data3-v2.zip
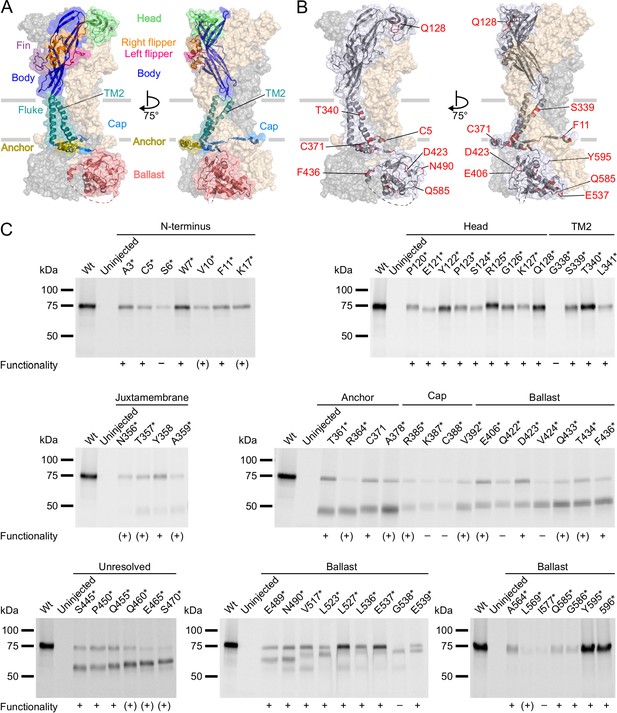
P2X7Rs containing L-3-(6-acetylnaphthalen-2-ylamino)–2-aminopropanoic acid (ANAP) at various positions within the extracellular, transmembrane, and cytoplasmic regions are expressed in the plasma membrane and functional.
(A, B) Surface representations of the rat P2X7 Cryo-EM structure in the open state (PDB ID: 6u9w). The different domains (A) and selected sites of ANAP substitutions (B) are indicated in one subunit while the two other subunits are shown in gray and wheat, respectively. (C) Evaluation of surface expression and functionality of P2X7Rs generated from constructs containing an amber stop codon in the indicated positions. X. laevis oocytes expressing the constructs were labeled with membrane-impermeant Cy5-NHS ester. His-tagged P2X7Rs were extracted in 0.5% n-dodecyl-β-D-maltoside, purified via Ni2+ NTA agarose, and analyzed by SDS-PAGE (8%). Symbols indicate current responses to 0.3 mM ATP as determined by two-electrode voltage clamp recordings in the voltage clamp fluorometry setup: +, functional and currents comparable to wt P2X7 after 2–4 days of expression; (+), functional and currents comparable to wt P2X7 after 5–7 days of expression; –, not functional or currents ≤0.5 μA and not reproducible after 4 days. Representative data from two to five independent biochemical experiments are shown.
-
Figure 2—source data 1
Original gel (1), Figure 2C (N-terminus).
- https://cdn.elifesciences.org/articles/82479/elife-82479-fig2-data1-v2.zip
-
Figure 2—source data 2
Original gel (2), Figure 2C (head domain and TM2).
- https://cdn.elifesciences.org/articles/82479/elife-82479-fig2-data2-v2.zip
-
Figure 2—source data 3
Original gel (3), Figure 2C (juxtamembrane).
- https://cdn.elifesciences.org/articles/82479/elife-82479-fig2-data3-v2.zip
-
Figure 2—source data 4
Original gel (4), Figure 2C (anchor, cap, and ballast domain).
- https://cdn.elifesciences.org/articles/82479/elife-82479-fig2-data4-v2.zip
-
Figure 2—source data 5
Original gel (5), Figure 2C (unresolved).
- https://cdn.elifesciences.org/articles/82479/elife-82479-fig2-data5-v2.zip
-
Figure 2—source data 6
Original gel (6), Figure 2C (ballast domain, part a).
- https://cdn.elifesciences.org/articles/82479/elife-82479-fig2-data6-v2.zip
-
Figure 2—source data 7
Original gel (7), Figure 2C (ballast domain, part b).
- https://cdn.elifesciences.org/articles/82479/elife-82479-fig2-data7-v2.zip
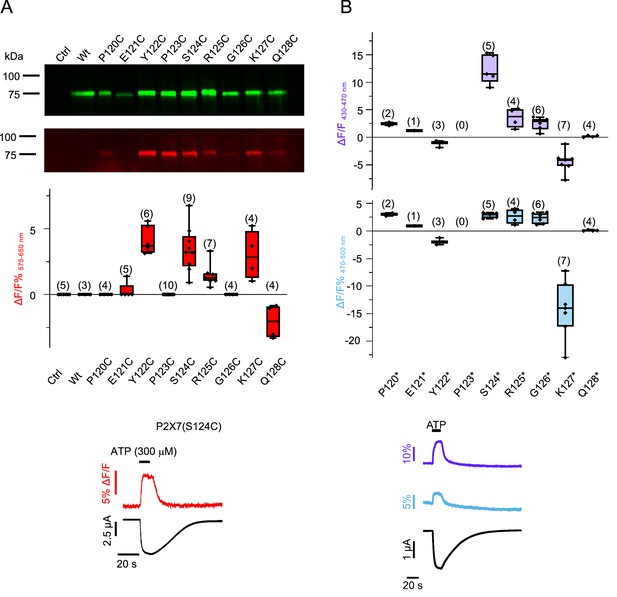
Comparative analysis of tetramethyl-rhodamine-maleimide (TMRM)-labeled and L-3-(6-acetylnaphthalen-2-ylamino)–2-aminopropanoic acid (ANAP)-labeled P2X7 head domain mutants.
(A) To estimate plasma membrane expression (upper gel) and accessibility of introduced cysteine residues (lower gel), intact oocytes expressing the indicated cysteine-substituted P2X7R mutants were either labeled with the membrane-impermeant amino-reactive Cy5-NHS ester or with TMRM. Purified His-tagged protein was visualized by fluorescence scanning. Below: Box plot summarizing results from TMRM-labeled oocytes expressing indicated P2X7R mutants with ΔF/F% representing the maximum fluorescence signal during a 15-s ATP application (300 μM). Numbers of recordings are given in brackets and a representative voltage clamp fluorometry (VCF)-recording (-60 mV) of a TMRM-labeled oocyte expressing P2X7(S124C) is shown. (B) Summary of fluorescence (violet and blue) changes of oocytes expressing P2X7Rs containing ANAP in the indicated positions within the head domain and a representative VCF-recording (at –30 mV) from an oocyte expressing P2X7 with ANAP in position 124 (P2X7(S124*)). Note that in case of mutants P120*, E121*, and P123* less than three recordings fulfilled the inclusion criteria described in Material and methods. Original recordings have also been deposited with Dryad and summarized and assigned in Table 1—source data 1.
-
Figure 2—figure supplement 1—source data 1
Original gel, Figure 2—figure supplement 1A.
- https://cdn.elifesciences.org/articles/82479/elife-82479-fig2-figsupp1-data1-v2.zip
-
Figure 2—figure supplement 1—source data 2
Summarized data for box plot in Figure 2—figure supplement 1A.
- https://cdn.elifesciences.org/articles/82479/elife-82479-fig2-figsupp1-data2-v2.zip
-
Figure 2—figure supplement 1—source data 3
Original recordings for box plot in Figure 2—figure supplement 1A and for representative VCF recordings in Figure 2—figure supplement 1A and B.
- https://cdn.elifesciences.org/articles/82479/elife-82479-fig2-figsupp1-data3-v2.zip
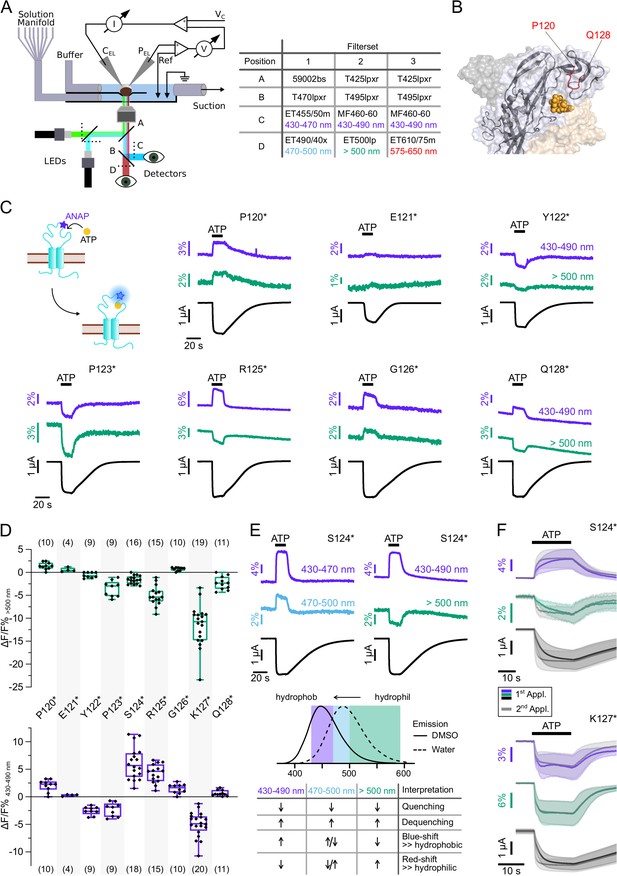
Characterization of ATP-induced fluorescence changes in the P2X7 head domain recorded at different wavelengths.
