Acetylation of a fungal effector that translocates host PR1 facilitates virulence
Figures
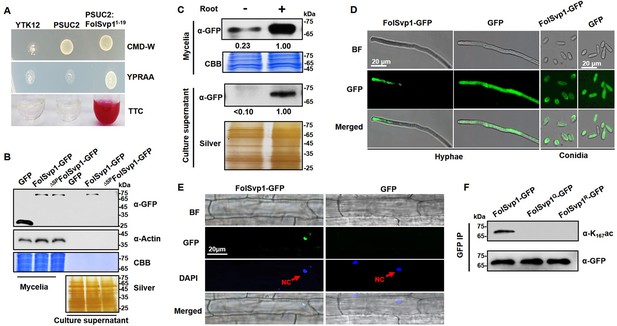
Secretion and K167 acetylation of Fol-Secreted Virulence-related Protein1 (FolSvp1).
(A) Functional validation of the signal peptide of FolSvp1. The indicated strains were grown on CMD-W or YPRAA medium for 3 days. Triphenyltetrazolium chloride (TTC) was used to test the enzymatic activity of reducing TTC to red triphenylformazan (TPF). (B) Secretion of FolSvp1. Conidia of strains carrying GFP or FolSvp1-GFP with or without signal peptide (SP) (ΔSPFolSvp1-GFP) were inoculated into 5% liquid YEPD in the presence of 2-week-old tomato roots. Forty hours after inoculation, total proteins were extracted from mycelia or culture supernatant, and probed with anti-GFP monoclonal antibody (α-GFP) and anti-Actin antibody (α-Actin), respectively. Coomassie brilliant blue (CBB) or silver staining shows protein loading to each lane. (C) Secretion of FolSvp1 with or without tomato roots. The amount of FolSvp1 in mycelia and secreted FolSvp1 in the presence of tomato roots was each set as 1. (D) Enrichment of FolSvp1-GFP at hyphal tips. Hyphae and conidia of the indicated strains were imaged. Scale bar, 20 µm. (E) Subcellular localization of FolSvp1 in tomato root cells. Three days after inoculation (DAI) by the indicated strains, tomato roots were imaged. Red arrows indicate the nuclei of plants. Scale bar, 20 µm. (F) Acetylation of the wild-type (WT) and mutant FolSvp1 proteins. FolSvp1 proteins pulled down from the indicated strains with GFP-Trap beads were probed with α-GFP and anti-K167ac antibody (α-K167ac), respectively. (D) and (F) were performed in the presence of tomato roots. Each gel shown is a representative experiment carried out three times.
-
Figure 1—source data 1
Uncropped images of gels and blots in Figure 1.
- https://cdn.elifesciences.org/articles/82628/elife-82628-fig1-data1-v2.zip
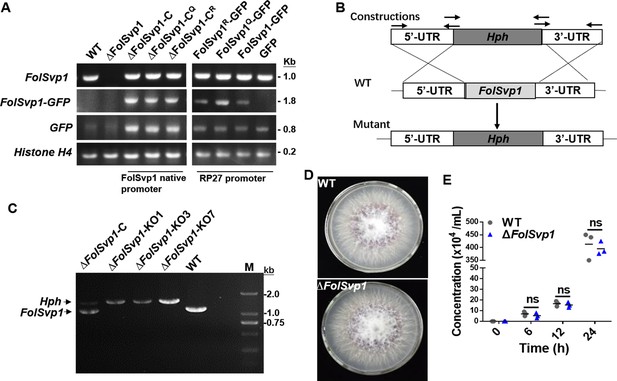
Generation of Fol-Secreted Virulence-related Protein1 (FolSvp1) mutant strains and phenotypic analysis.
(A) PCR analysis to determine ∆FolSvp1, ∆FolSvp1-C, and K167 mutant strains under its native promoter or PR27 constitutive promoter. Primer pair FolSvp1-PQB-F/R for amplification across the FolSvp1 coding sequence, FolSvp1-PQB-F and pYF11-cGFP-R for amplification of the FolSvp1 fused GFP coding sequences, primer pair pYF11-cGFP-F/R for amplification of the GFP coding sequences. Primer pair H4-qRT-F/R specific to Histone H4 (FOXG_09402) was used as a positive PCR control. Genomic DNA was analyzed by PCR with the indicated primer pairs. (B) Homologous recombination-based deletion of FolSvp1. (C) PCR analysis to identify the ∆FolSvp1 (KO1, KO3, and KO7) and ∆FolSvp1-C strains with primer pair FolSvp1-out-F/R. (D) Mycelial growth of the wild-type (WT) and ∆FolSvp1 (∆FolSvp1-KO7) mutant strains on potato dextrose agar (PDA) plates after 5 days of cultivation. (E) Conidia production of the WT and ∆FolSvp1 mutant strains in PDB liquid medium at the indicated times. No significant difference (ns) was indicated according to multiple t-test.
-
Figure 1—figure supplement 1—source data 1
Uncropped images of gels in Figure 1—figure supplement 1.
- https://cdn.elifesciences.org/articles/82628/elife-82628-fig1-figsupp1-data1-v2.zip
-
Figure 1—figure supplement 1—source data 2
Statistical analysis in Figure 1—figure supplement 1.
- https://cdn.elifesciences.org/articles/82628/elife-82628-fig1-figsupp1-data2-v2.xlsx
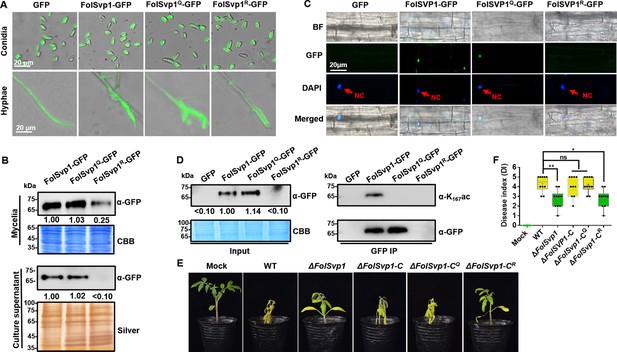
Contribution of K167 acetylation to Fol-Secreted Virulence-related Protein1 (FolSvp1) secretion and Fusarium oxysporum f. sp. lycopersici (Fol) virulence.
