An armoured marine reptile from the Early Triassic of South China and its phylogenetic and evolutionary implications
Abstract
Sauropterygia was a taxonomically and ecomorphologically diverse clade of Mesozoic marine reptiles spanning the Early Triassic to the Late Cretaceous. Sauropterygians are traditionally divided into two groups representing two markedly different body plans – the short-necked, durophagous Placodontia and the long-necked Eosauropterygia – whereas Saurosphargidae, a small clade of armoured marine reptiles, is generally considered as the sauropterygian sister-group. However, the early evolutionary history of sauropterygians and their phylogenetic relationships with other groups within Diapsida are still incompletely understood. Here, we report a new saurosphargid from the Early Triassic (Olenekian) of South China – Prosaurosphargis yingzishanensis gen. et sp. nov. – representing the earliest known occurrence of the clade. An updated phylogenetic analysis focussing on the interrelationships among diapsid reptiles recovers saurosphargids as nested within sauropterygians, forming a clade with eosauropterygians to the exclusion of placodonts. Furthermore, a clade comprising Eusaurosphargis and Palatodonta is recovered as the sauropterygian sister-group within Sauropterygomorpha tax. nov. The phylogenetic position of several Early and Middle Triassic sauropterygians of previously uncertain phylogenetic affinity, such as Atopodentatus, Hanosaurus, Majiashanosaurus, and Corosaurus, is also clarified, elucidating the early evolutionary assembly of the sauropterygian body plan. Finally, our phylogenetic analysis supports the placement of Testudines and Archosauromorpha within Archelosauria, a result strongly corroborated by molecular data, but only recently recovered in a phylogenetic analysis using a morphology-only dataset. Our study provides evidence for the rapid diversification of sauropterygians in the aftermath of the Permo-Triassic mass extinction event and emphasises the importance of broad taxonomic sampling in reconstructing phylogenetic relationships among extinct taxa.
Editor's evaluation
This significant new study is built around a remarkable fossil of a new genus and species of armoured marine reptile from the Early Triassic of China. More importantly, this paper also adds to our understanding of the diversification of reptiles during the Triassic and also sheds light on the interrelationships of a wide range of important groups. The authors present a solid phylogenetic analysis of sauropterygians, which reveals a possible new clade, Sauropterygomorpha, which is recovered close to Archosauromorpha. The authors suggest that the latter, as well as the Testudines and Ichthyosauromorpha, belong to a clade that had previously only been recovered using phylogenomic data, the Archelosauria. This paper will be of interest to a broad range of scientists, including palaeontologists, herpetolgists, and evolutionary biologists.
https://doi.org/10.7554/eLife.83163.sa0eLife digest
Around 252 million years ago, just before the start of a period of time known as the Triassic, over 90% of animals, plants and other species on Earth went extinct in what was the worst mass extinction event in the planet’s history. It is thought to have happened because of an increase in volcanic eruptions that led to global warming, acid rain and other catastrophic changes in the environment.
The loss of so many species caused ecosystems to restructure as the surviving species evolved to fill niches left by those that had gone extinct. On land, reptiles diversified to give rise to dinosaurs, the flying pterosaurs, and the ancestors of modern crocodiles, lizards, snakes and turtles. Some of these land-based animals evolved to live in water, resulting in many species of marine reptiles emerging during the Triassic period.
This included the saurosphargids, a group of marine reptiles that lived in the Middle Triassic around 247–237 million years ago. They were ‘armoured’ with a shield made of broadened ribs superficially similar to that of turtles, and a covering of bony plates. However, it is unclear how the saurosphargids evolved and how closely they are related to other marine reptiles.
Here, Wolniewicz et al. studied a new species of saurosphargid named Prosaurosphargis yingzishanensis that was found fossilized in a quarry in South China. The animal was around 1.5 metres long and had a chest shield and armoured plates like other saurosphargids. The characteristics of the rock surrounding the fossil suggest that this individual lived in the Early Triassic, several million years before other saurosphargid species.
The team used a phylogenetic approach to infer the evolutionary relationships between P. yingzishanensis and numerous other land-based and marine reptiles based on over 220 anatomical characteristics of the animals. The resulting evolutionary tree indicated that the saurosphargids represented an early stage in the evolution of a larger group of marine reptiles known as the sauropterygians. The analysis also identified the closest land-based relatives of sauropterygians.
These findings provide evidence that marine reptiles rapidly diversified in the aftermath of the mass extinction event 252 million years ago. Furthermore, they contribute to our understanding of how ecosystems recover after a major environmental crisis.
Introduction
Several groups of reptiles invaded the marine realm in the aftermath of the Permo-Triassic mass extinction (PTME), the largest extinction event in Earth’s history (Benton, 2015). This phenomenon was likely a result of the scarcity of marine competitors and predators caused by the PTME and high productivity in the incipient shallow marine environment (Vermeij and Motani, 2018). Triassic marine reptiles, including the iconic Ichthyosauromorpha and Sauropterygia, as well as some other smaller and lesser known groups, achieved high taxonomic and ecological diversity rapidly after their emergence in the late Early Triassic and played a pivotal role in the reorganisation of marine food webs following the PTME (Scheyer et al., 2014; Kelley and Pyenson, 2015; Motani et al., 2015; Motani et al., 2017; Jiang et al., 2016; Stubbs and Benton, 2016; Cheng et al., 2019; Moon and Stubbs, 2020; Li and Liu, 2020; Sander et al., 2021; Qiao et al., 2022). Because Mesozoic marine reptiles represent likely several independent transitions from a terrestrial to an aquatic lifestyle, they also provide an ideal system to analyse the roles of function and constraint in determining evolutionary pathways (Motani, 2009; Benson, 2013).
Sauropterygia was a diverse clade of Mesozoic marine reptiles that first appeared in the late Early Triassic (Jiang et al., 2014; Li and Liu, 2020) and its members remained important predators in marine ecosystems until their extinction at the end of the Late Cretaceous (Bardet, 1994; Rieppel, 2000a; Benson and Druckenmiller, 2014). Sauropterygia is traditionally divided into two major lineages, representing two markedly different body plans – the Placodontia and the Eosauropterygia, the latter comprising Pachypleurosauria, Nothosauroidae and Pistosauroidea (which includes the iconic Plesiosauria; Rieppel, 2000a). Placodonts were characterised by the presence of short necks and short skulls and possessed crushing palatal dentition almost certainly used for feeding on hard-shelled invertebrates (Rieppel, 2002; Crofts et al., 2017). Some early-diverging placodonts had a limited covering of osteoderms on their backs and possibly limbs (Jiang et al., 2008; Klein and Scheyer, 2013), but derived forms evolved extensive dorsal armour superficially similar to that of turtles (Scheyer, 2007; Wang et al., 2020). Eosauropterygians, on the other hand, had elongated necks and elongated skulls with pointed dentition suitable for capturing fast-moving prey (Rieppel, 2000a; Rieppel, 2002). Placodonts remained restricted to shallow marine environments until their extinction in the Late Triassic, whereas eosauropterygians evolved a suite of adaptations for a pelagic lifestyle and became one of the dominant groups of marine reptiles in the Jurassic and Cretaceous (Kelley et al., 2014).
Even though sauropterygians have a rich fossil record and a long history of scientific research, the early evolution of the group is still incompletely understood. In a broad phylogenetic context, sauropterygians have been consistently recovered within Diapsida, but their exact phylogenetic position relative to other diapsid groups remains unresolved. Several diapsid clades, including Saurosphargidae (Li et al., 2014; Li et al., 2018; Neenan et al., 2015; Wang et al., 2022), Testudines (deBraga and Rieppel, 1997; Schoch and Sues, 2015), Ichthyosauromorpha (Martínez et al., 2021), Thalattosauria (Simões et al., 2022) and the armoured reptile Eusaurosphargis dalsassoi (Scheyer et al., 2017) were previously proposed to be the sauropterygian sister-group, but its exact identity is still a matter of debate. Because sauropterygians were suggested as being closely related not only to turtles, but also to archosauromorphs (Chen et al., 2014a; Neenan et al., 2015; Martínez et al., 2021; Simões et al., 2022), resolving their phylogenetic placement within diapsids is of crucial importance for solving the phylogenetic uncertainty surrounding Archelosauria – a clade comprising turtles and archosauromorphs strongly supported by molecular data (Crawford et al., 2015; Lyson and Bever, 2020), but only recently recovered in a phylogenetic analysis using a morphology-only dataset (Simões et al., 2022).
Saurosphargids are a small clade of Mesozoic marine reptiles characterised by the presence of body armour comprising broadened dorsal ribs, forming a closed ‘rib-basket’, and a moderately- to well-developed osteoderm covering (Li et al., 2011; Li et al., 2014). Saurosphargids are known from the Middle Triassic of Europe and South China, although recent evidence suggests they could have survived as late as the Late Triassic (Scheyer et al., 2022). Saurosphargids comprise as many as four taxa – Saurosphargis volzi (considered a nomen dubium by some authors) (Huene, 1936; Nosotti and Rieppel, 2003; Scheyer et al., 2017), the heavily armoured Sinosaurosphargis yunguiensis (Li et al., 2011; Hirasawa et al., 2013), and two species in the genus Largocephalosaurus (L. polycarpon and L. qianensis; Cheng et al., 2012; Li et al., 2014). Saurosphargids are one of the reptile groups proposed as the sister-group of sauropterygians (see above), but some recent phylogenetic analyses have suggested their placement within sauropterygians instead, as either the sister-group to placodonts (Schoch and Sues, 2015; Simões et al., 2018; Martínez et al., 2021) or eosauropterygians (Scheyer et al., 2017; Wang et al., 2019; Simões et al., 2022).
Our understanding of the early evolution of the sauropterygian body plan is also hindered by the uncertain phylogenetic position of several Early and Middle Triassic taxa. Hanosaurus hupehensis (Young, 1972; Rieppel, 1998a; Wang et al., 2022) and Majiashanosaurus discocoracoidis (Jiang et al., 2014) from the Early Triassic of South China are variably recovered as either lying outside of the clade comprising Saurosphargidae + Sauropterygia (Hanosaurus; Wang et al., 2022), as pachypleurosaurs (Jiang et al., 2014; Neenan et al., 2015; Lin et al., 2021; Wang et al., 2022), or as outgroups to a clade comprising nothosauroids and pachypleurosaurs to the exclusion of pistosauroids (Li and Liu, 2020). The phylogenetic placement of Corosaurus alcovensis from the Early–Middle Triassic of Wyoming, USA, is also unresolved, with different authors arguing for its early-diverging eosauropterygian (Rieppel, 1994; Li and Liu, 2020), eusauropterygian (more closely related to nothosauroids and pistosauroids than to pachypleurosaurs; Neenan et al., 2015; Lin et al., 2021), or pistosauroid (Rieppel, 1998b; Wang et al., 2022) affinity. The phylogenetic position of the herbivorous hammer-headed sauropterygian Atopodentatus unicus from the Middle Triassic of South China relative to placodonts and eosauropterygians also remains uncertain (Cheng et al., 2014; Li et al., 2016; Wang et al., 2022). These conflicting phylogenetic placements are likely the result of inadequate sampling of Early and Middle Triassic sauropterygian ingroup taxa, as well as the inclusion of only a limited number of diapsid reptile clades, including potential sauropterygian outgroups, in previous phylogenetic analyses.
Here, we report an Early Triassic saurosphargid from South China, representing the earliest recorded occurence of the group. We also present an updated phylogenetic hypothesis for Diapsida with a particular focus on Sauropterygia, which we use as context for discussing the early evolutionary assembly of the sauropterygian body plan.