(A) Schematic of the voltage clamp fluorometry (VCF)-recording system and summary of filter/dichroic mirror configurations used to detect distinct spectral parts of L-3-(6-acetylnaphthalen-2-ylamino)–2-aminopropanoic acid (ANAP)-fluorescence (sets 1 and 2) and ANAP in combination with tetramethyl-rhodamine-maleimide (TMRM) or R-GECO1.2 (set 3). The corresponding positions A, B, C, and D are shown in the schematic. A second LED (green) was used for additional excitation of TMRM or R-GECO1.2. (B) Close-up of the P2X7 head domain in surface representation indicating the ANAP-substituted amino acid residues P120-Q128 (red). The three subunits are colored in gray, wheat, and light blue. (C) Principle of VCF and representative VCF recordings in response to 0.3 mM ATP (upon second application). Change of fluorescence intensity of a site-specifically introduced environment-sensitive fluorophore can be induced by ligand binding and/or conformational changes. (D) Box plots summarizing results from the indicated ANAP-labeled P2X7Rs at two different emission wavelengths with ΔF/F% representing the maximum fluorescence signal during a 15-s ATP application. Numbers of recordings are given in brackets. (E) Representative VCF recordings in response to 0.3 mM ATP of P2X7(S124*) at three different emission wavelengths and summary of most likely interpretations. Note that fluorescence changes are most likely resulting from multiple effects, and only the dominant effect is stated. Arrows indicate direction of fluorescent changes. (F) Overlay of VCF recordings upon first (colored) and second (gray) ATP applications (0.3 mM) at two different emission wavelengths for P2X7(S124*) (14 oocytes) and P2X7(K127*) (17 oocytes), respectively. Averaged VCF recordings are shown as lines, and standard deviations are plotted as envelopes. Baseline currents (15 s before ATP application) were adjusted for clarity. All recordings were performed in divalent-free buffer, and oocytes were clamped at –30 mV. Original recordings have also been deposited with Dryad and summarized and assigned in Table 1—source data 1.
-
Figure 3—source data 1
Original recordings, Figure 3C, E and F.
- https://cdn.elifesciences.org/articles/82479/elife-82479-fig3-data1-v2.zip
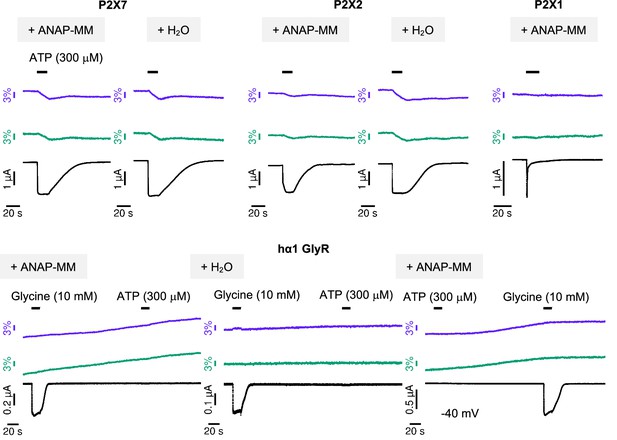
Control voltage clamp fluorometry (VCF) recordings from oocytes expressing different non-mutated ion channels.
Representative VCF-recordings of oocytes injected with cRNA encoding the indicated receptors plus either water (negative control) or L-3-(6-acetylnaphthalen-2-ylamino)–2-aminopropanoic acid (ANAP)-Master Mix (ANAP-MM, containing ANAP, tRNA, cRNA ecoding tRNA-syntethase, and cRNA encoding eRF1(E55D)). The holding potential was –30 mV, if not otherwise indicated.
-
Figure 3—figure supplement 1—source data 1
Original recordings, Figure 3—figure supplement 1.
- https://cdn.elifesciences.org/articles/82479/elife-82479-fig3-figsupp1-data1-v2.zip
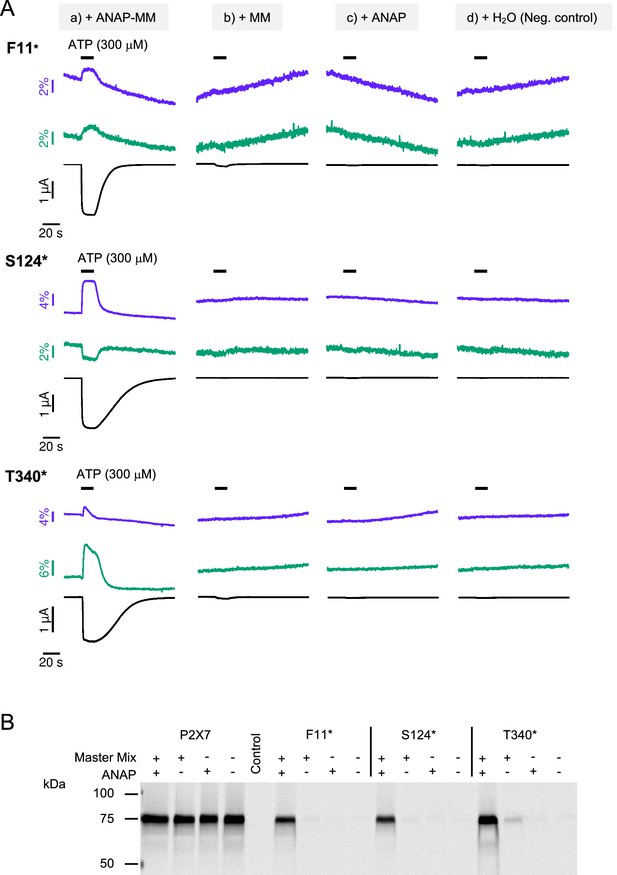
Control experiments to test the specificity of tRNA-loading and L-3-(6-acetylnaphthalen-2-ylamino)–2-aminopropanoic acid (ANAP) incorporation into P2X7.
(A,B) Representative voltage clamp fluorometry (VCF) recordings (A) and analysis of membrane expression (B) from oocytes that were injected with cRNA encoding non-mutated P2X7 or P2X7 containing an amber stop codon at the indicated positions together with (a) both, ANAP and a master mix containing tRNA-synthetase cRNA, tRNA, and eRF1(E55D) cRNA (positive control), (b) with the master mix only, (c) with ANAP only, or (d) with water as a negative control. ANAP emission was recorded at two different wavelengths (purple: 430–490 nm, green: >500 nm). VCF recordings showed clear ATP-evoked signals only for the oocytes injected with all essential components necessary for ANAP-incorporation and were in agreement with surface-expression analysis experiments. Although faint surface expression of full-length receptors was seen in the absence of ANAP (indicating limited read through), ATP-evoked fluorescence and current responses were negligible (no fluorescence change and current responses less than 10% of those from positive controls).
-
Figure 3—figure supplement 2—source data 1
Original recordings, Figure 3—figure supplement 2A.
- https://cdn.elifesciences.org/articles/82479/elife-82479-fig3-figsupp2-data1-v2.zip
-
Figure 3—figure supplement 2—source data 2
Original gel, Figure 3—figure supplement 2B.
- https://cdn.elifesciences.org/articles/82479/elife-82479-fig3-figsupp2-data2-v2.zip
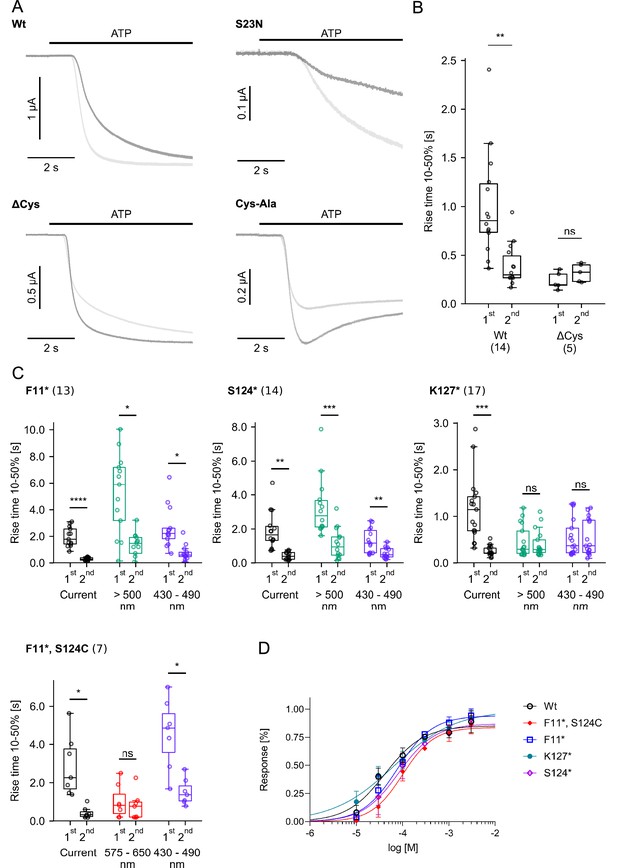
Deletion of the cysteine-rich region eliminates current facilitation, and F11* and S124* mutants track current facilitation.