(A) Location of the wild-type (WT) and mutant FolSvp1 proteins in conidia and hyphae. Scale bar, 20 µm. (B) Level of the WT and Q, R mutant FolSvp1 proteins in mycelia and their secreted amount. The amount of WT FolSvp1 in mycelia and secreted was each set as 1. (C) Subcellular localization of the WT and Q, R mutant FolSvp1 proteins in tomato root cells. Red arrows indicate the nucleus. Scale bar, 20 µm. For (A–C), location, amount, secretion, and subcellular localization of FolSvp1 proteins were determined as in Figure 1. (D) Amount and acetylation of the WT and Q, R mutant FolSvp1 proteins in tomato root cells. Nuclear proteins were extracted from protoplast of tomato root cells and immunoprecipitated with GFP-Trap beads. FolSvp1-GFP proteins pulled down were then probed with α-GFP and α-K167ac, respectively (right). Input proteins were shown by Western blotting with α-GFP and CBB staining (left). The amount of WT FolSvp1 was set as 1. (E) Virulence of the WT and FolSvp1 mutant strains on tomato. Two-week-old tomato seedlings were inoculated with conidial suspension (5×106 spores/ml) of the indicated strains. The disease symptoms were observed, and photographs were taken at 14 days after inoculation (DAI). Mock, inoculation with water. (F) Disease index scored at 14 DAI. Star represents significant differences according to one-way analysis of variance (ANOVA) (**p<0.01, *p<0.05; ns, no significance; n=10). Whiskers of the boxplots display the upper and lower quartiles, the boxes display the interquartile range, and the plus displays the mean. Each gel shown is a representative experiment carried out three times.
-
Figure 2—source data 1
Uncropped gels and blots in Figure 2.
- https://cdn.elifesciences.org/articles/82628/elife-82628-fig2-data1-v2.zip
-
Figure 2—source data 2
Statistical analysis in Figure 2.
- https://cdn.elifesciences.org/articles/82628/elife-82628-fig2-data2-v2.xlsx
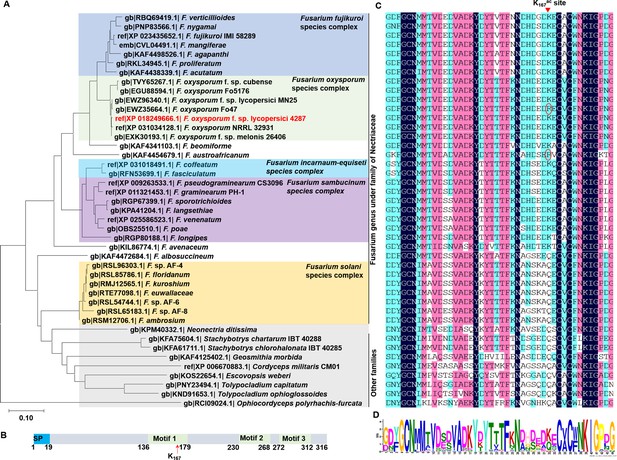
Phylogenetic and structural analyses of Fol-Secreted Virulence-related Protein1 (FolSvp1) homologs.
(A) Phylogenetic analysis of FolSvp1 homologs in Fusarium genus and other families under Hypocreales fungi. The phylogenetic tree was generated based on a neighbor-joining analysis using MEGA-X. (B) Motif prediction of FolSvp1 using the MEME (Multiple Em for Motif Elicitation) online tool. Three identified motifs and K167 were indicated. (C) Amino acid sequence alignment of motif 1 of FolSvp1 homologs from the strains in (A) using DNAMAN6.0. K167 in FolSvp1 was marked on the top of the graph. Two R variants were indicated by red circle. (D) Weblogo presentation of motif1 conservation among FolSvp1 homologs in (C). The overall height of the stack indicates the sequence conservation, and the height of symbols within the stack indicates the relative frequency of each amino acid at that position.
-
Figure 2—figure supplement 1—source data 1
Sequences used for phylogenetic tree, multi-alignments, MEME (Multiple Em for Motif Elicitation), and motif Weblogo in Figure 2—figure supplement 1.
- https://cdn.elifesciences.org/articles/82628/elife-82628-fig2-figsupp1-data1-v2.zip
-
Figure 2—figure supplement 1—source data 2
Uncropped images of multi-alignments in Figure 2—figure supplement 1C.
- https://cdn.elifesciences.org/articles/82628/elife-82628-fig2-figsupp1-data2-v2.pdf
-
Figure 2—figure supplement 1—source data 3
MEME (Multiple Em for Motif Elicitation) analysis of Fol-Secreted Virulence-related Protein1 (FolSvp1) and its homologs sequences.
- https://cdn.elifesciences.org/articles/82628/elife-82628-fig2-figsupp1-data3-v2.pdf
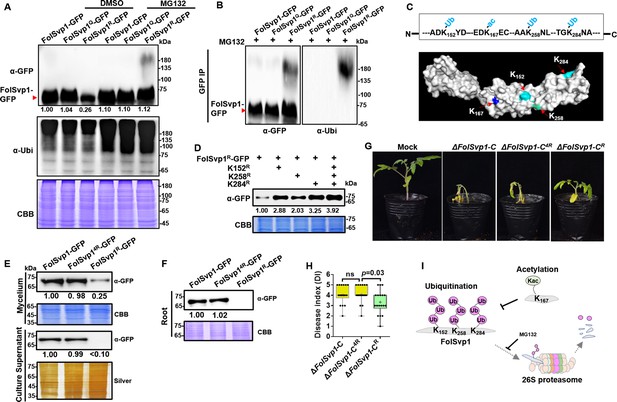
Amount and stability of wild-type (WT) and K167 mutant Fol-Secreted Virulence-related Protein1 (FolSvp1) proteins in Fol.