Results
Geological background
Yingzishan quarry, where the new specimen (HFUT YZSB-19-109) was collected, is located on the northern boundary of the Yangtze Platform (Figure 1A; Li and Liu, 2020). The new specimen represents the Early Triassic Nanzhang-Yuan’an fauna (Li and Liu, 2020) and originates from the upper part of the third member of the Jialingjiang Formation (Figure 1B). Traditionally, the Jialingjiang Formation has been divided into four members by most authors studying the region (Li et al., 2002; Zhao et al., 2008; Cheng et al., 2015). From base to top, the first member consists of thick-bedded to massive dolostone, the second member consists of vermicular limestone intercalated with dolostone, the third member consists of laminated thin- to medium-bedded limestone to dolostone, whereas the fourth member is composed of dolostone and karstified breccia. Thus, in the four-member division of the Jialingjiang Formation, the thick volcanic ash (Figure 1B) marks the bottom of the fourth member. However, recent geological mapping in the region (Chen et al., 2016a) proposed that the fourth member of the Jialingjiang Formation should be included in the Middle Triassic Badong Formation, indicating a three-member division of the Jialingjiang Formation. This division is consistent with the lithology of the Jianlingjiang and Badong formations as defined by the official geological guide of Hubei Province (Bureau of Geology and Mineral Resources of Hubei Province, 1990). The division of the Lower and Middle Triassic in the region is also consistent with the widespread thick volcanic ash as a marker of the Lower–Middle Triassic boundary in the Yangtze Platform (Chen et al., 2020). Consequently, this three-member division was followed by Cheng et al., 2019, Li and Liu, 2020, Qiao et al., 2019 and is also followed in this study.
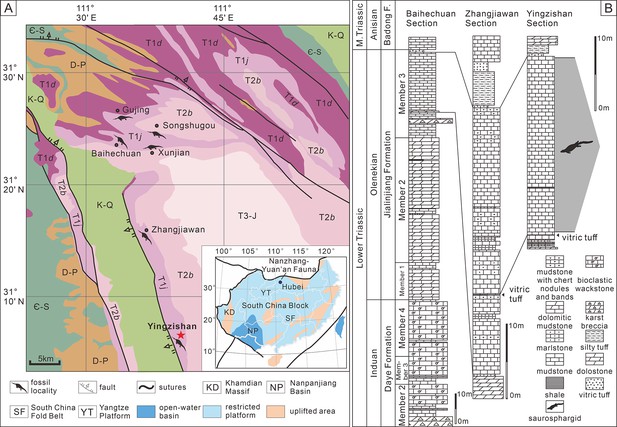
Locality and horizon of Prosaurosphargis yingzishanensis (HFUT YZSB-19-109).
(A) The geological map of the Nanzhang-Yuan’an region showing Yingzishan quarry, where HFUT YZSB-19-109 was collected; inset is a paleogeographic map of the South China Block in the Triassic showing the location of the Nanzhang-Yuan’an fauna (after Qiao et al., 2019). (B) Stratigraphic column showing the horizon from which HFUT YZSB-19-109 was collected. Abbreviations: Є-S, Cambrian–Silurian; D-P, Devonian–Permian; F., Formation; K-Q, Cretaceous–Quaternary; M., Middle; T1d, Daye Formation, Lower Triassic; T1j, Jialingjiang Formation, Lower Triassic; T2b, Badong Formation, Middle Triassic; T3-J, Upper Triassic–Jurassic.
However, the second and third members of the Jialingjiang Formation were recently redefined by Yan et al., 2021, who regarded the lowermost base of the thick vitric tuff as the boundary between the second and third members (contra Chen et al., 2016a; Cheng et al., 2019). As a consequence, the Nanzhang-Yuan’an fauna was placed into the newly defined second member of the Jialingjiang Formation (Cheng et al., 2022; Zhao et al., 2022). We argue that this new definition and division of different members of the Jialingjiang Formation contradicts the official geological guide (Bureau of Geology and Mineral Resources of Hubei Province, 1990) and causes confusion. Therefore, we prefer to maintain the definition of the thick vitric tuff as the boundary between the Early Triassic Jialingjiang and the Middle Triassic Badong formations in the Nanzhang-Yuan’an region (Figure 1B), pending future updates of the official geological guide of Hubei Province.
The new specimen is buried in dark grey, laminated, and thin-bedded carbonate mudstone (Figure 1B) with some carbonaceous interactions. There are also some peloids, replacive dolomites, and microbial mats in the fossiliferous levels (Chen et al., 2022). Based on the published sedimentological accounts (Wang et al., 2011; Chen et al., 2016b; Yan et al., 2018; Yan et al., 2021; Li et al., 2020; Zhao et al., 2022) and field observations, a restricted, stagnant, and hypersaline lagoon within a tidal flat environment is inferred as the burial setting of the marine reptiles of the Nanzhang-Yuan’an fauna (Chen et al., 2022).
Systematic palaeontology
Reptilia Laurenti, 1768
Diapsida Osborn, 1903
Archelosauria Crawford et al., 2015
Sauropterygomorpha tax. nov.
Definition: The most recent common ancestor of Eusaurosphargis dalsassoi and Pistosaurus longaevus, and all of its descendants (min ∇ Eusaurosphargis dalsassoi Nosotti and Rieppel, 2003 & Pistosaurus longaevus Meyer, 1839).
Diagnosis: Osteoderms present (ch. 143.1), body strongly flattenned dorso-ventrally (ch. 144.1), clavicle applied to the medial surface of scapula (ch. 148.1), metatarsal V long and slender (ch. 186.0), metatarsal I less than 50% the length of metatarsal IV (ch. 189.1).
Sauropterygia Owen, 1860
Saurosphargidae Li et al., 2011
Prosaurosphargis yingzishanensis gen. et sp. nov.
urn:lsid:zoobank.org:act:36BED757-A86D-4951-B664-A91969F7CDBF urn:lsid:zoobank.org:act:825E86DD-E636-4A99-AA85-7AF4DBCD7E8B
Holotype: HFUT YZSB-19-109, a partial postcranial skeleton (Figure 2). The specimen is housed in the Geological Museum of Hefei University of Technology, Hefei, Anhui Province, China (HFUT).
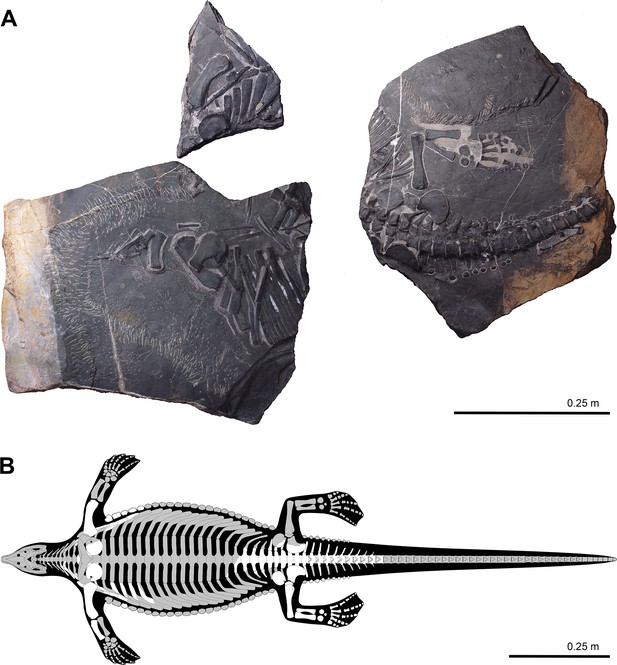
Holotype of Prosaurosphargis yingzishanensis (HFUT YZSB-19-109) (A) and skeletal reconstruction with known elements highlighted in white (gastralia removed for clarity) (B).
Etymology: Genus name from the Greek preposition πρό (pró), meaning before, and Saurosphargis, the name of the type genus of the family Saurosphargidae (Li et al., 2011). The specific epithet refers to the type locality (see above).
Horizon and locality: Third Member of the Jialingjiang Formation (uppermost Spathian, Olenekian, Lower Triassic), Yingzishan quarry, Yuan’an County, Hubei Province, China.
Diagnosis: A saurosphargid characterised by the following combination of character states: (1) spaces between dorsal transverse processes anteroposteriorly shorter than the anteroposterior widths of the transverse processes (like in Saurosphargis and Sinosaurosphargis, but different from Largocephalosaurus, in which the spaces between the dorsal transverse processes are wider than the transverse processes); (2) ribs without uncinate processes (like in Sinosaurosphargis, but unlike in Saurosphargis and Largocephalosaurus, in which uncinate processes are present); (3) osteoderms forming a median, parasaggital and lateral rows (similar to Largocephalosaurus polycarpon, but different from L. qianensis, in which additional, small osteoderms more extensively cover the lateral sides of the body, and different from Sinosaurosphargis, in which the osteoderms form an extensive dorsal armour); (4) ectepicondylar groove on humerus present (like in Largocephalosaurus qianensis, absent in L. polycarpon); (5) entepicondylar foramen in humerus absent (as in Largocephalosaurus) (details of humerus morphology unknown in Sinosaurosphargis); (6) radius short relative to humerus compared with other saurosphargids; (7) presence of a single distal tarsal (distal tarsal IV) (different from Largocephalosaurus, which possesses two distal tarsals – III and IV) (number of tarsals unknown in Sinosaurosphargis); (8) anterior caudal ribs shorter than sacral ribs (anterior caudal ribs longer than sacral ribs in Largocephalosaurus, unknown in Sinosaurosphargis).
Description and comparisons
HFUT YZSB-19-109 comprises three blocks (Figure 2). The first large block contains mostly disarticulated parts from the anterior right portion of the postcranial skeleton – 2 cervical neural arches and 5 cervical ribs, 1 dorsal centrum and 2 dorsal neural arches (all three preserved in articulation), 9 dorsal ribs, 1 median, 11 lateral and 13 lateralmost gastral elements, a single parasaggital osteoderm, the right scapula, right coracoid, right humerus, and right radius (Figure 3). The second small block contains the distal ends of the six most anterior dorsal ribs, five lateralmost gastral elements, as many as eight osteoderms, a partial left coracoid, and a left humerus (Figure 4). The third large block preserves the articulated posterior part of the body, including 5 posterior dorsal neural arches and associated ribs, 4 posterior dorsal/sacral vertebrae and ribs, an articulated series of 11 anterior caudals with associated ribs, chevrons and osteoderms, 14 lateralmost gastralia, a single parasaggital osteoderm, a partial right and a complete left pelvic girdle, and a complete left hindlimb (Figures 5 and 6). Based on the humerus:total body length and femur:total body length ratios of the type specimen of Largocephalosaurus qianensis (specimen IVPP V 15638, total body length = 2317 mm; Li et al., 2014), the only completely preserved saurosphargid specimen discovered to date, the total length of HFUT YZSB-19-109 is estimated to have reached between 1468 mm to 1583 mm, respectively. Selected measurements of HFUT YZSB-19-109 are given in Table 1.
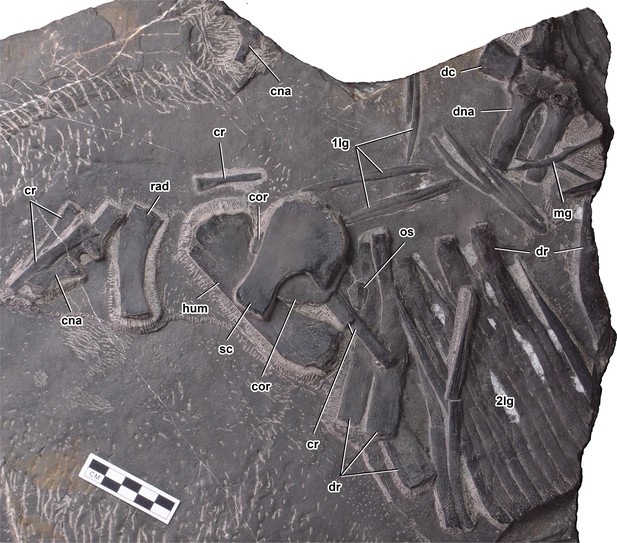
Anterior vertebral column, right pectoral girdle, and right forelimb elements of Prosaurosphargis yingzishanensis.
Abbreviations: 1lg, first lateral gastral element; 2lg, second lateral gastral element; cna, cervical neural arch; cor, coracoid; cr, cervical rib; dc, dorsal centrum; dna, dorsal neural arch; dr, dorsal rib; hum, humerus; mg, median gastral element; os, osteoderm; rad, radius; sc, scapula. Scale bar = 5 cm.