(A) Overlay of representative current traces upon first (black) and second (gray) ATP applications (0.3 mM in 195 s interval) for wt P2X7, and the indicated mutations that were expected to prevent facilitation. Baseline currents (15 s before ATP application) were adjusted for clarity. (B) Box plot summarizing 10–50% rise times of the first and second current responses to ATP for wt and ΔCys P2X7. Note that the low expression of the S23N and Cys-Ala mutants prevented further analysis. (C) Box plots summarizing 10–50% rise times of the first and second current (black) and fluorescence (colored) responses at the indicated emission wavelengths for F11*, S124*, K127*, and the tetramethyl-rhodamine-maleimide-labeled double mutant (F11*, S124C). Significance was determined using the two-tailed paired Student’s t-test (*, p<0.05; **, p<0.005; ***, p<0.0005; ****, p<0.00005; ns, not significant). (D) Normalized dose-response curves for ATP at wt P2X7 and the indicated L-3-(6-acetylnaphthalen-2-ylamino)–2-aminopropanoic acid-containing receptors. Lines represent nonlinear curve fits of the Hill equation to the data. For EC50 values see Table 2. Error bars represent S.D. of 3–11 experiments. All recordings were performed in divalent-free buffer, and oocytes were clamped at –30 mV. Original recordings have also been deposited with Dryad and summarized and assigned in Table 1—source data 1.
-
Figure 3—figure supplement 3—source data 1
Summarized data, Figure 3—figure supplement 3A and B.
- https://cdn.elifesciences.org/articles/82479/elife-82479-fig3-figsupp3-data1-v2.zip
-
Figure 3—figure supplement 3—source data 2
Original recordings, Figure 3—figure supplement 3A and B.
- https://cdn.elifesciences.org/articles/82479/elife-82479-fig3-figsupp3-data2-v2.zip
-
Figure 3—figure supplement 3—source data 3
Original recordings, Figure 3—figure supplement 3C.
- https://cdn.elifesciences.org/articles/82479/elife-82479-fig3-figsupp3-data3-v2.zip
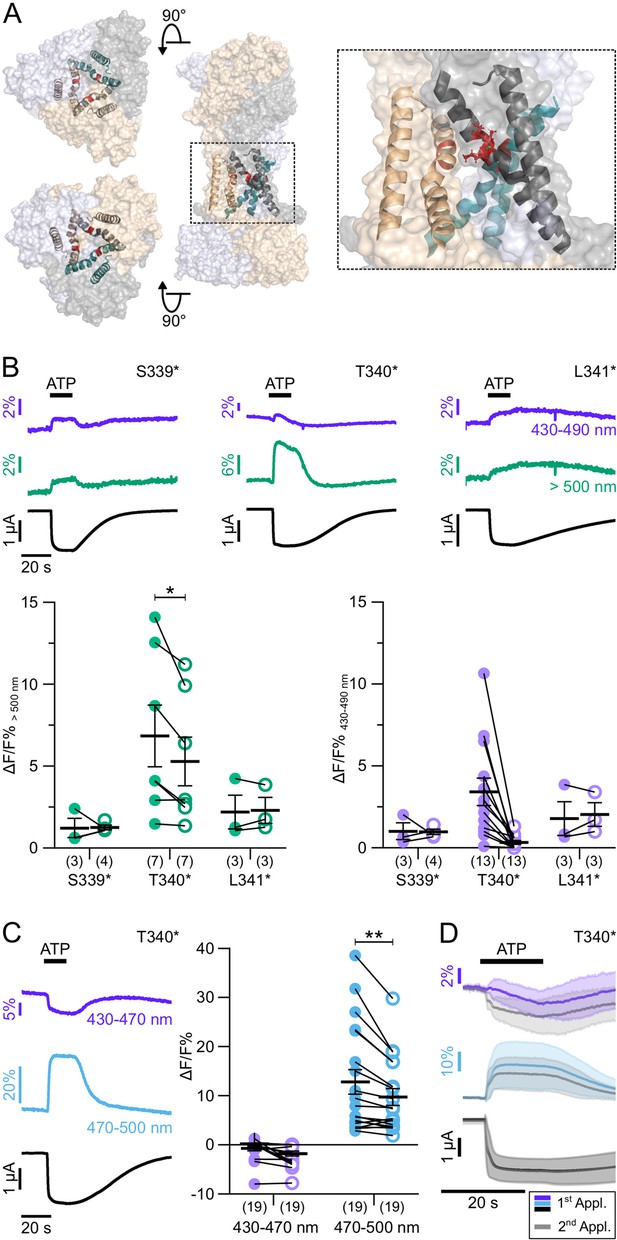
ATP-induced L-3-(6-acetylnaphthalen-2-ylamino)–2-aminopropanoic acid (ANAP) fluorescence changes in the P2X7 TM2 domain.
(A) Overview and close-up of the three P2X7 subunits (in wheat, gray, and purple) with the TM helices as cartoon representations (in wheat, gray, and green) and the ANAP-substituted residues S339, T340, and L341 (in red). (B) Representative voltage clamp fluorometry (VCF) recordings from the indicated mutants in response to 0.3 mM ATP (upon second application) and summary of results at two different emission wavelengths. Note that recordings from all constructs were compromised by high leak currents. Graphs compare maximal fluorescence signals during first (closed circles) and second (open circles) ATP applications (interval 195 s). Data are represented as mean ± S.E.M. Significance was determined using the two-tailed paired Student’s t-test (*, p<0.05; **, p<0.005). (C) Representative recordings and summary (performed as in B) from P2X7(T340*) with filter set 2. (D) Overlay of VCF recordings from P2X7(T340*) upon first (colored) and second (gray) ATP applications (0.3 mM) at two different emission wavelengths. Averaged VCF recordings from 11 oocytes are shown as lines, and standard deviations are plotted as envelopes. Baseline currents (15 s before ATP application) were adjusted for clarity. All recordings were performed in divalent-free buffer, and oocytes were clamped at –30 mV. Wavelengths passed by the used filter sets are indicated. Original recordings have also been deposited with Dryad and summarized and assigned in Table 1—source data 1.
-
Figure 4—source data 1
Original recordings, Figure 4B, C, and D.
- https://cdn.elifesciences.org/articles/82479/elife-82479-fig4-data1-v2.zip
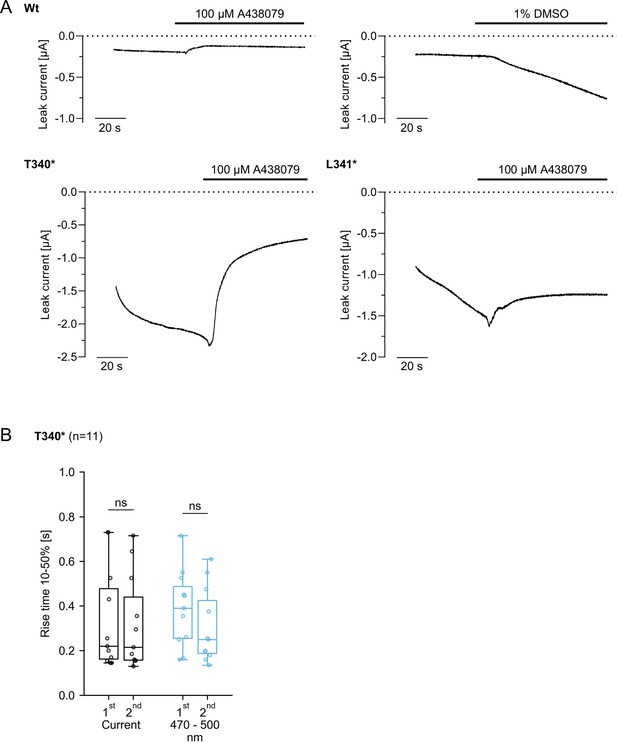
L-3-(6-acetylnaphthalen-2-ylamino)–2-aminopropanoic acid (ANAP) in TM2 causes leakiness and affects current facilitation.