(A) Amount of FolSvp1-GFP, FolSvp1Q-GFP, and FolSvp1R-GFP with or without MG132 treatment. Conidia of the indicated strains were cultured in 5% liquid YEPD for 14 hr, followed by treatment with DMSO or 50 µM MG132 for 2 hr. Total proteins extracted were probed with α-GFP or anti-ubiquitin (P4D1) monoclonal antibody (α-Ubi). The amount of WT FolSvp1 treated with DMSO was set as 1. (B) Ubiquitination of FolSvp1-GFP, FolSvp1Q-GFP, and FolSvp1R-GFP. FolSvp1 proteins pulled down from the indicated strains with GFP-Trap beads were probed with α-GFP or α-Ubi. (C) Ubiquitination of K152, K258, and K284 and acetylation of K167 (up), and their distribution within FolSvp1 according to the predicted protein modeling structure (bottom). (D) Amount of K152, K258, and K284 mutant FolSvp1R proteins. The amount of native FolSvp1R was set as 1. (E) Level of FolSvp1, FolSvp1R (K167R), and FolSvp14R (simultaneous mutation of K152, K167, K258, and K284 to R) proteins in mycelia and their secreted amount. (F) Amount of the WT and mutant FolSvp1 proteins in tomato root cells. (G) Virulence of the WT and FolSvp1 mutant strains on tomato. (H) Disease index scored at 14 days after inoculation (DAI). For (E–H), amount, secretion, virulence, and disease index were determined as in Figure 2. (I) Schematic representation of K167 acetylation inhibiting ubiquitination-dependent degradation of FolSvp1 in Fol. All the experiments were performed in the presence of tomato roots. Each gel shown is a representative experiment carried out at least twice.
-
Figure 3—source data 1
Uncropped gels and blots in Figure 3.
- https://cdn.elifesciences.org/articles/82628/elife-82628-fig3-data1-v2.zip
-
Figure 3—source data 2
Statistical analysis in Figure 3.
- https://cdn.elifesciences.org/articles/82628/elife-82628-fig3-data2-v2.xlsx
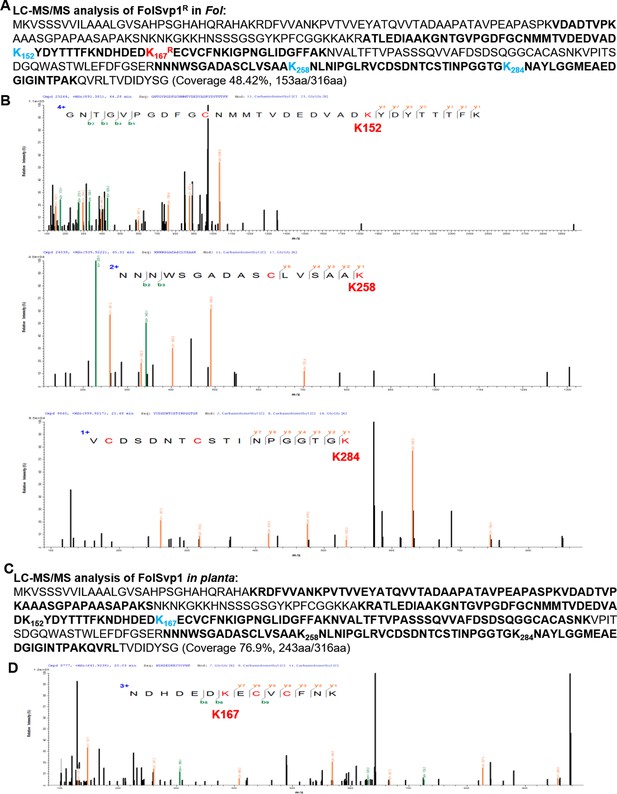
Identification of the ubiquitinated sites in Fol-Secreted Virulence-related Protein1 (FolSvp1).
(A) Liquid chromatography-tandem mass spectrometry (LC-MS/MS) identification of FolSvp1 ubiquitination in Fol. After MG132 treatment for 2 hr, the immunoprecipitated FolSvp1R-GFP was subjected to LC-MS/MS analysis. The coverage (as highlighted in bold) of FolSvp1 protein sequence was 48.42%. (B) The spectra showing FolSvp1 ubiquitination at K152, K258, and K284. (C) LC-MS/MS identification of FolSvp1 ubiquitination in planta. After MG132 treatment for 4 hr, the immunoprecipitated FolSvp1-GFP was subjected to LC-MS/MS analysis. The coverage (as highlighted in bold) of FolSvp1 protein sequence was 76.9%. For (A) and (C), the ubiquitinated residues identified were highlighted in blue. (D) The spectrum showing FolSvp1 ubiquitination at K167.
-
Figure 3—figure supplement 1—source data 1
Liquid chromatography-tandem mass spectrometry (LC-MS/MS) identification of Fol-Secreted Virulence-related Protein1 (FolSvp1) ubiquitination in Fusarium oxysporum f. sp. lycopersici (Fol).
- https://cdn.elifesciences.org/articles/82628/elife-82628-fig3-figsupp1-data1-v2.xlsx
-
Figure 3—figure supplement 1—source data 2
Liquid chromatography-tandem mass spectrometry (LC-MS/MS) identification of Fol-Secreted Virulence-related Protein1 (FolSvp1) ubiquitination in planta.