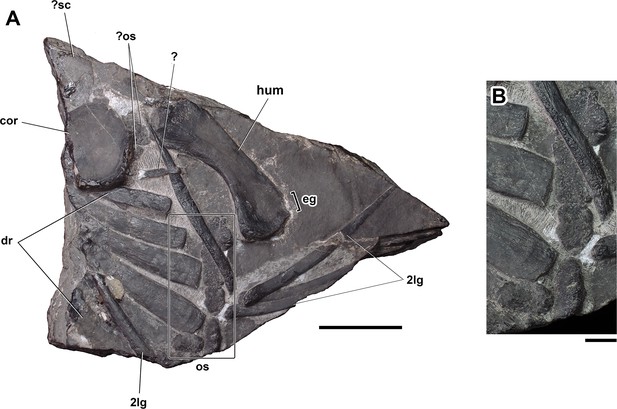
Dorsal ribs, osteoderms, coracoid, and humerus from the left side of the body of Prosaurosphargis yingzishanensis (A) and detail of lateral osteoderms (B).
Abbreviations: 2lg, second lateral gastral element; cor, coracoid; dr, dorsal rib; eg, ectepicondylar groove; hum, humerus; os, osteoderm, ?sc, ?scapula. Scale bar = 5 cm in (A) and 1 cm in (B).
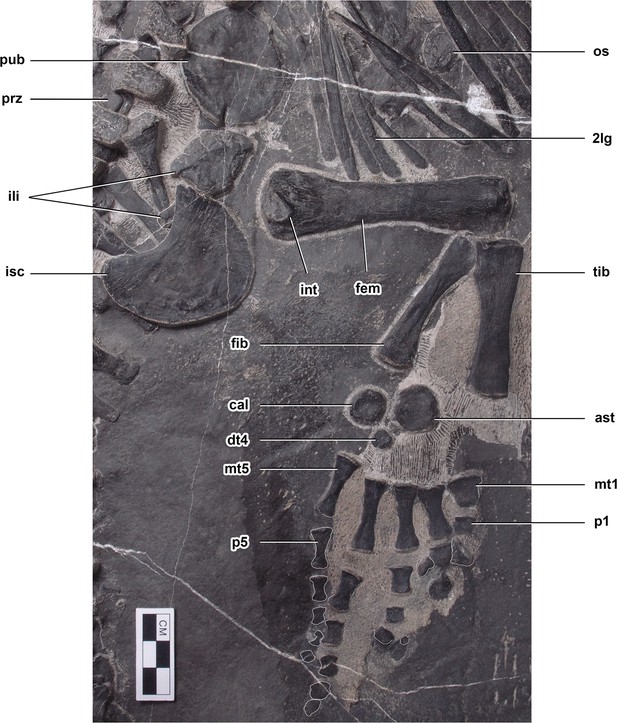
Pelvic girdle and hindlimb of Prosaurosphargis yingzishanensis.
Abbreviations: 2lg, second lateral gastral element; ast, astragalus; cal, calcaneum; dt4, distal tarsal IV; fem, femur; fib, fibula; ili, ilium; int, internal trochanter; isc, ischium; mt1, metatarsal I; mt5, metatarsal V; os, osteoderm; p1, first phalanx of digit 1; p5, first phalanx of digit 5; pub, pubis; prz, prezygapophysis; tib, tibia. Scale bar = 3 cm.
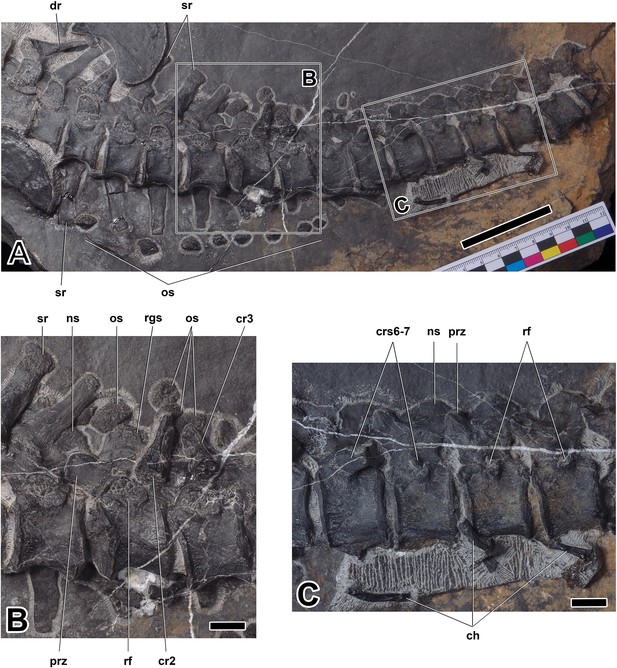
Sacral and caudal vertebrae of Prosaurosphargis yingzishanensis (A), close-up of anterior caudal vertebrae (B), and close-up of more posterior caudal vertebrae (C).
Abbreviations: ch, chevron; cr2, second caudal rib; cr3, third caudal rib; crs6-7, sixth and seventh caudal ribs; dr, dorsal rib; ns, neural spine; os, osteoderm; prz, prezygapophysis; rf, rib facet; rgs, rugose surface; sr, sacral rib. Scale bar = 5 cm in (A) and 1 cm in (B) and (C).
Selected measurements of HFUT YZSB-19-109, holotype of Prosaurosphargis yingzishanensis.
Vertebral column | |
---|---|
Centrum position | Maximum anteroposterior length(measured along mid-dorsoventral height) |
Last dorsal | 18.25 mm |
1st sacral | 19.45 mm |
2nd sacral | 20.49 mm |
3rd sacral | 20.50 mm |
1st caudal | 20.50 mm |
2nd caudal | 18.35 mm |
3rd caudal | 19.40 mm |
4th caudal | 20.14 mm |
5th caudal | 18.64 mm |
6th caudal | 19.10 mm |
7th caudal | 17.95 mm |
8th caudal | 18.84 mm |
9th caudal | 18.10 mm |
10th caudal | 17.86 mm |
Pectoral girdle | |
Right scapula | |
Maximum proximodistal length | 68.09 mm |
Maximum proximal width | 51.20 mm |
Right coracoid | |
Maximum proximodistal length | 51.65 mm |
Left coracoid | |
Maximum proximodistal length | 52.25 mm |
Maximum anteroposterior length | 39.40 mm |
Forelimb | |
Right humerus | |
Maximum proximodistal length | 104.44 mm |
Maximum proximal width | 38.85 mm |
Maximum distal width | 27.80 mm |
Left humerus | |
Maximum anteroposterior length | 104.24 mm |
Minimum mediolateral width | 20.10 mm |
Maximum distal width | 31.50 mm |
Right radius | |
Maximum proximodistal length (measured along proximodistal axis) | 58.50 mm |
Maximum proximodistal length (measured along anterior margin) | 60.95 mm |
Maximum proximodistal length (measured along posterior margin) | 51.10 mm |
Pelvic girdle | |
Left ilium | |
Maximum proximodistal length | 23.20 mm |
Maximum distal width | 32.99 mm |
Left ischium | |
Maximum anteroposterior (proximodistal) length | 51.44 mm |
Maximum mediolateral width | 36.59 mm |
Left pubis | |
Maximum proximodistal length | 43.25 mm |
Maximum anteroposterior length | 36.85 mm |
Hindlimb | |
Left femur | |
Maximum proximodistal length | 93.85 mm |
Maximum proximal width | 27.65 mm |
Maximum distal width | 23.40 mm |
Minimum mediolateral (anteroposterior) width | 14.25 mm |
Left tibia | |
Maximum proximodistal length | 60.35 mm |
Maximum proximal width | 18.29 mm |
Maximum distal width | 16.39 mm |
Minimum mediolateral (anteroposterior) width | 10.90 mm |
Left fibula | |
Maximum proximodistal length | 55.59 mm |
Maximum proximal width | 12.90 mm |
Maximum distal width | 15.24 mm |
Minimum mediolateral (anteroposterior) width | 7.25 mm |
Astragalus | |
Maximum proximodistal length | 18.45 mm |
Maximum mediolateral (anteroposterior) width | 18.20 mm |
Calcaneum | |
Maximum proximodistal length | 14.25 mm |
Maximum mediolateral (anteroposterior) width | 13.55 mm |
Distal tarsal IV | |
Maximum proximodistal length | 9.49 mm |
Maximum mediolateral (anteroposterior) width | 8.95 mm |
Metacarpals | |
Metacarpal I maximum proximodistal length | 13.44 mm |
Metacarpal II maximum proximodistal length | 20.89 mm |
Metacarpal III maximum proximodistal length | 25.89 mm |
Metacarpal IV maximum proximodistal length | 28.19 mm |
Metacarpal V maximum proximodistal length | 24.25 mm |
Axial skeleton
Vertebrae: The vertebral column of HFUT YZSB-19-109 is represented by two disarticulated cervical neural arches, a single anterior dorsal centrum and two anterior dorsal neural arches (all three preserved in articulation), and an articulated series of 20 vertebrae comprising five posterior dorsal neural arches, four complete posterior dorsal/sacral vertebrae and 11 complete caudal vertebrae.
Two cervical neural arches are preserved in HFUT YZSB-19-109 (Figure 3). Only the left side of a small neural arch, representing one of the anterior post-axial cervicals, is preserved in dorsal view, whereas a larger neural arch, belonging to one of the posterior cervicals, is completely preserved and exposed in ventral view, but it is partially obscured by an overlying cervical rib and is rotated by approximately 180 degrees, so that its anterior end faces posteriorly. The transverse processes of the cervical neural arches extend posterolaterally and are proportionally shorter than the transverse processes of the dorsal neural arches. A well-developed prezygapophysis is clearly visible in the smaller neural arch. Well-developed postzygapophyses are preserved in both the anterior and posterior cervical neural arches, where they are visible protruding from just underneath the overlying cervical rib in the latter. Approximately oval, anteroposteriorly elongate facets for articulation with the centrum are exposed in the posterior cervical neural arch.
The only preserved dorsal centrum is exposed in ventral view (Figure 3). It is mediolaterally constricted at its anteroposterior mid-length, resembling the dorsal centra of other saurosphargids (Huene, 1936; Li et al., 2011; Li et al., 2014). The pedicels of the dorsal neural arches are anteroposteriorly elongate and mediolaterally narrow, resembling those preserved in Saurosphargis (Huene, 1936). The transverse processes are straight and mediolaterally elongate. The maximum width of the transverse process is greater than the maximum width of the space between the transverse processes of adjacent neural arches (Figure 3), a condition similar to that exhibited by Saurosphargis (Huene, 1936) and Sinosaurosphargis (Li et al., 2011), but different from Largocephalosaurus (Li et al., 2014) and Eusaurosphargis (Scheyer et al., 2017), in which the widths of the spaces between the dorsal transverse processes are approximately equal or greater than the widths of the transverse processes.
Five posterior dorsal neural arches are preserved in articulation in HFUT YZSB-19-109 and are exposed in ventral view. In adddition, a fragmentary centrum is associated with the second preserved posterior dorsal neural arch. The articulation surfaces for the centra located on the pedicels of the posterior dorsal neural arches are anteroposteriorly elongate, but seem mediolaterally broader and not as well demarcated from the transverse processes as those in the more anterior dorsal neural arches. The posterior dorsal transverse processes gradually become mediolaterally shorter and anteroposteriorly broader posteriorly. Well-developed prezygapophysis-postzygapophysis articulations are preserved and exposed between the second and fifth posterior dorsal neural arches. Articulated anterior and posterior dorsal neural arches preserved without their respective centra indicate a lack of fusion of the neurocentral suture, a paedomorphic feature characteristic for Sauropterygia (Rieppel, 2000a) and also present in Saurosphargis (Huene, 1936) and Largocephalosaurus (Li et al., 2014).