(A) Representative current traces showing the effect of the P2X7 antagonist A438079 (100 µM) on baseline currents of mutants containing ANAP within TM2 (T340*, L341*) and on wt P2X7. A438079 was diluted from a 10 mM stock in Dimethyl sulfoxide (DMSO) using divalent-free buffer and applied into the static bath. Control experiments were conducted with 1% DMSO. Oocytes were clamped at –30 mV. (B) Box plot showing 10–50% rise times (in seconds) of the first and second current (black) and fluorescence (blue) responses to 0.3 mM ATP (interval 195 s) for P2X7(T340*). Significance was determined using the two-tailed paired Student’s t-test (*, p<0.05; ns, not significant). Original recordings have also been deposited with Dryad and summarized and assigned in Table 1—source data 1.
-
Figure 4—figure supplement 1—source data 1
Original recordings, Figure 4—figure supplement 1A.
- https://cdn.elifesciences.org/articles/82479/elife-82479-fig4-figsupp1-data1-v2.zip
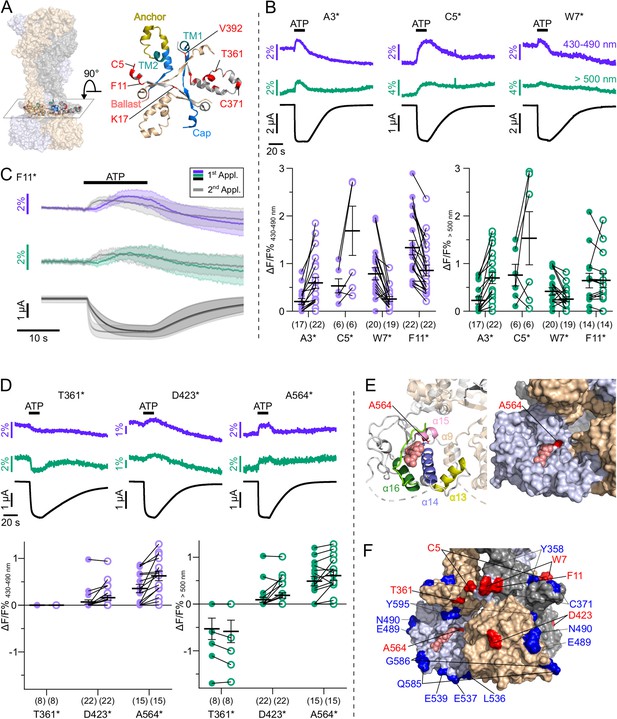
L-3-(6-acetylnaphthalen-2-ylamino)–2-aminopropanoic acid (ANAP) incorporation into 41 positions of the cytosolic P2X7 domain identified seven positions that report ATP-induced fluorescence changes.
(A) Surface representation of all three P2X7 subunits (in wheat, gray, and purple) showing location of the juxtamembrane regions and close up (top view) detailing the anchor and cap domains (in yellow and blue, respectively) and ANAP-substituted positions (in red) within a single P2X7 subunit. (B) Representative voltage clamp fluorometry (VCF) recordings and data summary from P2X7R mutants containing ANAP at different positions within the N-terminus. Responses to 0.3 mM ATP were recorded at two different emission wavelengths. Graphs compare maximal fluorescence signals during first (closed circles) and second (open circles) ATP applications (interval 195 s). Data are represented as mean ± S.E.M. (C) Overlay of VCF recordings from P2X7(F11*) upon first (colored) and second (gray) ATP application (0.3 mM) at two different emission wavelengths. Lines represent averaged VCF recordings from 13 oocytes. Standard deviations are plotted as envelopes. Baseline currents (15 s before ATP application) were adjusted for clarity. (D) Representative VCF recordings from the indicated mutants in response to a second application of 0.3 mM ATP and summary of results at the indicated emission wavelengths (performed as in B). Graphs compare maximal fluorescence signals during first (closed circles) and second (open circles) ATP applications (interval 195 s). Data are represented as mean ± S.E.M. All recordings were performed in divalent-free buffer, and oocytes were clamped at –30 mV. (E) Close-up of the cytoplasmic ballast domain from one P2X7 subunit in cartoon and surface representation highlighting a bound GDP (salmon), surrounding α-helices, and residue A564 (red). (F) Surface representation of the cytoplasmic domains of all three P2X7 subunits (in gray, light blue, and wheat) with bound GDP (salmon). Positions in which ATP-induced ANAP fluorescence changes were identified are shown in red. ANAP-substituted positions in which no fluorescence changes were seen (despite surface expression and current responses) are shown in blue. Original recordings have also been deposited with Dryad and summarized and assigned in Table 1—source data 1.
-
Figure 5—source data 1
Original recordings, Figure 5B and D.
- https://cdn.elifesciences.org/articles/82479/elife-82479-fig5-data1-v2.zip
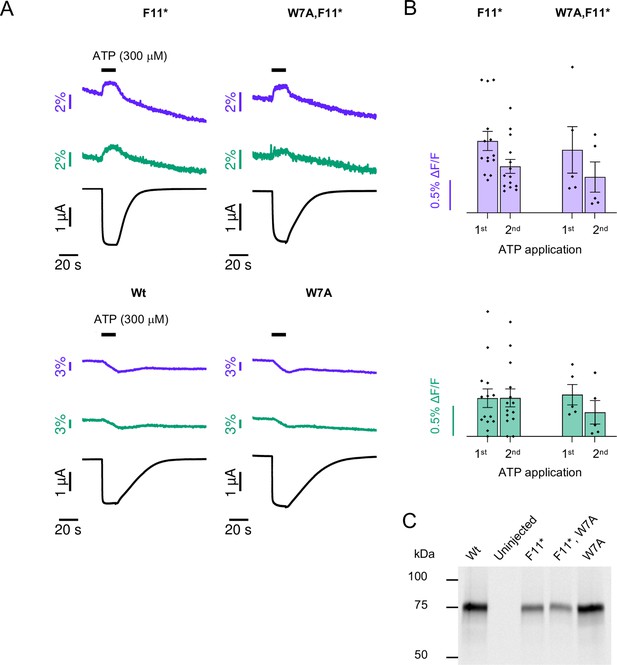
The fluorescence change in P2X7(F11*) is not caused by a dequenching effect of the nearby Trp residue.
(A, B) Comparison of voltage clamp fluorometry recordings from oocytes expressing P2X7(F11*) with or without Trp in position 7 (W7A, F11*). Oocytes expressing either non-mutated P2X7R or P2X7(W7A) served as controls. Recordings from P2X7(F11*) and P2X7 (W7A, F11*) are not significantly different, indicating that L-3-(6-acetylnaphthalen-2-ylamino)–2-aminopropanoic acid in position 11 is not quenched by Trp7. Data are represented as mean ± S.E.M. (C) Surface expression of P2X7(F11*), P2X7(F11*, W7A), and P2X7(W7A), with unmutated P2X7 and uninjected oocytes as positive and negative controls, respectively.
-
Figure 5—figure supplement 1—source data 1
Summarized data for bar graph in Figure 5—figure supplement 1B.
- https://cdn.elifesciences.org/articles/82479/elife-82479-fig5-figsupp1-data1-v2.zip
-
Figure 5—figure supplement 1—source data 2
Original recordings, Figure 5—figure supplement 1A and B.
- https://cdn.elifesciences.org/articles/82479/elife-82479-fig5-figsupp1-data2-v2.zip
-
Figure 5—figure supplement 1—source data 3
Original gel, Figure 5—figure supplement 1C.
- https://cdn.elifesciences.org/articles/82479/elife-82479-fig5-figsupp1-data3-v2.zip
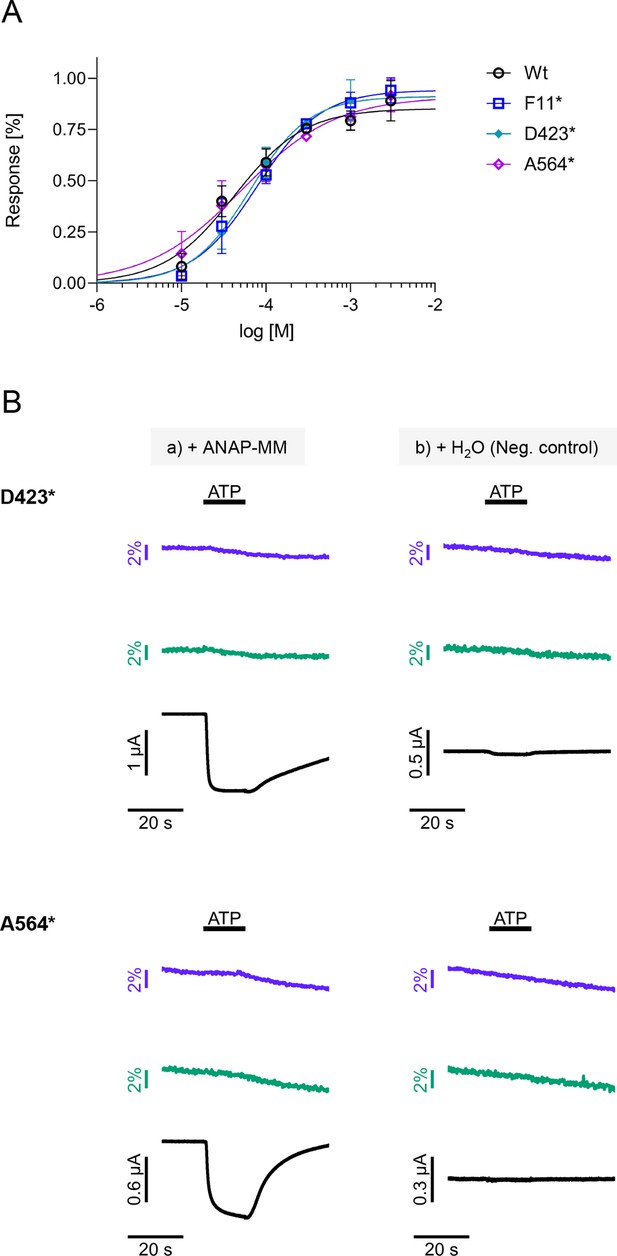
Dose-response analysis for intracellular P2X7 mutants F11*, D423*, and A564* and contribution of D423 and A564 deletion mutants to current responses.