- https://cdn.elifesciences.org/articles/82628/elife-82628-fig3-figsupp1-data2-v2.xlsx
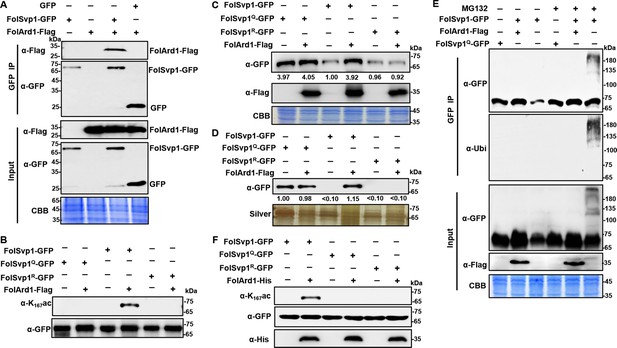
Acetylation of Fol-Secreted Virulence-related Protein1 (FolSvp1) by FolArd1.
(A) Physical interaction of FolSvp1 and FolArd1 in vivo. Co-immunoprecipitation (co-IP) assays were performed as described in Materials and methods. Proteins pulled down with GFP-Trap beads were probed with α-GFP and α-Flag, respectively (top). Input proteins were shown by Western blotting with α-GFP and α-Flag, and CBB staining (bottom). (B) Acetylation of FolSvp1-GFP, FolSvp1Q-GFP, and FolSvp1R-GFP with or without FolArd1 overexpression. Proteins pulled down with GFP-Trap beads were probed with α-GFP and α-K167ac, respectively. The same amount of FolSvp1-GFP, FolSvp1Q-GFP, and FolSvp1R-GFP was loaded to compare K167 acetylation of different samples. (C) Amount of FolSvp1-GFP, FolSvp1Q-GFP, and FolSvp1R-GFP with or without FolArd1 overexpression. Input proteins were shown by Western blotting with α-GFP and α-Flag, and CBB staining. The amount of WT FolSvp1 without FolArd1 overexpression was set as 1. (D) Amount of FolSvp1-GFP, FolSvp1Q-GFP, and FolSvp1R-GFP secreted with or without FolArd1 overexpression. Proteins pulled down from culture supernatant with GFP-Trap beads were probed with α-GFP. The amount of secreted FolSvp1Q without FolArd1 overexpression was set as 1. (E) Ubiquitination of FolSvp1-GFP and FolSvp1Q-GFP with or without FolArd1 overexpression, performed as in Figure 3. (F) FolArd1 directly acetylates FolSvp1 in vitro. FolSvp1-GFP, FolSvp1Q-GFP, or FolSvp1R-GFP pulled down with GFP-Trap beads were incubated with or without 5 μg of purified FolArd1 in the presence of 0.2 mM Ac-CoA. Products were then analyzed by immunoblotting using α-GFP, α-His, or α-K167ac. (A) was performed in the presence of tomato roots, and (B–F) were carried in the absence of tomato roots. Each gel shown is a representative experiment carried out at least twice.
-
Figure 4—source data 1
Uncropped gels and blots in Figure 4.
- https://cdn.elifesciences.org/articles/82628/elife-82628-fig4-data1-v2.zip
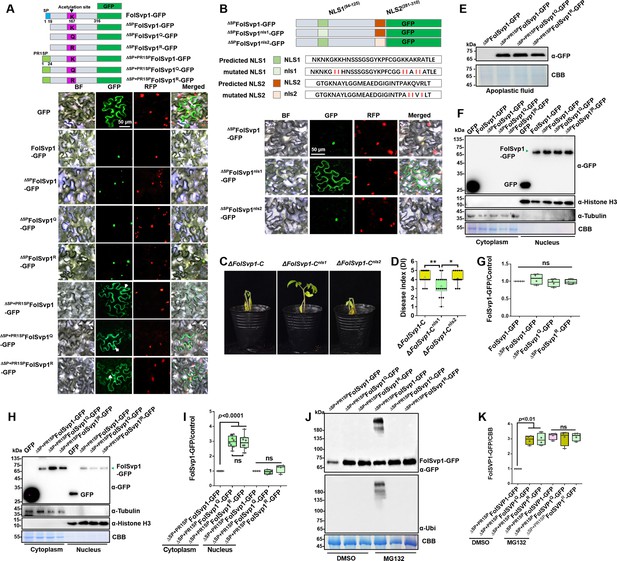
Subcellular localization and stability of Fol-Secreted Virulence-related Protein1 (FolSvp1) in planta.
(A) Subcellular localization of wild-type (WT) and K167 mutant FolSvp1-GFP proteins in tobacco leaves. ΔSP indicates removal of the native signal peptide of FolSvp1, and ΔSP + PR1SP indicates replacement of its native signal peptide with the plant PR1 signal peptide. (B) Subcellular localization of FolSvp1-GFP proteins with the native or mutant nuclear localization signal (NLS) in tobacco leaves. For (A) and (B), the indicated constructs (top) were transiently expressed in Histone H2B-RFP overexpression Nicotiana benthamiana, and images were taken at 3 days after inoculation (DAI) (bottom). Scale bars = 50 µm. (C) Virulence of the indicated strains on tomato. (D) Disease index scored at 14 DAI. For (C–D), virulence and disease index were determined as in Figure 2. (E) Amount of WT and K167 mutant FolSvp1 proteins in the apoplast of tobacco leaves. Proteins were extracted from apoplast as described in Materials and methods and probed with α-GFP. Coomassie brilliant blue (CBB) staining shows equal protein loading to each lane. (F) Subcellular fractionation of WT and K167 mutant FolSvp1-GFP proteins with or without its native signal peptide in tobacco leaves. Nuclear and cytoplasmic proteins were separately extracted and FolSvp1-GFP were detected with α-GFP. The fractionation controls were tubulin (cytoplasm), histone H3 (nucleus), and CBB staining. (G) Quantification of nuclear FolSvp1-GFP proteins relative to histone H3 in (F). Statistical significance was revealed by one-way analysis of variance (ANOVA) (mean ± SE of four independent biological replicates, p-values are shown). (H) Subcellular fractionation of WT and K167 mutant ΔSP+PR1SPFolSvp1-GFP proteins in tobacco leaves. (I) Quantification of cytoplasmic and nuclear FolSvp1-GFP proteins relative to tubulin and histone H3, respectively, in (H). Statistical significance was revealed by one-way ANOVA (mean ± SE of at least four independent biological replicates, n=8 for cytoplasm, n=4 for nucleus, p-values are shown). (J) Amount and ubiquitination of WT and K167 mutant ΔSP+PR1SPFolSvp1-GFP proteins in the cytoplasm of tobacco leaves with or without MG132 treatment. Three days after overexpression of the indicated constructs, tobacco leaves were treated with DMSO or MG132 for 4 hr and cytoplasmic proteins were extracted. Proteins pulled down with GFP-Trap beads were probed with α-GFP and α-Ubi, respectively. CBB staining shows protein loading to each lane. (K) Quantification of ΔSP+PR1SPFolSvp1-GFP proteins relative to CBB staining in (J). Statistical significance was revealed by p-value from one-way ANOVA analysis (mean ± SE of four independent biological replicates, p-values are shown).