Four complete posterior dorsal/sacral vertebrae are preserved and exposed in left ventrolateral view. Two sacral vertebrae can be identified by the presence of associated sacral ribs with clearly expanded distal ends. However, the distal ends of the first two ribs lying immediately posterior to the last unambiguous dorsal rib are obscured by the overlying ilium and ischium, so it is not possible to confidently determine whether they represent the last two dorsal ribs or the first two sacral ribs (Figure 6A). A series of 11 articulated and complete caudal vertebrae is also preserved, with two anterior caudals exposed in left ventrolateral view and the remaining caudals exposed in lateral view (Figure 6). The sacral and caudal centra have concave lateral and ventral surfaces and are likely amphicoelus, as evidenced by the concave anterior articular surface of the second caudal centrum, but in most cases the articular surfaces are obscured by matrix or adjacent centra, giving a false impression of procoely or amphiplaty. The caudal centra are dorsoventrally taller than anteroposteriorly long and gradually decrease in size posteriorly. The caudal neural arches bear well-developed, anterodorsally inclined prezygapophyses. The neural spines of some caudal neural arches are exposed in lateral view (Figure 6B). They are dorsoventrally short, anteroposteriorly broad and possess a convex dorsal margin. A slightly rugose/striated dorsal surface, reminiscent of a similar surface present in the dorsal neural spines of Helveticosaurus (Rieppel, 1989a), Augustasaurus (Sander et al., 1997), Nothosaurus (Klein et al., 2022), and Pomolispondylus (Cheng et al., 2022), is preserved in some of the anterior caudal neural spines. Rib facets are visible from the second sacral to the penultimate preserved caudal vertebra (Figure 6). The dorsal portions of the rib facets are located on the ventrolateral surfaces of the neural arches, whereas their ventral portions are located on the dorsolateral surfaces of the centra and are demarcated by a prominent, ventrally arcuate ridge (Figure 6B). The surface of the rib facets is rugose. The last visible minute caudal rib is preserved in articulation with the seventh caudal vertebra, but small rib facets are also preserved in the following three vertebrae (Figure 6C). It is not clear, however, if small ossified caudal ribs were associated with these vertebrae in life.
Ribs: Six cervical ribs are preserved in HFUT YZSB-19-109 (Figures 3 and 4). Three anterior cervical ribs are approximately straight and possess a single, expanded head and narrow distal ends. Two posterior cervical ribs are also preserved, but their proximal portions are obscured by the overlying coracoid and scapula. These ribs are much longer than the anterior cervical ribs and possess slightly curved shafts and relatively broad distal ends. HFUT YZSB-19-109 also preserves 16 dorsal ribs, but most of them are incomplete and/or obscured by overlying skeletal elements, such as gastralia or other ribs (Figures 3, 4 and 6A). The anterior dorsal ribs are much more robust than the posterior cervical ribs, being proximodistally longer and anteroposteriorly much broader (Figures 3 and 4). They have a single head and greatly expanded distal portions that abut against each other, forming a characteristic ‘rib-basket’ also present in other saurosphargids (Huene, 1936; Li et al., 2011; Li et al., 2014), but differ from the dorsal ribs of Eusaurosphargis, which are narrow and widely spaced (Scheyer et al., 2017). The dorsal ribs do not bear a distinct uncinate process, being similar in this respect to the dorsal ribs of Sinosaurosphargis (Li et al., 2011), but differ from the dorsal ribs of Largocephalosaurus, Saurosphargis, Eusaurosphargis and a possible isolated saurosphargid rib from the Late Triassic of Switzerland, all of which possess uncinate processes (Li et al., 2014; Scheyer et al., 2017; Scheyer et al., 2022).
Five to seven posterior dorsal ribs are preserved in HFUT YZSB-19-109; they have an expanded head, a narrow distal end and are much shorter and slender in comparison with the more anterior dorsal ribs. As a consequence, they did not form part of the closed ‘rib-basket’. Two to four pairs of sacral ribs are preserved in HFUT YZSB-19-109, although the right sacral ribs are damaged and partially preserved as impressions (see above regarding the uncertainty in establishing the correct number of sacral vertebrae/rib pairs; Figure 6A). Two unambiguous sacral ribs are proximodistally slightly longer than the last evident dorsal rib and possess clearly expanded distal ends. The penultimate left sacral rib bears a small posteroproximal process. The caudal ribs are proximodistally short, possess a broad head and taper distally (Figure 6). They are not fused with the caudal centra. The anterior caudal ribs in HFUT YZSB-19-109 are shorter than the sacral ribs, in contrast to Largocephalosaurus, in which the anterior caudal ribs are longer than the sacral ribs (Li et al., 2014). The caudal ribs decrease in size posteriorly and extend at least to the level of the seventh caudal centrum, although it is not clear if caudal ribs extended posteriorly beyond the seventh caudal (see above). In Largocephalosaurus, the caudal ribs extend to the level of the 10th or 11th caudal centrum (Li et al., 2014).
Chevrons: Eight chevrons are preserved in HFUT YZSB-19-109 in association with some of the posterior caudal vertebrae (Figure 6A and C). In lateral view, the chevrons are slender and straight or display a gentle ventral curvature. The chevrons have a proximal end that is approximately equal in size or only slightly expanded relative to the distal end.
Gastralia: The gastral rib basket is partially preserved in HFUT YZSB-19-109 (Figures 3—5). Like in Eusaurosphargis, Placodus and eosauropterygians (Drevermann, 1933; Rieppel, 1989b; Nosotti and Rieppel, 2003; Shang et al., 2011), each gastral rib was composed of five elements – a median element, two lateral (first lateral) elements and two lateralmost (second lateral) elements. Gastral ribs comprising five elements were also inferred for Saurosphargis (Huene, 1936), whereas in Sinosaurosphargis the gastral ribs were described as comprising three elements – one median and two lateral elements (Li et al., 2011), and the number of gastral rib elements was not specified for Largocephalosaurus (Li et al., 2014). A single anterior median gastral element, 11 lateral and 33 lateralmost elements are preserved in HFUT YZSB-19-109. The median element is weakly angulated anteriorly, whereas the first lateral elements form proportionally short rods with a blunt medial end and a pointed lateral end (Figure 3) and closely resemble the first lateral gastral elements of Placodus (Drevermann, 1933). The lateralmost elements are concentrated into two articulated series comprising 13 (anterior block; Figure 3) and 14 (posterior block; Figure 5) elements each. They form mediolaterally elongate rods which are approximately straight or gently angulated posteriorly. The medial end of the second lateral element is narrow and tapers into a pointed apex, whereas the lateral end is broadened and blunt with a distinctly rugose/granulated surface. One anteriorly positioned first lateral element seems to bifurcate laterally, forming two lateral prongs (Figure 3).
Osteoderms
Several osteoderms are preserved in HFUT YZSB-19-109. A single osteoderm is preserved in the anterior block between the anterior dorsal ribs (Figure 3) and a second isolated osteoderm is preserved in the posterior block between the posterior gastral elements (Figure 5). In addition, two possible osteoderms are also preserved in association with the proximal end of the left humerus (Figure 4). These osteoderms are approximately oval in outline and likely represent osteoderms forming parasaggital rows similar to those in Largocephalosaurus (Li et al., 2014; Scheyer et al., 2022). A partial series comprising six osteoderms is preserved along the distal ends of a series of partially preserved left dorsal ribs (Figure 4). These osteoderms represent the left lateral osteoderm row and are anteroposteriorly elongate, have an irregular, sub-oval, or sub-rectangular outline, and a densely pitted surface. One of the posterior elements in this series bears a prominent ridge extending along the midline of its exposed surface. In all these features, these osteoderms closely resemble the lateral osteoderms reported for Saurosphargis (Huene, 1936) and Largocephalosaurus polycarpon (Li et al., 2014).
Another partial series of small osteoderms is preserved lateral to the distal ends of the right sacral and anterior caudal ribs, indicating that the lateral osteoderm rows extended to the level of the seventh caudal vertebra (Figure 6A). A few small osteoderms are also preserved in close association with the anterior caudal neural spines and seem to have formed a median row along the dorsal midline (Figure 6B), likely overlying the caudal neural spines in a manner similar to that in Largocephalosaurus (Li et al., 2014) and Placodus (Jiang et al., 2008). In L. qianensis, a dense covering of small osteoderms is present on the dorsal surface of the neck, trunk and caudal region (Li et al., 2014), but no such osteoderms were found in association with HFUT YSZB-19-109. A dense covering of osteoderms is also absent in L. polycarpon (Cheng et al., 2012; Li et al., 2014).
Pectoral girdle
Scapula: The right scapula is completely preserved in HFUT YZSB-19-109 (Figure 3). In addition, a large and broad broken bone fragment likely representing the left scapula is visible lying anterodorsally to the left coracoid (Figure 4). The scapula possesses an anteroproximally expanded glenoid portion and a much narrower, straight, and posterodorsally projecting scapular blade. The glenoid portion forms a low acromion process proximodorsally. The coracoid facet is relatively broad and convex, whereas the glenoid facet is straight and short and oriented nearly parallel to the long axis of the scapular blade. The scapular blade is separated from the glenoid portion by a deep posteroventral notch. The scapular blade is straight, with gently concave anterodorsal and posteroventral margins and an approximately straight posterior margin. In general shape and proportions, the scapula of HFUT YZSB-19-109 closely resembles the scapula of Largocephalosaurus (Cheng et al., 2012; Li et al., 2014) and Corosaurus (Storrs, 1991), although the notch separating the glenoid portion from the scapular blade is much deeper and narrower in the latter.
Coracoid: The coracoids are only partially visible in HFUT YZSB-19-109 – the right coracoid is almost entirely covered by the right scapula (Figure 3), whereas the proximal portion of the left coracoid is broken (Figure 4). The preserved parts of both coracoids indicate that it was a dorsoventrally flat, plate-like element, approximately sub-circular in outline, closely resembling the coracoid of Largocephalosaurs (Li et al., 2014; Figure 7). The coracoid of HFUT YZSB-19-109 differs, however, from the coracoids of Helveticosaurus (Rieppel 1989), Eusaurosphargis, Atopodentatus, early diverging placodonts, Majiashanosaurus, a referred specimen of Hanosaurus, and Lariosaurus sanxiaensis, in which the coracoid is proximodistally more elongate and approximately sub-oval in outline (Figure 7). It also differs from the coracoids of eosauropterygians, which possess weakly- or well-developed anterior and posterior emarginations (Figure 7). A small notch in the proximal part of the right coracoid, seen just above the anterior margin of the right scapula, likely represents the coracoid foramen. The exposed ventral surface of the left coracoid bears numerous radial striations extending from the centre of the bone towards its outer margins.
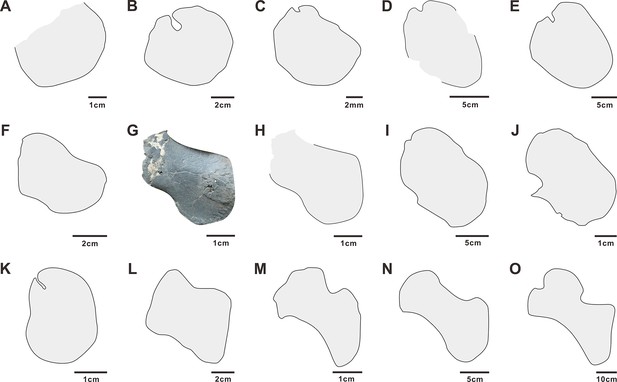
Coracoids of selected Early and Middle Triassic sauropterygians.
A - Prosaurosphargis (based on specimen HFUT YZSB-19-109); B - Largocephalosaurus (after Li et al., 2014); C - Eusaurosphargis (after Scheyer et al., 2017); D - Atopodentatus (after Cheng et al., 2014); E - Placodus (after Drevermann, 1933); F - Paraplacodus (after Rieppel, 2000b); G (photo) and H (outline) - Hanosaurus (holotype, based on specimen IVPP V 3231); I - Hanosaurus (referred specimen; after Wang et al., 2022); J - Lariosaurus sanxiaensis (after Li and Liu, 2020); K – Majiashanosaurus (after Jiang et al., 2014), L – Corosaurus (after Storrs, 1991); M - Wumengosaurus (after Wu et al., 2011); N – Anarosaurus (after Klein, 2012); O – Nothosaurus (after Ji et al., 2014).