(A) Normalized dose-response curves for ATP at wt P2X7 and the indicated L-3-(6-acetylnaphthalen-2-ylamino)–2-aminopropanoic acid (ANAP)-containing receptors. For EC50 values see Table 2. Error bars represent S.D. of three to eight experiments. (B) Representative voltage clamp fluorometry (VCF) recordings from oocytes that were injected with cRNA encoding P2X7 containing an amber stop codon at D423 or A564 with either ANAP and a master mix containing tRNA-synthetase cRNA, tRNA, and eRF1(E55D) cRNA, or with water to produce P2X7 protein truncated at these positions. ANAP emission was recorded at two different wavelengths (purple: 430–490 nm, green: >500 nm). VCF recordings showed clear ATP-evoked signals only for the oocytes injected with all essential components necessary for ANAP incorporation. Current responses of truncated P2X7Rs were less than 5% of those from positive controls. All recordings were performed in divalent-free buffer, and oocytes were clamped at –30 mV.
-
Figure 5—figure supplement 2—source data 1
Original recordings, Figure 5—figure supplement 2B.
- https://cdn.elifesciences.org/articles/82479/elife-82479-fig5-figsupp2-data1-v2.zip
-
Figure 5—figure supplement 2—source data 2
Summarized data, Figure 5—figure supplement 2B.
- https://cdn.elifesciences.org/articles/82479/elife-82479-fig5-figsupp2-data2-v2.zip
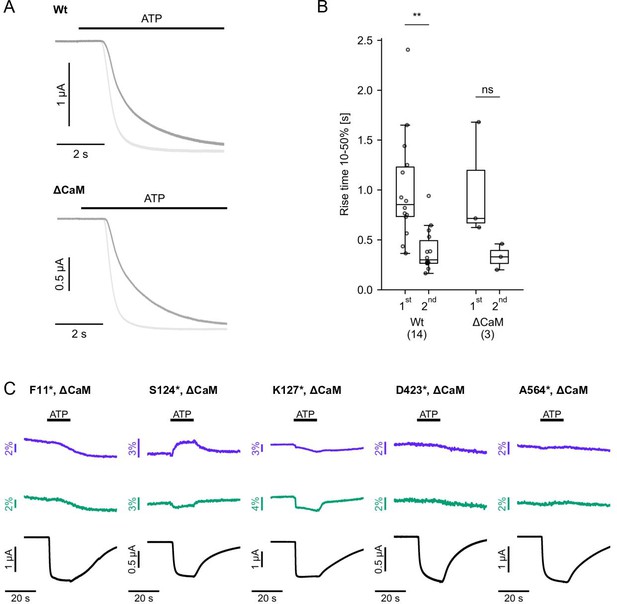
Elimination of a CaM-binding motif has no apparent effect on current kinetics or fluorescence responses.
(A) ΔCaM-P2X7 contained the single point mutations I541T, S552C, and V559G (Roger et al., 2010). Overlay of representative current traces during first (dark gray) and second (light gray) ATP applications (0.3 mM, applied in 195 s interval). Baseline currents (15 s before ATP application) were adjusted for clarity. (B) Box plot showing 10–50% rise times (in seconds) of the first and second current responses for wt P2X7 and ΔCaM P2X7. Significance was determined using the two-tailed paired Student’s t-test (**, p<0.005; ns, not significant). All recordings were performed in divalent-free buffer, and oocytes were clamped at –30 mV. (B) Representative voltage clamp fluorometry (VCF) recordings in response to the second ATP application of the indicated mutants ΔCaM F11*, n=2 (3); ΔCaM, S124*, n=1 (3); ΔCaM, K127*, n=3 (8); ΔCaM, D423*, n=4 (9); ΔCaM, A564*, n=3 (15) with n and numbers in brackets indicating the number of successful and total VCF recordings, respectively. All recordings were performed in divalent-free buffer, and oocytes were clamped at –30 mV.
-
Figure 5—figure supplement 3—source data 1
Summarized data for box plot in Figure 5—figure supplement 3A and representative VCF recordings in Figure 5—figure supplement 3B.
- https://cdn.elifesciences.org/articles/82479/elife-82479-fig5-figsupp3-data1-v2.zip
-
Figure 5—figure supplement 3—source data 2
Original recordings, Figure 5—figure supplement 3A and B.
- https://cdn.elifesciences.org/articles/82479/elife-82479-fig5-figsupp3-data2-v2.zip
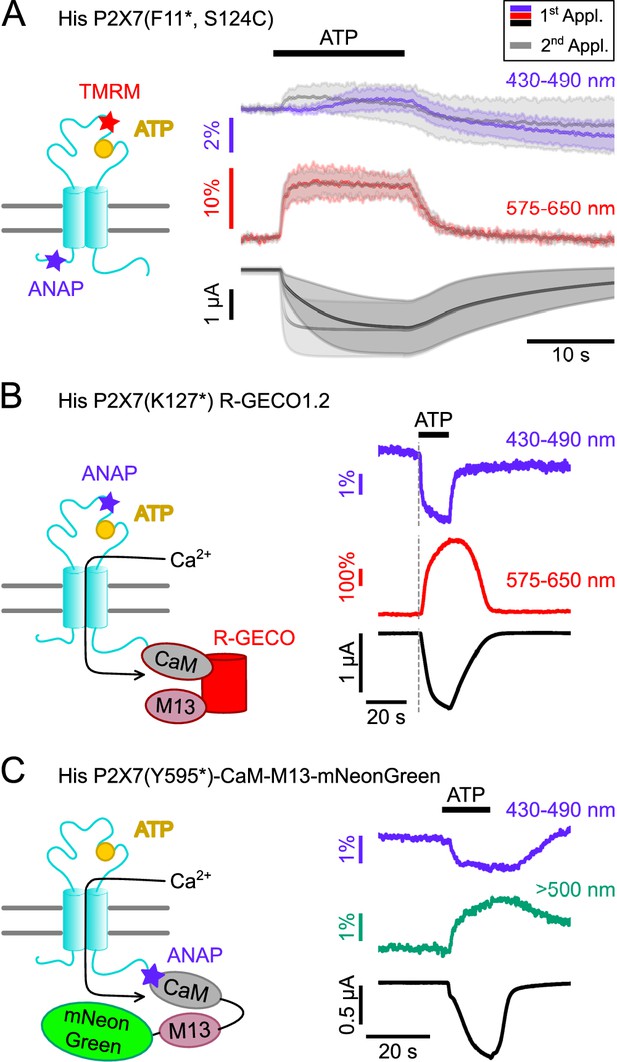
Double-labeled P2X7Rs as potential tools to analyze intracellular domain movements, downstream signaling events, and protein interactions.
(A) Scheme of a P2X7 subunit double-labeled with L-3-(6-acetylnaphthalen-2-ylamino)–2-aminopropanoic acid (ANAP) and tetramethyl-rhodamine-maleimide (TMRM) (F11*, S124C) and overlay of fluorescence and current responses to first (colored) and second (gray) ATP applications (0.3 mM) at the indicated emission wavelengths. Lines represent averaged voltage clamp fluorometry (VCF) recordings from five different oocytes and standard deviations are plotted as envelopes. Baseline currents (15 s before ATP application) were adjusted for clarity. (B) Scheme of P2X7(K127*) subunit C-terminally fused to R-GECO1.2 and representative VCF recording in response to 0.3 mM ATP. Recordings were performed in buffer containing 0.5 mM Ca2+. (C) Scheme showing the P2X7(Y595*)-CaM-M13-mNeonGreen construct that served as positive control for recordings of FRET between ANAP and mNeonGreen. Ca2+ entry through the P2X7R is supposed to induce conformational changes in the CaM-M13-mNeonGreen reporter, which are detected as a FRET signal. A representative VCF recording in response to 0.3 mM ATP is shown. In all recordings, oocytes were clamped at –30 mV.