-
Figure 5—source data 1
Uncropped gels and blots in Figure 5.
- https://cdn.elifesciences.org/articles/82628/elife-82628-fig5-data1-v2.zip
-
Figure 5—source data 2
Statistical analysis in Figure 2.
- https://cdn.elifesciences.org/articles/82628/elife-82628-fig5-data2-v2.xlsx
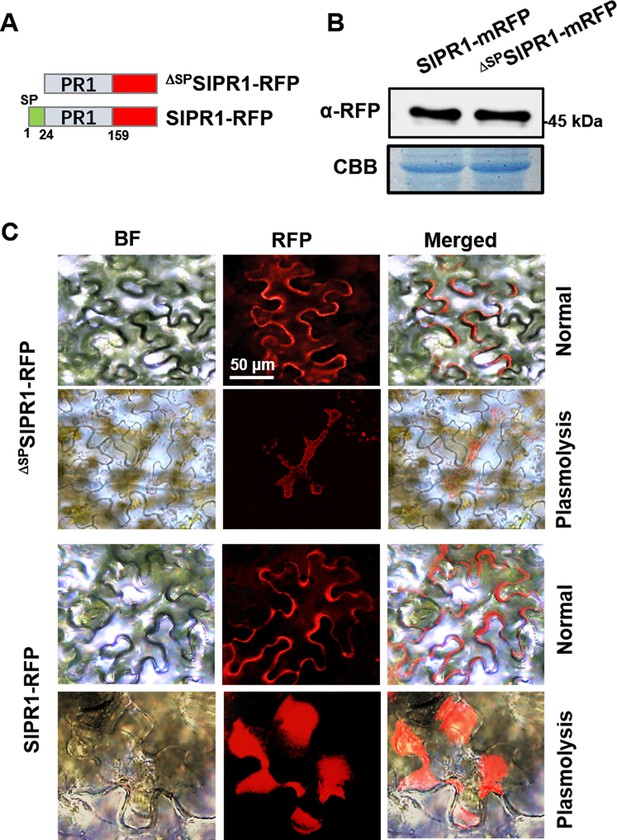
Subcellular localization of SlPR1 with or without its N-terminal signal peptide in tobacco leaves.
(A) Constructs of SlPR1-mRFP and ΔSPSlPR1-mRFP. ΔSP indicates removal of the signal peptide. (B) Western blot analysis showing the expression of SlPR1-mRFP and ΔSPSlPR1-mRFP in tobacco leaves. Total proteins extracted were probed with α-RFP. Coomassie brilliant blue (CBB) staining shows protein loading to each lane. (C) Subcellular localization of SlPR1-mRFP and ΔSPSlPR1-mRFP in tobacco leaves. Images were taken at 3 days after inoculation (DAI). The plasmolysis was visualized after treatment with 800 mM mannitol for 6 hr.
-
Figure 5—figure supplement 1—source data 1
Uncropped gels and blots in Figure 5—figure supplement 1.
- https://cdn.elifesciences.org/articles/82628/elife-82628-fig5-figsupp1-data1-v2.zip
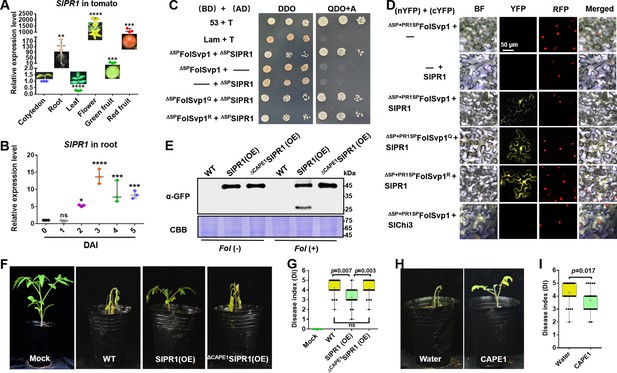
Physical interaction of Fol-Secreted Virulence-related Protein1 (FolSvp1) with SlPR1 and contribution of SlPR1 to tomato resistance.