Forelimb
Humerus: Both humeri are preserved in HFUT YZSB-19-109 – the right humerus is complete, but partially overlapped by the right scapular blade (Figure 3), whereas the left humerus is slightly broken proximally (Figure 4). The humerus is proximodistally elongate; it is also posteriorly curved along its proximodistal axis, like the humerus of other saurosphargids (Li et al., 2011; Li et al., 2014; Cheng et al., 2012), Placodus (Jiang et al., 2008), Majiashanosaurus (Jiang et al., 2014), Lariosaurus sanxiaensis (Li and Liu, 2020), a referred specimen of Hanosaurus (Wang et al., 2022) and numerous eosauropterygians (Rieppel, 1994). However, in contrast to Largocephalosaurus, the anterior margin of the humerus is not convex, but straight, making it more similar to the humeri of Placodus and Nothosaurus (Rieppel, 1994). The shape of the anterior margin of the humerus is unknown in Sinosaurosphargis. The posterior margin of the humerus is concave. The proximal and distal ends of the humerus are expanded, but a distinct humeral head and distal condyles are not present. Anteriorly, the distal end of the humerus bears a shallow ectepicondylar groove (notch), like in Largocephalosaurus qianensis (Li et al., 2014), but unlike in L. polycarpon (Cheng et al., 2012), which possesses an entepicondylar foramen. Anteroproximally, the exposed surface of the right humerus bears a short, straight groove, which likely represents a muscle insertion site.
Radius: A disarticulated right radius is completely preserved in HFUT YZSB-19-109 in close proximity to the right humerus and right pectoral girdle (Figure 3). The radius is proximodistally elongate and anteriorly curved, with a convex anterior margin and a concave posterior margin. The proximal and distal ends of the radius are straight and slightly expanded, with the proximal end being anteroposteriorly broader than the distal end. The radius is similar in general shape to that in other saurosphargids (Li et al., 2011; Li et al., 2014; Cheng et al., 2012), Majiashanosaurus (Jiang et al., 2014), Placodus (Jiang et al., 2008), and Helveticosaurus (Rieppel, 1989a), but differs from the straight and anteriorly and posteriorly concave radius of Eusaurosphargis (Scheyer et al., 2017). The radius/humerus proximodistal length ratio in HFUT YZSB-19-109 is ~0.55, in line with Eusaurosphargis (~0.51–0.57; Scheyer et al., 2017) and Placodus (~0.56; Jiang et al., 2008), but is significantly smaller than the ratio in Sinosaurosphargis (~0.66; Li et al., 2011), Largocephalosaurus polycarpon (~0.74; Cheng et al., 2012), L. qianensis (~0.67–0.72; Li et al., 2014), a referred specimen of Hanosaurus (~0.67; Wang et al., 2022), and Majiashanosaurus (~0.74; Jiang et al., 2014). This indicates that HFUT YZSB-19-109 had a proportionally shorter forearm compared with other saurosphargids and early eosauropterygians.
Pelvic girdle
Ilium: The left ilium is completely preserved in HFUT YZSB-19-109 and is exposed in lateral or medial view (Figure 5). The ilium consists of a distally expanded acetabular portion and a posteriorly projecting iliac blade, which is largely obscured by the overlying ischium. The ilium of HFUT YZSB-19-109 is very similar to the ilia of Largocephalosaurus (Li et al., 2014), Eusaurosphargis (Nosotti and Rieppel, 2003), Placodus (Rieppel, 1995), and Corosaurus (Storrs, 1991), which all possess a well-developed, posteriorly projecting iliac blade.
Pubis: The left pubis is completely preserved in HFUT YZSB-19-109 and exposed in ventral view, whereas only the distal portion of the right pubis is preserved (Figure 5). The pubis is approximately oval in outline, being proximodistally longer than anteroposteriorly broad and bears a posteroproximally positioned, open obturator foramen. The pubis of HFUT YZSB-19-109 resembles the pubis of Largocephalosaurus (Li et al., 2014) in outline and proportions. It is also similar to the pubis of the holotype and referred specimens of Hanosaurus (Rieppel, 1998a; Wang et al., 2022) and Pararcus diepenbroekii (Klein and Scheyer, 2013), which are however more circular in outline, being approximately as wide proximodistally as long anteroposteriorly. The pubis of HFUT YZSB-19-109 differs markedly from the anteriorly and posteriorly shallowly emarginated pubis of Eusaurosphargis (Scheyer et al., 2017) and Placodus (Drevermann, 1933), and the deeply emarginated pubis of eosauropterygians (e.g. Rieppel, 2000a).
Ischium: The left ischium of HFUT YZSB-19-109 is completely preserved, but is rotated 180° relative to its life position and is exposed in dorsal view, whereas only the distal portion of the right ischium is preserved (Figure 5). The ischium forms an anterodistally convex and posteroproximally concave plate, similar to that in Largocephalosaurus (Li et al., 2014), Pararcus (Klein and Scheyer, 2013) and Hanosaurus (Rieppel, 1998a; Wang et al., 2022), but differs markedly from the anteriorly emarginated ischium of Eusaurosphargis (Scheyer et al., 2017) and the anteriorly and posteriorly emarginated ischia of some placodonts and eosauropterygians (Rieppel, 2000a).
Hindlimb
Femur: The left hindlimb is completely preserved in HFUT YZSB-19-109. The femur is exposed in posterior view (Figure 5). The shaft of the femur is straight and both the proximal and distal ends are expanded. The internal trochanter is well-developed and located proximally, as in Largocephalosaurus (Li et al., 2014), Simosaurus, and Nothosaurus (Rieppel, 1994), but differs from the more distally located trochanter in the holotype of Hanosaurus (Rieppel, 1998a). Distally, the femur produces weakly-developed, but still distinct, condyles for the tibia and fibula, separated ventrally by a shallow popliteal area. The humerus/femur ratio in HFUT YZSB-19-109 is ~1.19, which is similar to the ratio in Largocephalosaurus (~1.20; Li et al., 2014), but is significantly greater than the ratio in Helveticosaurus (~1.11; Rieppel 1989), Placodus (~1.05; Jiang et al., 2008), and Eusaurosphargis (~0.89; Scheyer et al., 2017).
Tibia and fibula: The tibia is proximodistally slightly longer than the fibula and possesses proximally and distally expanded ends, with the proximal end slightly broader than the distal end (Figure 5). Posteroproximally, the tibia bears a proximodistally elongate, shallow facet for the fibula. The anterior and posterior margins of the tibia are gently concave. The fibula possesses an approximately straight posterior margin and a concave anterior margin (Figure 5). The proximal and distal ends of the fibula are slightly expanded, with the distal end being slightly broader than the proximal end. This is in contrast to Largocephalosaurus, in which the proximal end of the fibula is markedly broader than the distal end (Li et al., 2014).
Tarsals: Three tarsals are present in the left hindlimb of HFUT YZSB-19-109 – the largest one being the astragalus, the medium-sized representing the calcaneum, and the smallest element interpreted here as distal tarsal IV (Figure 5). The tarsals are sub-circular in outline, with the astragalus possessing minute notches anteriorly and posteriorly, and their exposed ventral surfaces are weakly concave. The morphology of the tarsals of HFUT YZSB-19-109 is similar to that in Largocephalosaurus, which, however, possesses four tarsals instead of three (astragalus, calcaneum and distal tarsals III and IV; Li et al., 2014).
Metatarsals: All five metatarsals are preserved in the left hindlimb of HFUT YZSB-19-109 (Figure 5). Metatarsal I is the proximodistally shortest metatarsal, being much broader proximally than distally. It is also the most robust of the metatarsals. Metatarsals II–V are slender, approximately hourglass-shaped in outline, with expaned proximal and distal ends. The proximodistal length of the metatarsals increases from metatarsal II–IV, with metatarsal IV being the longest of all metatarsals (similar to Largocephalosaurus polycarpon, but different from L. qianensis, in which metatarsal III is the longest; Li et al., 2014); the length of metatarsal V is comparable to that of metatarsal III. Metatarsal V is proportionally the most slender of the metatarsals (similar to the condition in L. qianensis, but unlike in L. polycarpon, in which metatarsal IV is the most slender; Li et al., 2014).
Phalanges: The pedal phalanges are completely preserved in the left pes of HFUT YZSB-19-109 (Figure 5). The proximal phalanges of digits 1 and 2 are sub-rectangular in outline, whereas those of digits 3–5 are hourglass-shaped and have expanded proximal and distal ends. Distally, the phalanges become proximodistally shorter and sub-rectangular in outline. The phalangeal formula is 2-3-4-5-5 (unguals 2 and 3 are slightly displaced).
Phylogenetic results
The phylogenetic analysis recovered 48 most parsimonious trees (MPTs) of 1008 steps each (CI = 0.272, RI = 0.620) (Figure 8). Prosaurosphargis was recovered as a member of Saurosphargidae, forming a polytomy with Sinosaurosphargis and Largocephalosaurus. Saurosphargidae is supported by the following three unambiguous synapomorphies: dorsal ribs transversely broadened and in antero-posterior contact with each other, forming closed ‘rib-basket’ (char. 135.1); lateral gastralia expanded and flat (char. 141.1); distal end of ulna distinctly expanded (char. 167.1).
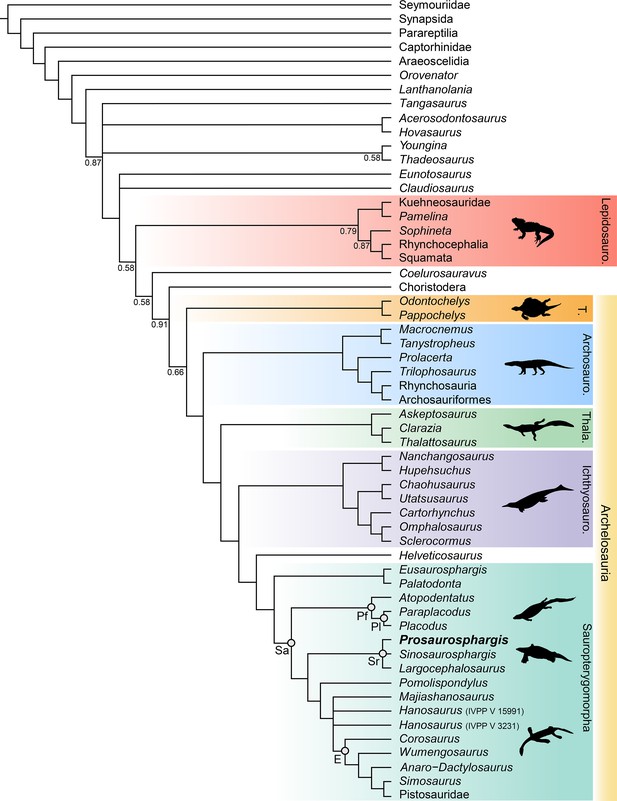
Phylogenetic relationships of Prosaurosphargis yingzishanensis within Diapsida.
The 50% majority rule consensus of 48 most parsimonious trees (MPTs) obtained from analysis of the updated dataset of Qiao et al., 2022. Numbers below nodes indicate proportion of MPTs in which the node is recovered if it is lower than 1. Abbreviations: Archosauro., Archosauromorpha; E, Eosauropterygia; Ichthyosauro., Ichthyosauromorpha; Lepidosauro., Lepidosauromorpha; Pf, Placodontiformes; Pl, Placodontia; Sa, Sauropterygia; Sr, Saurosphargidae; T., Testudines (total-group); Thala., Thalattosauria. All silhouettes are from Phylopic (http://phylopic.org).
© 2013, N Tamura. Ticinosuchus, Askeptosaurus, Utatsusaurus and Paraplacodus silhouettes were drawn by N. Tamura. Used with permission under license CC BY-NC 3.0 (https://creativecommons.org/licenses/by-nc/3.0/). Reproduction of this figure must abide by the terms of this license.