-
Figure 6—source data 1
Original recordings, Figure 6A, B, and C.
- https://cdn.elifesciences.org/articles/82479/elife-82479-fig6-data1-v2.zip
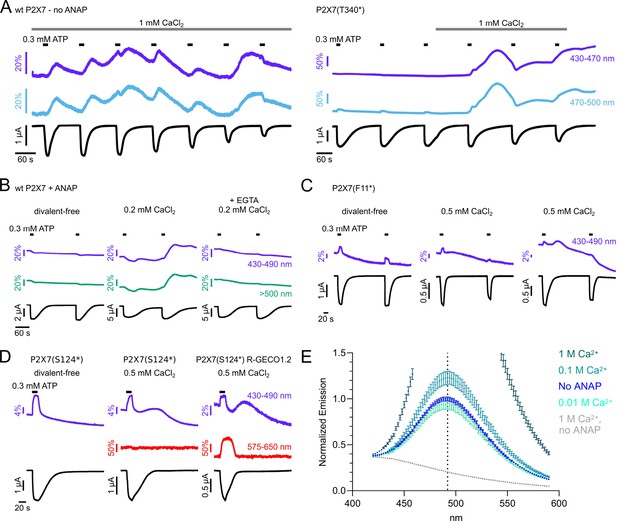
Ca2+-containing buffers cause large fluorescence changes, even in the absence of L-3-(6-acetylnaphthalen-2-ylamino)–2-aminopropanoic acid (ANAP).
(A) In Ca2+-containing buffers, voltage clamp fluorometry (VCF) recordings from unmutated wt P2X7R (left) showed large irregular fluorescence changes in the ANAP emission range, even in the absence of ANAP. These masked ATP-evoked and ANAP-specific fluorescence signals from ANAP-substituted P2X7(T340*) (right). (B) The irregular fluorescence changes in Ca2+-containing buffers could be prevented by injection of EGTA (1 mM) 3–4 hr before the measurement. (C) VCF recordings of oocytes expressing P2X7(F11*) in divalent-free buffer supplemented with EGTA and flufenamic acid (left) and in buffer containing 0.5 mM Ca2+ (center and right). (D) VCF recordings of oocytes expressing P2X7(S124*) and P2X7(S124*)-R-GECO1.2. The Ca2+-dependent fluorescence changes are only detected in the ANAP emission spectrum. If not otherwise indicated, recordings were performed at –30 mV in divalent-free buffer supplemented with EGTA and flufenamic acid. (E) ANAP (1 μM) and the indicated CaCl2 concentrations were dissolved in otherwise divalent-free recording solution. Fluorescence emission spectra were measured using a Tecan Reader Infinite M200 Pro (excitation 360 nm) and normalized to the averaged fluorescence emission at the maximum emission (492 nm, dotted line) of buffer containing only ANAP. Note that values for the (1 M Ca2+ + ANAP)–solution were out of measurement range.
-
Figure 6—figure supplement 1—source data 1
Original recordings, Figure 6—figure supplement 1A, B, C.
- https://cdn.elifesciences.org/articles/82479/elife-82479-fig6-figsupp1-data1-v2.zip
-
Figure 6—figure supplement 1—source data 2
Original and summarized photometric data for Figure 6—figure supplement 1E.
- https://cdn.elifesciences.org/articles/82479/elife-82479-fig6-figsupp1-data2-v2.zip
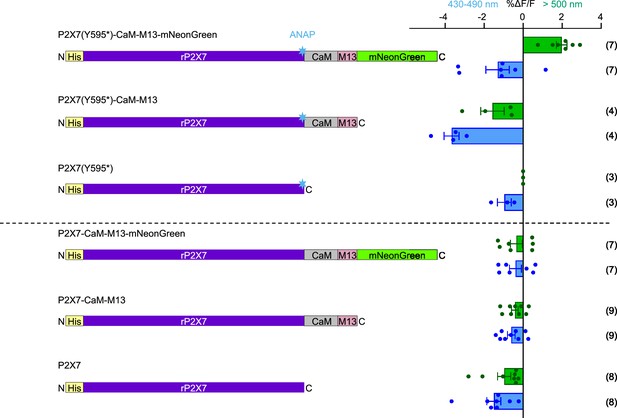
Control constructs and corresponding voltage clamp fluorometry recordings to confirm the specificity of the FRET signals.
Scheme representing the constructs tested (left) with fluorescence signals at two different emission wavelengths (for L-3-(6-acetylnaphthalen-2-ylamino)–2-aminopropanoic acid [ANAP; blue] and mNeonGreen [green], respectively) summarized as horizontal bar diagrams (right). The positive control P2X7(Y595*)-CaM-M13-mNeonGreen is the only construct showing negative fluorescence signals for ANAP and positive signals for mNeonGreen, consistent with FRET. The control constructs containing only ANAP show either negative or no fluorescence signal for mNeonGreen. Constructs without sites for ANAP incorporation but co-injected ANAP (as master mix with tRNA and cRNAs) served as a control for background fluorescence. Error bars represent S.E.M. Numbers of experiments are given in brackets.
-
Figure 6—figure supplement 2—source data 1
Summarized data for bar graph in Figure 6—figure supplement 2.
- https://cdn.elifesciences.org/articles/82479/elife-82479-fig6-figsupp2-data1-v2.zip
-
Figure 6—figure supplement 2—source data 2
Original recordings for bar graph in Figure 6—figure supplement 2.
- https://cdn.elifesciences.org/articles/82479/elife-82479-fig6-figsupp2-data2-v2.zip
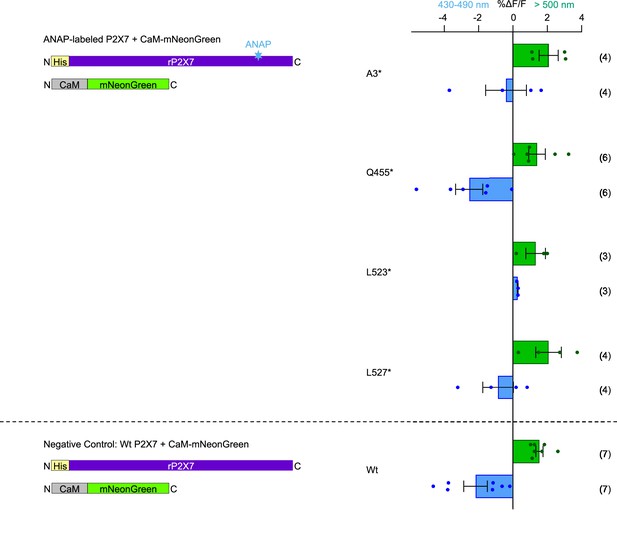
Experiments with L-3-(6-acetylnaphthalen-2-ylamino)–2-aminopropanoic acid (ANAP)-containing P2X7 constructs and soluble mNeonGreen-tagged CaM reveal unspecific fluorescence signals.
The indicated ANAP-containing P2X7 constructs were co-injected with soluble mNeonGreen-tagged CaM and ANAP-Master Mix containing ANAP, tRNA, cRNA encoding tRNA-syntethase, and cRNA encoding eRF1(E55D). Corresponding fluorescence signals at two different emission wavelengths are summarized for ANAP (blue) and mNeonGreen (green). Note that soluble mNeonGreen-tagged CaM appeared to interact with the co-injected ANAP. Error bars represent S.E.M. Numbers of experiments are given in brackets.
-
Figure 6—figure supplement 3—source data 1
Summarized data for bar graph in Figure 6—figure supplement 3.
- https://cdn.elifesciences.org/articles/82479/elife-82479-fig6-figsupp3-data1-v2.zip
-
Figure 6—figure supplement 3—source data 2
Original recordings for bar graph in Figure 6—figure supplement 3.
- https://cdn.elifesciences.org/articles/82479/elife-82479-fig6-figsupp3-data2-v2.zip
Tables
Summary of surface expression, current responses (ΔI), and L-3-(6-acetylnaphthalen-2-ylamino)–2-aminopropanoic acid fluorescence changes (%ΔF/F) of the investigated P2X7 mutants.