(A) Expression profile of SlPR1 in cotyledon, root, leaves, flowers, green fruit, and red fruit measured by qRT-PCR. (B) Expression profile of SlPR1 in tomato roots after Fusarium oxysporum f. sp. lycopersici (Fol) inoculation at the indicated times measured by qRT-PCR. For (A) and (B), the expression levels were normalized to that of the SlActin. Data represent means ± SE of three independent replicates. Statistical comparisons (Student’s t-test) were presented for each variable (*p<0.05, **p<0.01, ***p<0.001, ****p<0.0001). (C) Yeast two-hybrid (Y2H) assays showing direct interaction of SlPR1 with wild-type (WT) and K167 mutant FolSvp1 proteins in vitro. T was co-transformed with p53 or Lam to represent the positive and the negative control, respectively. ΔSP indicates removal of the native signal peptide. Serial dilutions from cell suspensions of a single yeast colony were shown to represent the strength of interaction. Images were taken at 3 days after inoculation (DAI). (D) Bimolecular fluorescence complementarity (BiFC) assays showing interaction of SlPR1 with WT and K167 mutant ΔSP+PR1SPFolSvp1 proteins in vivo. The indicated constructs were transiently expressed in Histone H2B-RFP overexpression Nicotiana benthamiana, and images were taken at 3 DAI. cYFP, C-terminal region of YFP; nYFP, N-terminal region of YFP. Scale bars = 50 µm. (E) Western blot analysis showing the expression of SlPR1-GFP proteins and the generation of CAPE1-GFP with (+) or without (-) Fol infection in tomato. Total proteins extracted were probed with α-GFP. Coomassie brilliant blue (CBB) staining shows protein loading to each lane. (F) Resistance of WT and SlPR1 overexpression tomato seedlings to Fol infection. (G) Disease index scored at 14 DAI of 20 plants in (F). Statistical significance was revealed by Student’s t-test. p-Values are shown. (H) Resistance of WT tomato seedlings treated with or without CAPE1 to Fol infection. Inoculation was carried out 6 hr after application of 250 nM synthetic CAPE1 peptide to tomato roots. (I) Disease index scored at 14 DAI of 20 plants in (H). Statistical significance was revealed by Student’s t-test. p-Values are shown.
-
Figure 6—source data 1
Uncropped gels and blots in Figure 6.
- https://cdn.elifesciences.org/articles/82628/elife-82628-fig6-data1-v2.zip
-
Figure 6—source data 2
Statistical analysis in Figure 6.
- https://cdn.elifesciences.org/articles/82628/elife-82628-fig6-data2-v2.xlsx
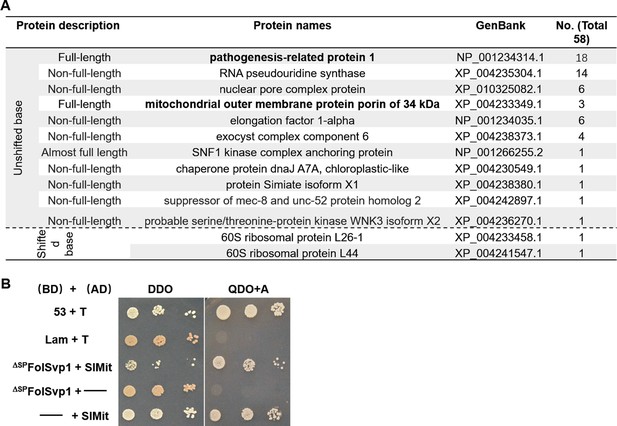
Identification of Fol-Secreted Virulence-related Protein1 (FolSvp1) interacting proteins in tomato.
(A) Annotation of 13 FolSvp1-interacting protein candidates obtained in yeast two-hybrid (Y2H) screening. (B) Self-activation activity of SlMit in yeast. The yeast transformants containing the indicated constructs were plated on DDO (SD-Leu/-Trp) and QDO/A (SD-Ade/-His/-Leu/-Trp/AbA) media and images were taken after incubation for 3 days at 28°C. T was co-transformed with p53 or Lam to represent the positive and the negative control.
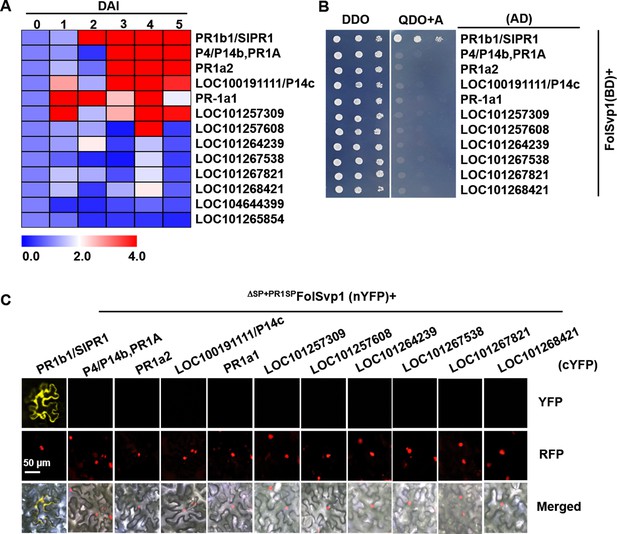
Expression profile and interaction of tomato PR1 proteins with Fol-Secreted Virulence-related Protein1 (FolSvp1).
(A) Hierarchical cluster of 13 PR1 proteins in transcript abundance in tomato roots after Fusarium oxysporum f. sp. lycopersici (Fol) inoculation at the indicated times measured by qRT-PCR. The expression levels were normalized to that of the SlActin. The color scale indicates the relative quantification of gene expression using the 2-ΔΔct method. (B) Yeast two-hybrid (Y2H) assays showing interaction of the indicated PR1 proteins with FolSvp1 in vitro. (C) Bimolecular fluorescence complementarity (BiFC) assays showing interaction of the indicated PR1 proteins with ΔSP+PR1SPFolSvp1 in vivo. Scale bars = 50 µm. (B) and (C) were performed as in Figure 6.
-
Figure 6—figure supplement 2—source data 1
qRT-PCR dataset of 13 genes used for Figure 6—figure supplement 2A.