© 2013, G Monger. Keichousaurus silhouette was drawn by G Monger. Used with permission under license CC BY-NC 3.0 (https://creativecommons.org/licenses/by-nc/3.0/). Reproduction of this figure must abide by the terms of this license.
Saurosphargidae was recovered within Sauropterygia, as the sister-group to the lineage leading to Eosauropterygia. The saurosphargid-eosauropterygian clade is supported by six unambiguous synapomorphies: frontal, butterfly-shaped with antero- and postero-lateral processes absent (ch. 29.0); long postorbital posterior process contacting squamosal (ch. 32.0); mandibular articulations displaced to a level distinctly behind occipital condyle (ch. 87.1); neural canal evenly proportioned (ch. 122.0); three sacral ribs (ch. 130.1); total number of carpal ossifications more than three (ch. 194.0).
Pomolispondylus, recently proposed as a sister-taxon of Saurosphargidae (Cheng et al., 2022), was recovered as the most basal member of the grade leading to Eosauropterygia (Pomolispondylus + eosauropterygian lineage supported by a single unambiguous synapomorphy: transverse processes of neural arches of the dorsal region relatively short [ch. 124.0]). The type (Rieppel, 1998a) and referred (Wang et al., 2022) specimens of Hanosaurus were not unambiguously recovered in a monophyletic group – both specimens were recovered alongside Majiashanosaurus in a polytomy at the base of Eosauropterygia (clade comprising Hanosaurus, Majiashanosaurus, and Eosauropterygia supported by a single unambiguous synapomorphy: osteoderms absent [ch. 143.0]). Corosaurus was recovered as the earliest-diverging member of Eosauropterygia, whereas Wumengosaurus was recovered outside of the clade comprising pachypleurosaurs, nothosaurs and pistosaurs.
The herbivorous sauropterygian Atopodentatus was recovered as the sister-taxon of placodonts (represented in our dataset by Paraplacodus and Placodus) within Placodontiformes, supported by four unambiguous synapomorphies: contact of the prefrontal and postfrontal excluding frontal from dorsal orbital margin (char. 21.1); interpterygoid vacuity absent (char. 81.1); splenial entering mandibular symphysis (char. 88.0); and femur internal trochanter well developed (char. 203.0).
Palatodonta was recovered as the sister-taxon of Eusaurosphargis. This clade is supported by one unambiguous synapomorphy – a small premaxilla (char. 196.1). The clade comprising Palatodonta + Eusaurosphargis was recovered as the sister-group to Sauropterygia within Sauropterygomorpha tax. nov. (see above). Helveticosaurus was recovered as the sister-group of Sauropterygomorpha; the clade comprising Helveticosaurus + Sauropterygomorpha is supported by the following six unambiguous synapomorphies: preorbital and postorbital regions of skull of subequal length (ch. 1.0); transverse processes of neural arches of the dorsal region distinctly elongated (ch. 124.1); scapula with a constriction separating a ventral glenoidal portion from a posteriorly directed dorsal wing (ch. 154.2); distal tarsal I absent (ch. 184.1); total number of tarsal ossifications less than four (ch. 192.1); total number of carpal ossifications two (char. 194.2).
Within Diapsida, the clade comprising Helveticosaurus + Sauropterygomorpha was recovered as forming a clade with Ichthyosauromorpha, Thalattosauria and Archosauromorpha, a result similar to that recovered in some other recent broad-scale analyses of diapsid phylogenetic interrelationships (Chen et al., 2014a; Neenan et al., 2015; Martínez et al., 2021; Simões et al., 2022). The three major marine reptile clades (Sauropterygomorpha, Ichthyosauromorpha and Thalattosauria), Archosauromorpha, and Testudines were recovered within a monophyletic Archelosauria supported by four unambiguous synapomorphies – frontal with distinct posterolateral processes (ch. 26.1), frontal anterior margins oblique, forming an angle of at least 30 degrees with long axis of the skull (ch. 27.1), interclavicle anterior process or triangle conspicuously present (ch. 157.0), and upper temporal fossae present and distinctly smaller than the orbit (ch. 207.3).
Discussion
Marine reptile diversity of the Early Triassic Nanzhang-Yuan’an Fauna
Prosaurosphargis represents the stratigraphically oldest occurrence of Saurosphargidae, extending their fossil record back by approximately 3 Ma from the Middle (Pelsonian) to the Early (Olenekian) Triassic (Figure 9). Saurosphargids are thus the fourth major marine reptile lineage known from the Early Triassic Nanzhang-Yuan’an fauna, which also includes as many as seven species of hupehsuchians (Chen et al., 2016a; Qiao et al., 2019), one species of ichthyosauriforms (Chen et al., 2013), and three taxa representing the sauropterygian lineage leading to Eosauropterygia – Hanosaurus, Lariosaurus sanxiaensis, and Pomolispondylus (Young, 1965; Rieppel, 1998a; Li and Liu, 2020; Cheng et al., 2022; Wang et al., 2022). Measuring approximately 1.5 m in total body length, Prosaurosphargis is one of the larger marine reptiles known from this ecosystem, smaller only than a large unidentified eosauropterygian (body length of 3–4 m; Chen et al., 2014b, Chen et al., 2016a) and an indeterminate hupehsuchian (body length of ~2.3 m; Qiao et al., 2019). The presence in the Nazhang-Yuan’an fauna of several marine reptiles representing a broad range of body sizes (~0.25–4.00 m; Chen et al., 2014b; Qiao et al., 2019) and displaying various ecomorphological adaptations supports the view of a rapid diversification of predators in the immediate aftermath of the PTME and high predation pressure in shallow marine ecosystems in the Early Triassic (Chen et al., 2014b; Chen et al., 2014c; Li and Liu, 2020).
Phylogeny of Sauropterygomorpha
A clade comprising Palatodonta + Eusaurosphargis and the lineage leading to Helveticosaurus are recovered as successive outgroups to Sauropterygia, with Palatodonta + Eusaurosphargis and Sauropterygia united within Sauropterygomorpha tax. nov. (see above) (Figure 9). The status of Eusaurosphargis + Palatodonta as the sister-group to Sauropterygia is corroborated by the presence in Eusaurosphargis of morphological features otherwise known exclusively in sauropterygians: a clavicle applied to the medial surface of the scapula and a pectoral fenestration (Scheyer et al., 2017). Furthermore, Eusaurosphargis and Sauropterygia also share a similar foot morphology with metatarsal I being proximodistally much shorter than metatarsal IV and metatarsal V being long and slender (see above). Helveticosaurus shares the presence of a skull with preorbital and postorbital regions subequal in length with Eusaurosphargis and Palatodonta, the presence of elongated dorsal transverse processes with Eusaurosphargis, placodonts and saurosphargids and the presence of a scapular constriction with Eusaurosphargis and sauropterygians. However, other anatomical features uniting it with Sauropterygomorpha include details of carpal and tarsal anatomy, which likely represent aquatic adaptations that might have evolved convergently in Helveticosaurus and aquatic representatives of Sauropterygomorpha (Chen et al., 2014a). Furthermore, Helveticosaurus lacks osteoderms, a feature present in early members of all major lineages within Sauropterygomorpha. Consequently, we interpret Helveticosaurus as a representative of a lineage closely related to Sauropterygomorpha but lying outside of it, that likely convergently evolved an aquatic lifestyle.
Our phylogenetic analysis does not recover Palatodonta from the Middle Triassic of the Netherlands as a sister-taxon of placodonts within Placodontiformes as was previously proposed (Neenan et al., 2013). Instead, Palatodonta is recovered as the sister-taxon of Eusaurosphargis. The Palatodonta + Eusaurosphargis clade recovered in our phylogenetic analysis is supported by one unambiguous synapomorphy – a small (anteroposteriorly short) premaxilla (ch. 196.0). In addition, both taxa also share anteriorly positioned external nares (ch. 197.0). Both of these character states are considered as typical for terrestrial taxa and contrast with the enlarged (anteroposteriorly elongate) premaxillae and posteriorly displaced external narial openings characteristic for marine reptiles (Chen et al., 2014a). A terrestrial lifetyle was previously proposed for Eusaurosphargis on the basis of manus and pes anatomy and bone microanatomy (Scheyer et al., 2017) and the femur of Eusaurosphargis is longer than its humerus (humerus:femur ratio ~0.89; Scheyer et al., 2017), which indicates hindlimb dominance characteristic of a terrestrial lifestyle (Motani and Vermeij, 2021). All this evidence strongly suggests that Palatodonta and Eusaurosphargis were terrestrial reptiles, likely representing the morphology of the last common terrestrial ancestor of Sauropterygia. Palatodonta is known from a single isolated skull (Neenan et al., 2013), whereas Eusaurosphargis is represented by two specimens with well preserved and largely complete postcrania, but only partially preserved skulls (Nosotti and Rieppel, 2003; Scheyer et al., 2017). Because postcranial remains referable to Eusaurosphargis were also reported from the type locality of Palatodonta, including a Palatodonta-like dentary preserved in close association with typical Eusaurosphargis-like vertebrae (Scheyer et al., 2019; Willemse et al., 2019), it is very likely that Palatodonta is a junior synonym of Eusaurosphargis, but the discovery of well-preserved skulls with associated postcranial elements of both of these taxa are needed to further test this hypothesis.
Atopodentatus and placodonts are recovered within Placodontiformes and this grouping is supported by four unambiguous synapomorphies (see above). Atopodentatus and the early-diverging placodonts Placodus and Paraplacodus possess a humerus which is longer than the femur, indicating a high level of adaptation to an aquatic lifestyle (Motani and Vermeij, 2021), but they also possess a massive femoral fourth trochanter and an ilium with a well-developed iliac blade (Jiang et al., 2008; Cheng et al., 2014). These features indicate that the hindlimbs in both Atopodentatus and placodonts were likely still important in locomotion at the bottom of the sea floor and/or on shore in a marginal marine environment and suggest a slightly lower degree of adaptation to an aquatic lifestyle in placodontiforms than in saurosphargids and eosauropterygians, in which the fourth trochanter is more reduced (Rieppel, 2000a; Li et al., 2014). The sister-group relationships of Atopodentatus and placodonts might also explain the absence of placodont fossils in Early Triassic fossil horizons worldwide. It is possible that the lineage leading to placodonts was represented in the Early Triassic by reptiles morphologically more similar to Atopodentatus than to placodonts and that the specialised placodont body plan did not evolve until the Middle Triassic. New discoveries of Early Triassic sauropterygians are likely to introduce new morphological data needed to test this hypothesis.
Pomolispondylus is not recovered as a sister-taxon of Saurosphargidae within Saurosphargiformes (contra Cheng et al., 2022), but as the most basal member of a grade of sauropterygians leading to Eosauropterygia. Such a phylogenetic position is supported by the presence in Pomolispondylus of dorsal transverse processes that are relatively short mediolaterally and broad anteroposteriorly, more similar in proportions to the dorsal transverse processes of Lariosaurus sanxiaensis (Li and Liu, 2020) and Hanosaurus (Wang et al., 2022) – two other representatives of the grade leading to Eosauropterygia – than to the mediolaterally broad and anteroposteriorly narrow dorsal neural spines of saurosphargids (Figure 3; Li et al., 2011; Li et al., 2014). Furthermore, Pomolispondylus possesses rows of rudimentary osteoderms on its body flanks, which are much more reduced than those present in Eusaurosphargis, early-diverging placodonts and saurosphargids (Klein and Scheyer, 2013; Li et al., 2014; Scheyer et al., 2017). Therefore, the osteoderms in Pomolispondylus likely represent a late stage of osteoderm reduction in the lineage leading to Eosauropterygia, rather than the first stages of osteoderm development in the saurosphargid lineage.