Position | Surface expression | ΔI | %ΔF/F Filter set 1 | %ΔF/F Filter set 2 | ||||
---|---|---|---|---|---|---|---|---|
Full-length | Truncated | 430–470 nm | 470–500 nm | 430–490 nm | >500 nm | |||
N-terminus | A3 | + | – | + | ↑ | ↑ | ↑ | ↑ |
C5 | + | – | + | (–) | (–) | ↑ | ↑ | |
S6 | + | – | – | (–) | (–) | (–) | (–) | |
W7 | + | – | + | (↑) | (↑) | ↑ | ↑ | |
V10 | + | – | (+) | (–) | (–) | (↑) | (↑) | |
F11 | + | – | + | (–) | (–) | ↑ | ↑ | |
K17 | + | – | (+) | (–) | (–) | (–) | (–) | |
Head domain | P120 | + | – | + | (↑) | (↑) | ↑ | ↑ |
E121 | + | – | + | (–) | (–) | ↑ | ↑ | |
Y122 | + | – | + | ↓ | ↓ | ↓ | ↓ | |
P123 | + | – | + | n.d. | n.d. | ↓ | ↓ | |
S124 | + | – | + | ↑ | ↑ | ↑ | ↓ | |
R125 | + | – | + | ↑ | ↑ | ↑ | ↓ | |
G126 | + | – | + | ↑ | ↑ | ↑ | ↑ | |
K127 | + | – | + | ↓ | ↓ | ↓ | ↓ | |
Q128 | + | – | + | – | – | ↑ | ↓ | |
TM2 | G338 | – | – | – | n.d. | n.d. | (–) | (–) |
S339 | + | – | + | ↑ | ↑ | ↑ | ↑ | |
T340 | + | – | + | – / ↓ | ↑ | ↑ | ↑ | |
L341 | + | – | + | (↑) | (↑) | ↑ | ↑ | |
C-terminus | N356 | + | – | (+) | (–) | (–) | (–) | (–) |
T357 | + | + | (+) | (–) | (–) | (–) | (–) | |
Y358 | + | + | + | (–) | (–) | – | – | |
A359 | + | + | (+) | (–) | (–) | (–) | (–) | |
T361 | + | + | + | (–) | (↓) | – | ↓ | |
R364 | + | + | (+) | n.d. | n.d. | (–) | (–) | |
C371 | + | + | + | – | – | (–) | (–) | |
A378 | + | + | (+) | (–) | (–) | n.d. | n.d. | |
R385 | + | + | (+) | n.d. | n.d. | (–) | (–) | |
K387 | + | + | – | n.d. | n.d. | (–) | (–) | |
C388 | + | + | – | n.d. | n.d. | (–) | (–) | |
V392 | + | + | (+) | (–) | (–) | (–) | (–) | |
E406 | + | + | (+) | (–) | (–) | (–) | (–) | |
Q422 | + | + | – | n.d. | n.d. | (–) | (–) | |
D423 | + | + | + | (–) | (–) | ↑ | ↑ | |
V424 | + | + | – | n.d. | n.d. | (–) | (–) | |
Q433 | + | + | (+) | (–) | (–) | n.d. | n.d. | |
T434 | + | + | (+) | (–) | (–) | n.d. | n.d. | |
F436 | + | + | + | n.d. | n.d. | (–) | (–) | |
Unresolved | S445 | + | + | + | (–) | (–) | – | (–) |
P450 | + | + | + | – | – | – | – | |
Q455 | + | + | + | – | – | – | – | |
Q460 | + | + | (+) | (–) | (–) | (–) | (–) | |
E465 | + | + | (+) | (–) | (–) | n.d. | n.d. | |
S470 | + | + | (+) | (–) | (–) | n.d. | n.d. | |
C-terminus | E489 | + | + | + | (–) | (–) | – | – |
N490 | + | + | + | (–) | (–) | – | – | |
V517 | + | + | + | n.d. | n.d. | – | – | |
L523 | + | + | + | n.d. | n.d. | – | – | |
L527 | + | + | + | n.d. | n.d. | – | – | |
L536 | + | + | + | (–) | (–) | – | – | |
E537 | + | + | + | – | – | – | – | |
G538 | – | + | – | (–) | (–) | (–) | (–) | |
E539 | + | + | + | (–) | (–) | – | – | |
A564 | + | ? | + | n.d. | n.d. | ↑ | ↑ | |
L569 | + | ? | (+) | n.d. | n.d. | (–) | (–) | |
I577 | + | ? | – | n.d. | n.d. | (–) | (–) | |
Q585 | + | ? | + | (–) | (–) | – | – | |
G586 | + | ? | + | (–) | (–) | – | – | |
Y595 | + | ? | + | – | – | – | – | |
596 | + | ? | + | – | – | – | – |
-
+ and − indicate presence and absence of protein or signals, respectively. In case of current responses, + means response comparable to wt receptors and (+) means reduced responses. ↑ and ↓ indicate positive and negative fluorescence signals, respectively. 3–50 oocytes were measured per construct and filter set. In case of fluorescence responses, symbols in brackets indicate where less than three recordings met the criteria defined in the methods (mostly because of impaired fucntionality) and represent tendencies only. ?, not distinguishable (because of similar length of full-length and truncated constructs); n.d., not determined.
-
Table 1—source data 1
Summarized data for Table 1 with assignment to the original VCF recordings; also including data from Figure 2—figure supplement 1B (box plot); Figure 3C, D, E, F; Figure 3—figure supplement 3C; Figure 4B, C, D; Figure 4—figure supplement 1B; and Figure 5B, C, D. The respective original recordings are deposited with Dryad.
- https://cdn.elifesciences.org/articles/82479/elife-82479-table1-data1-v2.zip
EC50 values for ATP and Hill coefficients (nH) at wt and L-3-(6-acetylnaphthalen-2-ylamino)–2-aminopropanoic acid-containing P2X7 receptor constructs.
Mutant | EC50 (M) | nH |
---|---|---|
Wt | 4.202e-005 (3.211e-005–5.704e-005) | 1.049 (0.7962–1.380) |
F11* | 7.802e-005 (6.268e-005–9.893e-005) | 1.148 (0.9345–1.410) |
S124* | 8.316e-005 (6.068e-005–0.0001236) | 1.122 (0.8271–1.519) |
F11*, S124C | 0.0001003 (8.439e-005–0.0001216) | 1.290 (1.069–1.571) |
K127* | 6.511e-005 (4.281e-005–0.0001339) | 0.6601 (0.4779–0.8662) |
D423* | 6.513e-005 (4.729e-005–0.0001057) | 1.240 (0.8087–1.821) |
A564* | 5.159e-005 (3.491e-005–9.976e-005) | 0.7810 (0.5364–1.087) |
-
Number in brackets are 95% confidence intervals, n=3–11.
-
Table 2—source data 1
Original recordings for Table 2; Figure 3—figure supplement 3D; and Figure 5—figure supplement 2A.
- https://cdn.elifesciences.org/articles/82479/elife-82479-table2-data1-v2.zip
-
Table 2—source data 2
Summarized data for Table 2; Figure 3—figure supplement 3D; and Figure 5—figure supplement 2A.