- https://cdn.elifesciences.org/articles/82628/elife-82628-fig6-figsupp2-data1-v2.xls
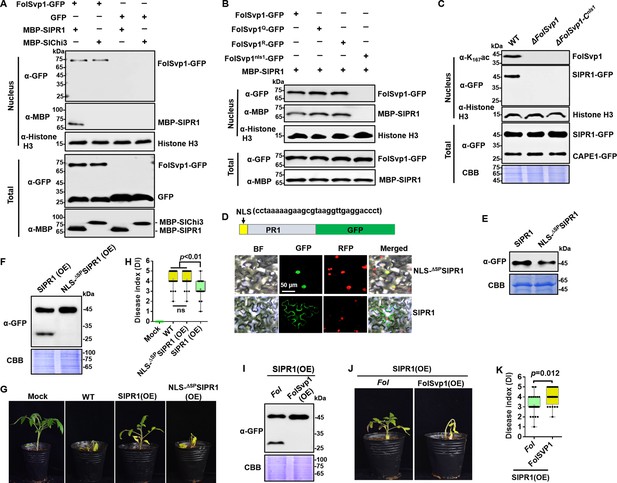
Relocation of SlPR1 into nucleus by Fol-Secreted Virulence-related Protein1 (FolSvp1) suppresses tomato resistance.
(A) Import of SlPR1 into the nucleus of tomato root protoplast by FolSvp1. (B) Import of SlPR1 into the nucleus of tomato root protoplast by wild-type (WT) and mutant FolSvp1 proteins. For (A) and (B), 50 µg purified SlPR1-MBP or SlChi3-MBP was incubated with protoplast prepared from tomato root in the presence of GFP or FolSvp1-GFP proteins pulled down from Fusarium oxysporum f. sp. lycopersici (Fol). Four hours after incubation, nuclear proteins extracted were probed with α-GFP, α-MBP, and α-histone H3, respectively (top). Input proteins were shown by Western blotting with α-GFP and α-MBP (bottom). (C) Detection of SlPR1 in the nucleus of SlPR1 overexpression tomato root infected by WT or FolSvp1 mutant strains. Nuclear proteins extracted were probed with α-K167ac, α-GFP, and α-histone H3, respectively (top). Input proteins were shown by Western blotting with α-GFP and Coomassie brilliant blue (CBB) staining (bottom). (D) Subcellular localization of SlPR1-GFP and NLS-ΔSPSlPR1-GFP in tobacco leaves. The DNA sequence for the nuclear localization signal (NLS) used here is shown in the inset. The indicated constructs were transiently expressed in Nicotiana benthamiana, and images were taken at 3 days after inoculation (DAI). Scale bars = 50 µm. (E) Western blot analysis showing the expression of SlPR1-GFP and NLS-ΔSPSlPR1-GFP in (D). Total proteins extracted were probed with α-GFP. CBB staining shows protein loading to each lane. (F) Western blot analysis showing the amount of SlPR1-GFP and CAPE1-GFP in SlPR1 and NLS-ΔSPSlPR1 overexpression tomato seedlings after Fol infection. (G) Resistance of WT and SlPR1 or NLS-ΔSPSlPR1 overexpression tomato seedlings to Fol infection. (H) Disease index scored at 14 DAI of 20 plants in (G). Statistical significance was revealed by one-way analysis of variance (ANOVA). p-Values are shown. (I) Western blot analysis showing the amount of SlPR1-GFP and CAPE1-GFP in SlPR1 overexpression tomato seedlings after infection by WT (Fol) or FolSvp1 overexpression (FolSvp1(OE)) strains. (J) Resistance of SlPR1 overexpression tomato seedlings to WT and FolSvp1 overexpression strains. (K) Disease index scored at 14 DAI of 24 plants in (J). Statistical significance was revealed by one-way ANOVA. p-Values are shown. Each gel shown is a representative experiment carried out at least twice.
-
Figure 7—source data 1
Uncropped gels and blots in Figure 7.
- https://cdn.elifesciences.org/articles/82628/elife-82628-fig7-data1-v2.zip
-
Figure 7—source data 2
Statistical analysis in Figure 7.
- https://cdn.elifesciences.org/articles/82628/elife-82628-fig7-data2-v2.xlsx
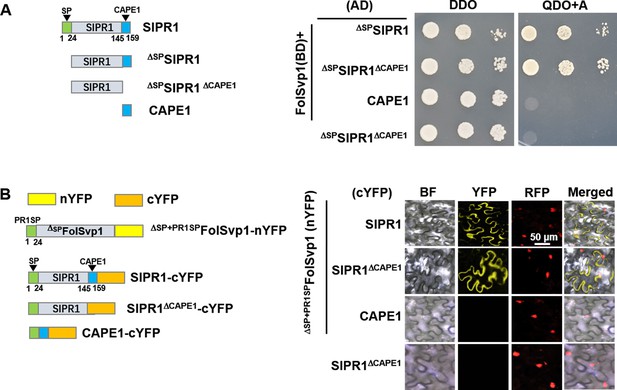
Association regions of SlPR1 with Fol-Secreted Virulence-related Protein1 (FolSvp1).
(A) Yeast two-hybrid (Y2H) assays showing interaction of the full-length or truncated SlPR1 proteins with FolSvp1 in vitro. (B) Bimolecular fluorescence complementarity (BiFC) assays showing interaction of the full-length or truncated SlPR1 proteins with ΔSP+PR1SPFolSvp1 in vivo. Scale bars = 50 µm. (A) and (B) were performed as in Figure 6.
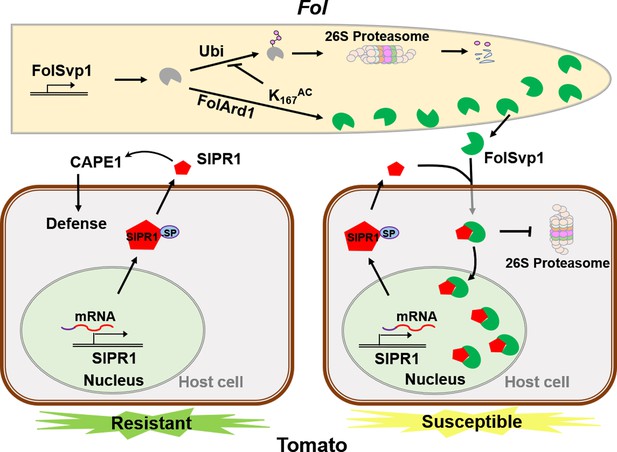
A model to explain the function of Fol-Secreted Virulence-related Protein1 (FolSvp1) in suppression of plant resistance.