The type (Young, 1972; Rieppel, 1998a) and referred (Wang et al., 2022) specimens of Hanosaurus and Majiashanosaurus (Jiang et al., 2014) are also recovered in the paraphyletic grade leading to Eosauropterygia, but in a position more derived than Pomolispondylus. This result is in contrast to previous studies which recovered these taxa as either the outgroup to Saurosphargidae + Sauropterygia (Hanosaurus; Wang et al., 2022), pachypleurosaurs (Rieppel, 1998a; Neenan et al., 2015; Lin et al., 2021) or successive outgroups to a clade comprising Pachypleurosauria + Nothosauroidea to the exclusion of Pistosauroidea (Li and Liu, 2020). Suprisingly, the type specimen of Hanosaurus is not unambiguously recovered in a clade with the referred specimen. Taxonomic distinction of both specimens is supported by the fact that the anteriorly and posteriorly weakly emarginated coracoid of the type specimen of Hanosaurus (Figure 7G and H; Rieppel, 1998a; pers. obs. of IVPP V 3231) more closely resembles that of Corosaurus than the sub-oval coracoid present in the referred specimen of Hanosaurus (Figure 7I; Wang et al., 2022), which likely does not represent Hanosaurus, but is probably closely related or even referable to Lariosaurus sanxiaensis (Figure 7J; Chen et al., 2016b; Li and Liu, 2020). Corosaurus is recovered as the earliest-diverging eosauropterygian, a result similar to that obtained by Rieppel, 1994 and Li and Liu, 2020, but in contrast to some other phylogenetic analyses, which recovered it as a pistosauroid (Rieppel, 1998b; Wang et al., 2022) or the earliest-diverging eusauropterygian (Lin et al., 2021). Wumengosaurus is recovered as the sister-taxon of the clade comprising pachypleurosaurs, nothosaurs and pistosaurs, in contrast to a recent phylogenetic analysis which recovered it wtihin pachypleurosaurs (Xu et al., 2022), but similar to the phylogenetic results obtained by Wu et al., 2011 that recovered Wumengosaurus as the outgroup to a clade comprising pachypleurosaurs and nothosaurs.
The results of our phylogenetic analysis differ significantly from the results of a phylogenetic analysis recently published by Wang et al., 2022, in which Hanosaurus was recovered as the sister-group to a clade comprising Saurosphargidae + Sauropterygia within a monophyletic Sauropterygiformes. However, we believe that our phylogenetic analysis presents a more accurate topology of sauropterygians and their relatives for the following reasons. Wang et al., 2022 used 16 outgroup (non-sauropterygiform) taxa (15 marine reptiles and a single terrestrial reptile), representing five major reptile lineages, whereas our study included 40 outgroup (non-sauropterygomorph) taxa (19 terrestrial and 21 aquatic reptiles), representing 23 major reptile lineages. The inclusion of only a single terrestrial outgroup taxon – Youngina – in the analysis of Wang et al., 2022 is problematic because Youngina likely represents a taxon rather distantly related to the Mesozoic marine reptile clade which includes Sauropterygia (Figure 8; see also Simões et al., 2022). Distantly related taxa likely share fewer character states with derived taxa (homoplasy accumulation through time), so a phylogenetic analysis containing a single, distantly related outgroup taxon will likely fail to adequately capture important character transformation sequences, in contrast to a phylogenetic analysis in which outgroups are more comprehensively sampled (Wilberg, 2015). Furthermore, the phylogenetic analysis of Wang et al., 2022 used 181 morphological characters, in contrast to 221 characters included in our study. Greater character sampling has been demonstrated as an important factor increasing the accuracy of phylogenetic reconstructions (Wiens, 2006), which also favours the results obtained by our analysis.
The different topologies recovered by both studies are likely also partially a consequence of differences in character scoring. For example, Wang et al., 2022 scored the humerus of Hanosaurus as ‘rather straight’ (plesiomorphic state), similar to the humerus of Youngina and other non-sauropterygiform marine reptiles. However, in our opinion, the humerus morphology of Hanosaurus matches the typical ‘curved’ morphology (derived state) characteristic of saurosphargids, placodonts and the majority of eosauropterygians (Rieppel, 2000a). Wang et al., 2022 also scored Hanosaurus into an updated version of the phylogenetic matrix of Neenan et al., 2013. Hanosaurus was recovered as the earliest-diverging sauropterygiform in this analysis as well, but its humerus was also scored as plesiomorphic (‘rather straight’) in the character-taxon matrix. Interestingly, this updated analysis of Neenan et al., 2013 included a more comprehensive outgroup (non-sauropterygiform) sample (12 terrestrial and 5 aquatic taxa, representing 12 major reptile lineages) than the analysis of Wang et al., 2022 and recovered Eusaurosphargis and Helveticosaurus as successive sister-groups to Sauropterygia – a result similar to the one obtained in this study (Figure 9).
The early evolutionary assembly of the sauropterygian body plan
Our phylogenetic analysis suggests that Eusaurosphargis and Palatodonta likely represent the morphology of the last common terrestrial ancestor of sauropterygians, indicating it possessed well-developed dermal armour and the characteristic pectoral girdle and pes morphology that underwent further modifications in Sauropterygia. The topology recovered by our phylogenetic analysis demonstrates that the early evolution of sauropterygians first involved diversification within a shallow marine environment and exploration of various food resources, as evidenced by the disparate ecologies exhibited by Atopodentatus (herbivore), placodonts (durophages), saurosphargids, and early-diverging members of the eosauropterygian lineage (likely feeding on fish and invertebrates). Three key episodes can be identified in the evolution of the eosauropterygian body plan (Figure 10). The first, represented by Pomolispondylus, involved a reduction of osteoderms and shortening of the transverse processes of the dorsal neural spines, features well-developed in Eusaurosphargis, placodonts and saurosphargids. Majiashanosaurus and the referred specimen of Hanosaurus exemplify the second episode, in which osteoderms underwent complete reduction. The type specimen of Hanosaurus represents the earliest stage of the evolution of the characteristic eosauropterygian pectoral girdle morphology with an anteriorly and posteriorly emarginated coracoid that ultimately allowed eosauropterygians to become efficient, paraxial swimmers. The presence of the stratigraphically oldest saurosphargid (Prosaurosphargis), stratigraphically oldest representatives of the lineage leading to Eosauropterygia (Pomolispondylus, Hanosaurus, Majiashanosaurus), and the earliest-diverging placodontiform Atopodentatus in the Early–Middle Triassic of South China (Figure 9) indicates that sauropterygians likely originated and underwent rapid diversification in South China in the aftermath of the end-Smithian extinction, similar to ichthyosauromorphs (Motani et al., 2017; Moon and Stubbs, 2020), but well-constrained stratigraphic data for early sauropterygians are needed to further test this hypothesis.
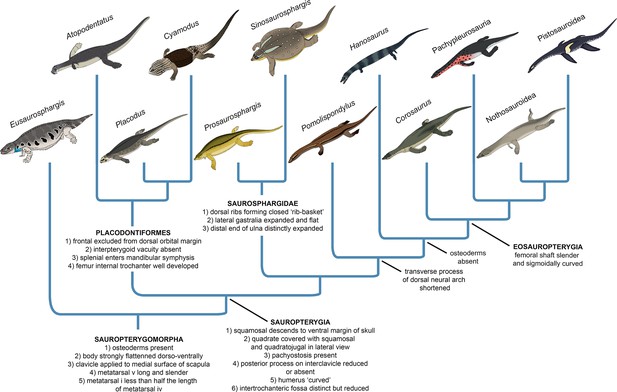
Evolution of the sauropterygian body plan.
Simplified phylogeny of Sauropterygomorpha with key anatomical traits (synapomorphies reconstructed from the phylogenetic analysis) indicated for important nodes.
Our phylogenetic analysis indicates the important role of body armour in sauropterygian evolution (Figure 10). Dermal armour was likely an important preadaptation that allowed colonisation of the shallow marine realm by a Eusaurosphargis-like ancestor, enabling it to counteract buoyancy and walk on the bottom of the shallow sea in search of food (Houssaye, 2009). Elaboration of the dermal armour occurred in the shallow marine placodonts and saurosphargids, perhaps as a response to predation pressure (Liu et al., 2014; Chen et al., 2014b; Qiao et al., 2019). The reduction and complete loss of dermal armour then occurred in the lineage leading to Eosauropterygia, and was likely associated with the evolution of active predation, an efficient swimming style and increasing adaptation to a pelagic lifestyle. The evolution of the sauropterygian body plan demonstrates striking parallels with the evolution of the body plan of another important group of Mesozoic marine reptiles – the ichthyosaurs. Early-diverging representatives of Ichthyosauromorpha – hupehsuchians and omphalosaurids – also possesed a covering of osteoderms superficially similar to that present in Eusaurosphargis, early-diverging placodonts, and saurosphargids (Chen et al., 2014b; Jiang et al., 2016; Qiao et al., 2022). In the ichthyosauromorph lineage, the osteoderm covering was completely lost in Chaohusaurus, a basal ichthyosauriform that evolved an anguilliform mode of swimming and most likely a pelagic lifestyle (Motani et al., 1996; Nakajima et al., 2014; Qiao et al., 2022). This indicates that the evolutionary reduction of dermal armour in both sauropterygians and ichthyosauromorphs followed a similar pattern, probably in response to increasing adaptation to an aquatic lifestyle. These evolutionary parallels seem to demonstrate that the dermal body armour could have been a possible prerequsite (preadaptation) for the invasion of the shallow marine realm in different diapsid clades, which allowed for buoyancy reduction and exploration of the shallow marine environment in search of food. Fossils of terrestrial relatives of ichthyosauromorphs and thalattosaurs are needed to further test this evolutionary scenario.
Phylogenetic interrelationships within Diapsida
Our phylogenetic analysis is thus far one of only two phylogenetic analyses based on a morphology-only dataset of Diapsida that recovers a close relationship between Archosauromorpha and Testudines within a monophyletic Archelosauria (Figure 8) (see also Simões et al., 2022). A close phylogenetic relationship between Archosauromorpha and Testudines has been strongly supported by molecular data for the last twenty years, but was until recently not recovered by any phylogenetic analysis based entirely on morphological data, in which turtles were usually recovered as more closely related to lepidosauromorphs than archosauromorphs (reviewed in Lyson and Bever, 2020). Furthermore, our analysis recovers Eunotosaurus from the Permian of South Africa outside of Sauria (Figure 8), which is in agreement with the results obtained by Simões et al., 2022, but in contrast to all other recent phylogenetic analyses focussing on the phylogenetic interrelationships among Reptilia, which recovered Eunotosaurus as a stem turtle (Lyson et al., 2013; Bever et al., 2015; Li et al., 2018; Schoch and Sues, 2018). This indicates that the characteristic morphological features of the skull and postcranial skeleton shared between Eunotosaurus and early turtles, such as elongate vertebrae and broadened ribs, evolved convergently in both taxa. Archelosauria are supported in our analysis by four unambiguous synapomorphies associated with the morphology of the skull and shoulder girdle (see above). This demonstrates that innovations of the cranium linked with the evolution of sensory organs and the feeding apparatus, as well as changes in locomotion, perhaps underlie the evolutionary success of archelosaurian reptiles.
Conclusions
The new saurosphargid Prosaurosphargis yingzishanensis gen. et sp. nov. from the Early Triassic of South China (Figure 11) represents the earliest reported occurrence of Saurosphargidae, extending their temporal range back by 3 Ma. An updated phylogenetic analysis of Diapsida recovers saurosphargids as nested within Sauropterygia, forming a clade with Eosauropterygia to the exclusion of Placodontia. A clade comprising Eusaurosphargis and Palatodonta forms the sister-group to Sauropterygia within Sauropterygomorpha tax. nov. and their morphology likely represents the morphology of the last common terrestrial ancestor of Sauropterygia. The herbivorous sauropterygian Atopodentaus is recovered within Placodontiformes, whereas Pomolispondylus, Hanosaurus and Majishanosaurus form a grade at the base of Eosauropterygia, with the type and referred specimens of Hanosaurus likely representing distinct taxa. Our new phylogenetic hypothesis indicates sauropterygians originated and diversified in South China in the aftermath of the Permo-Triassic mass extinction event and suggests an important role of dermal armour in their early evolutionary history. Three major marine reptile clades – Sauropterygomorpha, Ichthyosauromorpha and Thalattosauria – are recovered within Archelosauria, together with Archosauromorpha and Testudines. Our study demonstrates the importance of including not only a broad sample of outgroup taxa in phylogenetic analyses, but also choosing their stratigraphically oldest and/or anatomically most plesiomorphic representatives as operational taxonomic units, in order to accurately reconstruct the phylogenetic relationships between major extant and extinct reptilian lineages.