- https://cdn.elifesciences.org/articles/82479/elife-82479-table2-data2-v2.zip
Reagent type (species) or resource | Designation | Source or reference | Identifiers | Additional information |
---|---|---|---|---|
Recombinant DNA reagent | cDNA Xenopus laevis eRF1(E55D) | NCBI Reference Sequence: NM_001090894.1, Life Technologies / Thermo Fisher Scientific | GeneArt String DNA fragment (cloned into pET28a and pNKS2) | |
Recombinant DNA reagent | cDNA Rattus norvegicus P2X7 | in modified pUC19 (pUC19o) | ||
Recombinant DNA reagent | cDNA Rattus norvegicus P2X1 | Lörinczi et al., 2012 | in pNKS2 | |
Recombinant DNA reagent | cDNA Rattus norvegicus calmodulin-1 | UniProt: PDP29; NCBI Reference Sequence: NM_031969.3 | Codon-optimized for Xenopus laevis (Invitrogen / Thermo Fisher Scientific), C-terminally linked to Rattus norvegicus P2X7 via GS-linker (ggatct) | |
Recombinant DNA reagent | Plasmid: pNKS2 | Gloor et al., 1995 | ||
Recombinant DNA reagent | Plasmid: pUC19 | New England Biolabs GmbH | CAT# N3041S | |
Recombinant DNA reagent | Plasmid: pANAP | Chatterjee et al., 2013 | CAT#48696 | |
Recombinant DNA reagent | EGFP | Yang et al., 1996 | the original enhanced GFP, mammalian codon-optimized, C-terminally linked to Rattus norvegicus P2X1 via GSAGSA-linker sequence (ggatctgcaggatctgca) | |
Recombinant DNA reagent | R-GECO1.2 | Wu et al., 2013 | Addgene #45494 | |
Recombinant DNA reagent | mNeonGreen | Shaner et al., 2013 | Codon-optimized for Xenopus laevis (Invitrogen / Thermo Fisher Scientific) | |
Recombinant DNA reagent | M13-like peptide from CaM-dependent kinase | Rattus norvegicus myosin light chain kinase, smooth muscle; Uniprot: D3ZFU9 | RRKWQKTGNAVRAIGRLSSM; cloned between Rattus norvegicus calmodulin-1 and mNeonGreen with N- and C-terminal GS-linkers (ggcagc and ggatct, respectively) | |
Sequence-based reagent | Oligonucleotides | metabion GmbH | ||
Sequence-based reagent | Amber suppressor tRNA, synthesized oligonucleotide, sequence derived from pANAP, an universal 3’-terminal CCA-sequence was added: 5'-gcc cgg aug gug gaa ucg gua gac aca agg gau ucu aaa ucc cuc ggc guu cgc gcu gug cgg guu caa guc ccg cuc cgg gua cca –3' | biomers.net GmbH; Chatterjee et al., 2013; Durner and Nicke, 2022 | ||
Sequence-based reagent | Sanger sequencing | Eurofins Genomics, https://eurofinsgenomics.eu/ | ||
Sequence-based reagent | 5’-UTR, GeneArt String DNA fragment (cloned into pUC19 (small letters) before the start codon (italic letters)), gtacccggggatcctctTAATACGACTCACTATAGGCTTGTTCTTTTTGCAGAAGCTCAGAATAAACGCTCAACTTTGGCTCGAGGCCACCatg | Life Technologies / Thermo Fisher Scientific, Kozak, 1987 | ||
Sequence-based reagent | 3’-UTR, (cloned into pUC19 (small letters) after the stop codon (italic letters)), tgaCCCAAAACAAAAACGGAATATG CAAACAAAAAAAAAAAAAAAAAAAAAAAAAAAAAAAAAAAAAAAAAAAAAAAAAAAGAATTCTAGAGCGGCCGCagagtcgacctgcagg | pNKS2, Gloor et al., 1995 | ||
Peptide, recombinant protein | EcoRI-HF | New England Biolabs GmbH | CAT#R3101S | |
Peptide, recombinant protein | NotI-HF | New England Biolabs GmbH | CAT#R3189S | |
Commercial assay or kit | Gibson Assembly Master Mix | New England Biolabs GmbH | CAT#E2611L | |
Commercial assay or kit | Q5 Site-Directed Mutagenesis Kit | New England Biolabs GmbH | CAT#E0552S | |
Commercial assay or kit | MinElute Reaction Cleanup Kit | QIAGEN GmbH | CAT#28204 | |
Commercial assay or kit | ||||
Commercial assay or kit | Macherey-Nagel NucleoSpin Gel and PCR Clean-up Kit | Fisher Scientific / Thermo Fisher Scientific | CAT# 11992242 | |
Commercial assay or kit | mMESSAGE mMACHINE T7 Transcription Kit | Invitrogen / Thermo Fisher Scientific | CAT# AM1344 | |
Commercial assay or kit | mMESSAGE mMACHINE SP6 Transkription Kit | Invitrogen / Thermo Fisher Scientific | CAT#AM1340 | |
Chemical compound, drug | ATP disodium salt hydrate | Sigma-Aldrich | Cat#A3377 | |
Chemical compound, drug | L-ANAP trifluoroacetic salt | AsisChem Inc. | Cat#ASIS-0014 | |
Chemical compound, drug | L-ANAP methyl ester | AsisChem Inc. | Cat#ASIS-0146 | |
Chemical compound, drug | Collagenase NB 4 G proved grade | Nordmark Pharma GmbH | Cat#S1746502 | |
Chemical compound, drug | Gentamicin sulfate | Roth | CAT#0233.4 | |
Chemical compound, drug | Cy5 Mono NHS Ester | Merck / Sigma-Aldrich | CAT#GEPA15101 | |
Chemical compound, drug | Pefabloc SC | Merck / Sigma Aldrich | CAT#76307 | |
Chemical compound, drug | n-Dodecyl-β-D-Maltoside, ULTROL grade | Merck / Sigma Aldrich | CAT#324355 | |
Chemical compound, drug | Ni-NTA Agarose | QIAGEN GmbH | CAT#1018244 | |
Chemical compound, drug | Flufenamic acid | Merck / Sigma Aldrich | CAT#F9005 | |
Chemical compound, drug | 0.5 M EDTA ph 8.0 | Thermo Scientific | CAT#R1021 | |
Chemical compound, drug | TMRM | Biomol | CAT#ABD-419 | |
Chemical compound, drug | A 438079 hydrochloride | TOCRIS | CAT#2972 | |
Software, algorithm | CellWorks E 5.5.1 | npi electronic, http://cellworks.de/ | ||
Software, algorithm | PyMOL | http://www.pymol.org/ | RRID:SCR_000305 | |
Software, algorithm | Python Programming Language 3.10.4 | http://www.python.org/ | RRID:SCR_008394 | |
Software, algorithm | NumPy 1.22.3 | http://www.numpy.org | RRID:SCR_008633 | |
Software, algorithm | MatPlotLib 3.5.1 | http://matplotlib.sourceforge.net | RRID:SCR_008624 | |
Software, algorithm | SciPy 1.8.0 | http://www.scipy.org/ | RRID:SCR_008058 | |
Software, algorithm | GraphPad Prism 9.3.0 and 9.5.0 | http://www.graphpad.com/ | RRID:SCR_002798 | |
Software, algorithm | (Fiji Is Just) ImageJ 2.3.0 | Schindelin et al., 2012, http://fiji.sc | RRID:SCR_002285 | |
Other | Turbo Tec-05X Amplifier | npi electronic GmbH | CAT#TEC-05X | VCF-Setup components, electronics |
Other | PCI-6221, DAQ, Multifunction I/O Device, 16-Bit | National Instruments | CAT# 779066–01 | VCF-Setup components, electronics |
Other | Single-channel fully programmable Instrumentation Amplifier Low Pass Filter, USBPGF-S1/L with 8th pole Bessel filter characteristics | Alligator Technologies | CAT#USBPGF-S1/L | VCF-Setup components, electronics |
Other | 2 x MPPC modules | Hamamatsu Photonics K.K. | CAT#C13366-3050GA | VCF-Setup components, electronics |
Other | Power adapter/linear regulator | KNIEL System-Electronic GmbH | Custom-made | VCF-Setup components, electronics |
Other | Axiovert 200 inverted fluorescence microscope | Carl Zeiss Microscopy LLC | VCF-Setup components, optics | |
Other | Objektiv W N-Achroplan 63 x/0,9 M27 | Carl Zeiss Microscopy LLC | CAT#420987-9900-000 | VCF-Setup components, optics |
Other | M565L3, mounted LED at 565 nm | Thorlabs GmbH | CAT#M565L3 | VCF-Setup components, optics |
Other | M365LP1, Mid Power Mounted LED at 365 nm | Thorlabs GmbH | CAT#M365LP1 | VCF-Setup components, optics |
Other | 2× lenses for LED collimation | Thorlabs GmbH | CAT#ACL2520U-A | VCF-Setup components, optics |
Other | ET555/20×, 25 mm Dia Mounted, Single Bandpass Filter (for excitation) | Chroma Technology GmbH | CAT# IN026697 | VCF-Setup components, optics |
Other | ET365/20×, 25 mm Dia Mounted, Single Bandpass Filter (for excitation) | Chroma Technology GmbH | CAT# IN053211 | VCF-Setup components, optics |
Other | T387lp, 25.5×36×1 mm, Longpass Dichroic Beamsplitter | Chroma Technology GmbH | CAT# IN040921 | VCF-Setup components, optics |
Other | 79003bs, Multi Dichroic Beamsplitter | Chroma Technology GmbH | CAT# CS294227 | VCF-Setup components, optics |
Other | 59002bs, Multi Dichroic Beamsplitter | Chroma Technology GmbH | CAT# IN040206 | VCF-Setup components, optics |
Other | T425lpxr,25.5×36×1 mm, Longpass Dichroic Beamsplitter | Chroma Technology GmbH | CAT# IN025246 | VCF-Setup components, optics |
Other | Relay lense | Thorlabs GmbH | CAT#AC254-060-A | VCF-Setup components, optics |
Other | DMLP550R, Longpass Dichroic Beamsplitter | Thorlabs GmbH | CAT#DMLP550R | VCF-Setup components, optics |
Other | T470lpxr, Longpass Dichroic Beamsplitter | Chroma Technology GmbH | CAT# IN030502 | VCF-Setup components, optics |
Other | T495lpxr, 25.5×36×1 mm, Longpass Dichroic Beamsplitter | Chroma Technology GmbH | CAT# IN005752 | VCF-Setup components, optics |
Other | ET490/40×, 25 mm Dia Mounted (for emission) | Chroma Technology GmbH | CAT# IN039532 | VCF-Setup components, optics |
Other | ET610/75 m, 25 mm Dia Mounted (for emission) | Chroma Technology GmbH | CAT# IN036520 | VCF-Setup components, optics |
Other | ET455/50 m (for emission) | Chroma Technology GmbH | CAT# IN067607 | VCF-Setup components, optics |
Other | ET500lp, 25 mm Dia Mounted (for emission) | Chroma Technology GmbH | CAT# IN006640 | VCF-Setup components, optics |
Other | MF460-60 (for emission) | Thorlabs GmbH | CAT#MF460-60 | VCF-Setup components, optics |
Other | 2× lenses for focusing on MPPC | Thorlabs GmbH | CAT#LB1761-A | VCF-Setup components, optics |