During Fusarium oxysporum f. sp. lycopersici (Fol) invasion, synthesized FolSvp1 is acetylated on K167 by FolArd1. This modification represses ubiquitination-dependent degradation of FolSvp1, resulting in its secretion. K167 acetylation also prevents the degradation of FolSvp1 by the plant 26S proteasome, enabling it to translocate SlPR1 from the apoplast into plant nucleus. As a consequence, CAPE1 production is abolished, and Fol invades successfully.
Tables
Reagent type (species) or resource | Designation | Source or reference | Identifiers | Additional information |
---|---|---|---|---|
Gene (Fusarium oxysporum f. sp. lycopersic) | FolSvp1 | Fusarium genome resource | XP_018249666 | |
Gene (Fusarium oxysporum f. sp. lycopersic) | FolArd1 | Fusarium genome resource | XP_018240107 | |
Gene (Solanum lycopersicum) | SlPR1 | Solanum genome resource | P04284 | |
Gene (Solanum lycopersicum) | SlChi3 | Solanum genome resource | Z15141 | |
Genetic reagent (Nicotiana benthamiana) | RFP:H2B | Martin et al., 2009 | ||
Strain, strain background (Escherichia coli) | E. coli BL21 (DE3) | Tsingke Biotechnology | Cat. #: TSC-E05 | |
Strain, strain background(Agrobacterium tumefaciens) | LBA4404 | AngYuBio | Cat. #: AYBIO-G6038 | |
Strain, strain background (Yeast) | Y2HGold | 2nd Lab | Cat. #: YC1002 | |
Antibody | Anti-GFP (mouse monoclonal) | Abcam | Cat. #: ab183734 | 1:5000 |
Antibody | Anti-RFP (Rabbit polyclonal) | Abcam | Cat. #: ab183628 | 1:5000 |
Antibody | Anti-Flag (mouse monoclonal) | Sigma | F1804-200UG | 1:5000 |
Antibody | Anti-His (mouse monoclonal) | TransGen Biotech | Cat. #: HT501 | 1:5000 |
Antibody | Anti-MBP (mouse monoclonal) | ABclonal | Cat. #: AE016 | 1:5000 |
Antibody | Anti-Histone H3 (Rabbit polyclonal) | Abcam | Cat. #: ab1791 | 1:5000 |
Antibody | Anti-Actin (mouse monoclonal) | Affinity | Cat. #: AF7018 | 1:5000 |
Antibody | Anti-Tubulin (Rabbit polyclonal) | PTM Biolabs | Cat. #: PTM-1011 | 1:2500 |
Antibody | Anti-ubiquitination (mouse monoclonal) | Santa Cruz | Cat. #: sc-8017 | 1:2500 |
Antibody | Anti-K167ac (Rabbit polyclonal) | HUABIO | Materials and methods | 1:10,000 |
Recombinant DNA reagent | pTX041 (plasmid) | Deng et al., 2018 | ||
Recombinant DNA reagent | pQB-V3 (plasmid) | Chen et al., 2020 | ||
Recombinant DNA reagent | pK7FWG2 (plasmid) | Karimi et al., 2002 | ||
Recombinant DNA reagent | pGWB554 (plasmid) | Nakagawa et al., 2007 | ||
Recombinant DNA reagent | pEarleyGate201-YN (plasmid) | Chen et al., 2020 | ||
Recombinant DNA reagent | pEarleyGate202-YC (plasmid) | Chen et al., 2020 | ||
Sequence-based reagent | Primers | This paper | Supplementary file 1c | |
Sequence-based reagent | Synthetic genes | This paper | Supplementary file 1d | |
Commercial assay or kit | ClonExpress II One Step Cloning Kit | Vazyme | Cat. #: C112-01/02 | |
Commercial assay or kit | Nuclear Extraction Kit | Solarbio | Cat. #: R0050 | |
Commercial assay or kit | PrimeScriptTM RT reagent Kit | Takara | Cat. #: RR037A | |
Commercial assay or kit | SYBR Premix Ex Taq | Mei5bio | Cat. #: MF013-plus-10 | |
Commercial assay or kit | Omni-Easy Instant BCA Protein Assay Kit | Epizyme | Cat. #: ZJ102 | |
Chemical compound, drug | Protease inhibitor mixture | Solarbio | Cat. #: P6730 | |
Chemical compound, drug | Proteinase inhibitor cocktail | Sigma | Cat. #: P9599-5ML | |
Chemical compound, drug | Anti-GFP agarose | KT Health | Cat. #: KTSM1301 | |
Chemical compound, drug | Hygromycin B | Thermo Fisher Scientific | Cat. #: 10687010 | |
Chemical compound, drug | G418 | Solarbio | Cat. #: IG0010 | |
Chemical compound, drug | DAPI | Sigma | Cat. #: D9542 | |
Software, algorithm | ImageJ software | ImageJ(http://imagej.nih.gov/ij/) | Version 1.48 | |
Software, algorithm | GraphPad Prism software | GraphPad Prism (https://graphpad.com) | Version 6.0.0 |
Additional files
-
Supplementary file 1
Mass spectrum data, primers and synthesized genes used in this study.
(a) Target proteins obtained from the Fol-Secreted Virulence-related Protein1 (FolSvp1)-GFP co-purified liquid chromatography-tandem mass spectrometry (LC-MS/MS) data. (b) Target proteins obtained from the GFP co-purified LC-MS/MS data. (c) Primers used in this study. (d) Synthesized genes used in yeast two-hybrid (Y2H) study.
- https://cdn.elifesciences.org/articles/82628/elife-82628-supp1-v2.xlsx
-
MDAR checklist
- https://cdn.elifesciences.org/articles/82628/elife-82628-mdarchecklist1-v2.pdf