Materials and methods
In order to investigate the phylogenetic position of Prosaurosphargis yingzishanensis, specimen HFUT YZSB-19-109 was scored into a modified version of a data matrix focusing on the phylogenetic interrelationships between the major groups of diapsid reptiles published by Qiao et al., 2022, which in itself is a modified version of the data matrix previously published by Jiang et al., 2016 and Chen et al., 2014a. The data matrix contains 57 OTUs (Operational Taxonomic Units) scored for a total of 221 characters – characters 1–220 are the original characters of Qiao et al., 2022 and character 221 was adapted from Li et al., 2014 (ch. 88) (Source data 1). In addition to Prosaurosphargis, 10 OTUs were added to the original dataset of Qiao et al., 2022: Eunotosaurus africanus (scored after Cox, 1969; Gow, 1997; Lyson et al., 2013; Lyson et al., 2016; Bever et al., 2015), Pappochelys rosinae (Schoch and Sues, 2015; Schoch and Sues, 2018); Hanosaurus hupehensis (type specimen) (Rieppel, 1998a and personal observation of specimen IVPP V 3231), Hanosaurus hupehensis (referred specimen) (Wang et al., 2022), Majiashanosaurus discocoracoidis (Jiang et al., 2014), Corosaurus alcovensis (Storrs, 1991; Rieppel, 1998b), Atopodentatus unicus (Cheng et al., 2014; Li et al., 2016), Palatodonta bleekeri (Neenan et al., 2013), Paraplacodus broilii (Peyer, 1935; Rieppel, 2000b), and Pomolispondylus biani (Cheng et al., 2022). This was done in order to include the majority of currently known Early Triassic sauropterygians in the data matrix and increase the sampling of early-diverging representatives of the main sauropterygian lineages, as well as their potential sister-groups. Furthermore, the holotype and referred specimen of Sclerocormus, included as separate OTUs in the dataset of Qiao et al., 2022, were merged into a single OTU in the current analysis.
Parsimony analysis of the data matrix was performed in TNT 1.5 (Goloboff and Catalano, 2016) using a Traditional Search algorithm (random seed = 1, replications of Wagner trees = 1000, number of trees saved per replication = 10), followed by an additional round of TBR branch-swapping. All characters were treated as equally weighted and unordered.
Data availability
Specimen HFUT YZSB-19-109 is housed in the collections of the Geological Museum, Hefei University of Technology, Hefei, China and available for examination upon request to JL. The phylogenetic data matrix used in this study is available in Source data 1.
References
-
Extinction events among Mesozoic marine reptilesHistorical Biology 7:313–324.https://doi.org/10.1080/10292389409380462
-
BookMarine reptilesIn: MacLeod N, Archibald JD, Levin P, editors. Grzimek’s Animal Life Encyclopedia: Extinction. Gale Research Inc. pp. 267–279.
-
Faunal turnover of marine tetrapods during the Jurassic-Cretaceous transitionBiological Reviews of the Cambridge Philosophical Society 89:1–23.https://doi.org/10.1111/brv.12038
-
BookWhen Life Nearly Died: The Greatest Mass Extinction of All TimeThames & Hudson.
-
A new Triassic primitive ichthyosaur from Yuanan, South ChinaActa Geologica Sinica 87:672–677.https://doi.org/10.1111/1755-6724.12078
-
Nangzhang-Yuan’an Fauna, Hubei Province and its significance for biotic recoveryActa Geologica Sinica 90:409–420.
-
BookTriassic Marine Reptile Faunas from Middle and Upper Yangtze Areas and Their Coevolution with EnvironmentGeological Publishing House.
-
ConferenceCarbonate laminites from the Nanzhang-Yuanan fauna in the Lower Triassic Jialingjiang Formation, South China: origins and significancesThe 21st International Sedimentological Congress.
-
A new marine reptile from the Triassic of China, with A highly specialized feeding adaptationDie Naturwissenschaften 101:251–259.https://doi.org/10.1007/s00114-014-1148-4
-
Characteristics and significance of Nanzhang/Yuanan Fauna, Hubei ProvinceGeology in China 42:676–684.
-
The problematic permian reptile eunotosaurusBulletin of the British Museum (Natural History) Geology 18:165–196.
-
A phylogenomic analysis of turtlesMolecular Phylogenetics and Evolution 83:250–257.https://doi.org/10.1016/j.ympev.2014.10.021
-
Reptile phylogeny and the interrelationships of turtlesZoological Journal of the Linnean Society 120:281–354.https://doi.org/10.1111/j.1096-3642.1997.tb01280.x
-
Die Placodontier. 3. Das Skelett von Placodus gigas Agassiz im Senckenberg-MuseumAbhandlungender Senckenbergischen Naturforschenden Gesellschaft 38:319–364.
-
A reassessment of Eunotosaurus africanus Seeley (Amniota: Parareptilia)Palaeontologia Africana 34:33–42.
-
The endoskeletal origin of the turtle carapaceNature Communications 4:2107.https://doi.org/10.1038/ncomms3107
-
“Pachyostosis” in aquatic amniotes: a reviewIntegrative Zoology 4:325–340.https://doi.org/10.1111/j.1749-4877.2009.00146.x
-
A new specimen of Nothosaurus youngi from the Middle Triassic of Guizhou, ChinaJournal of Vertebrate Paleontology 34:465–470.https://doi.org/10.1080/02724634.2013.808204
-
First record of Placodontoidea (Reptilia, Sauropterygia, Placodontia) from the Eastern TethysJournal of Vertebrate Paleontology 28:904–908.https://doi.org/10.1671/0272-4634(2008)28[904:FROPRS]2.0.CO;2
-
The early Triassic eosauropterygian Majiashanosaurus discocoracoidis, genJournal of Vertebrate Paleontology 34:1044–1052.https://doi.org/10.1080/02724634.2014.846264
-
Selective extinction of Triassic marine reptiles during long-term sea-level changes illuminated by seawater strontium isotopesPalaeogeography, Palaeoclimatology, Palaeoecology 400:9–16.https://doi.org/10.1016/j.palaeo.2012.07.026
-
A new placodont sauropterygian from the Middle Triassic of the NetherlandsActa Palaeontologica Polonica 59:887–902.https://doi.org/10.4202/app.2012.0147
-
The horizon and age of the marine reptiles from Hubei Province, ChinaVertebrata Palasiatica 40:241–244.
-
A new Triassic marine reptile from southwestern ChinaJournal of Vertebrate Paleontology 31:303–312.https://doi.org/10.1080/02724634.2011.550368
-
The earliest herbivorous marine reptile and its remarkable jaw apparatusScience Advances 2:e1501659.https://doi.org/10.1126/sciadv.1501659
-
Research status of Hupehsuchia and preliminary understanding of their environmental evolutionResources Environment & Engineering 34:485–493.
-
Panzhousaurus rotundirostris Jiang et al., 2019 (Diapsida: Sauropterygia) and the recovery of the monophyly of PachypleurosauridaeJournal of Vertebrate Paleontology 41:e1901730.https://doi.org/10.1080/02724634.2021.1901730
-
Evolutionary origin of the turtle shellCurrent Biology 23:1113–1119.https://doi.org/10.1016/j.cub.2013.05.003
-
Fossorial origin of the turtle shellCurrent Biology 26:1887–1894.https://doi.org/10.1016/j.cub.2016.05.020
-
Origin and evolution of the turtle body planAnnual Review of Ecology, Evolution, and Systematics 51:143–166.https://doi.org/10.1146/annurev-ecolsys-110218-024746
-
Mittheilung, an Professor Bronn gerichtetNeues Jahrbuch Für Mineralogie, Geognosie, Geologie Und Petrefaktenkunde 1839:559–560.
-
Early high rates and disparity in the evolution of ichthyosaursCommunications Biology 3:68.https://doi.org/10.1038/s42003-020-0779-6
-
Pre- versus post-mass extinction divergence of Mesozoic marine reptiles dictated by time-scale dependence of evolutionary ratesProceedings. Biological Sciences 284:20170241.https://doi.org/10.1098/rspb.2017.0241
-
Ecophysiological steps of marine adaptation in extant and extinct non-avian tetrapodsBiological Reviews of the Cambridge Philosophical Society 96:1769–1798.https://doi.org/10.1111/brv.12724
-
Osteohistology of the Early Triassic ichthyopterygian reptile Utatsusaurus hataii: Implications for early ichthyosaur biologyActa Palaeontologica Polonica 59:343–352.
-
The cranial anatomy of Chinese placodonts and the phylogeny of Placodontia (Diapsida: Sauropterygia)Zoological Journal of the Linnean Society 175:415–428.https://doi.org/10.1111/zoj.12277
-
Eusaurosphargis dalsassoi N. gen N. sp., a new, unusual diapsid reptile from the Middle Triassic of Besano (Lombardy, N Italy)Memorie Della Società Italiana Di Scienze Naturali e Del Museo Civico Di Storia Naturale Di Milano 31:3–33.
-
The reptilian subclasses Diapsida and Synapsida and the early history of the DiaptosauriaMemoirs of the American Museum of Natural History 1:449–507.
-
BookPalaeontology; or, a Systematic Summary of Extinct Animals and Their Geologic RemainsAdam and Charles Black, Edinburgh.https://doi.org/10.5962/bhl.title.168965
-
Die Triasfauna der Tessiner Kalkalpen VIII Weitere PlacodontierfundeAbhandlungen Der Schweizerischen Palaontologischen Gesellschaft 55:1–26.
-
Helveticosaurus zollingeri Peyer (Reptilia, Diapsida) skeletal paedomorphosis, functional anatomy and systematic affinitiesPalaeontographica. Abteilung A, Paläozoologie, Stratigraphie 208:123–152.
-
A new pachypleurosaur (Reptilia: Sauropterygia) from the Middle Triassic of Monte San Giorgio, SwitzerlandPhilosophical Transactions of the Royal Society of London. B, Biological Sciences 323:1–73.https://doi.org/10.1098/rstb.1989.0001
-
Osteology of Simosaurus gaillardoti, and the phylogenetic interrelationships of stem-group SauropterygiaFieldiana 28:1–85.
-
The systematic status of Hanosaurus hupehensis (Reptilia, Sauropterygia) from the Triassic of ChinaJournal of Vertebrate Paleontology 18:545–557.https://doi.org/10.1080/02724634.1998.10011082
-
Corosaurus alcovensis Case and the phylogenetic interrelationships of Triassic stem-group SauropterygiaZoological Journal of the Linnean Society 124:1–41.https://doi.org/10.1111/j.1096-3642.1998.tb00568.x
-
BookSauropterygia IIn: Wellnhofer P, editors. Encyclopedia of Paleoherpetology. Verlag Dr. Friedrich Pfeil. pp. 1–134.
-
Paraplacodus and the phylogeny of the Placodontia (Reptilia: Sauropterygia)Zoological Journal of the Linnean Society 130:635–659.https://doi.org/10.1111/j.1096-3642.2000.tb02204.x
-
Feeding mechanics in Triassic stem-group sauropterygians: the anatomy of a successful invasion of Mesozoic seasZoological Journal of the Linnean Society 135:33–63.https://doi.org/10.1046/j.1096-3642.2002.00019.x
-
A new pistosaurid (Reptilia: Sauropterygia) from the Middle Triassic of Nevada and its implications for the origin of the plesiosaursJournal of Vertebrate Paleontology 17:526–533.https://doi.org/10.1080/02724634.1997.10010999
-
With plates and spikes-the heavily armoured Eusaurosphargis aff dalsassoiStaringia 16:216–222.
-
Osteology of the Middle Triassic stem-turtle Pappochelys rosinae and the early evolution of the turtle skeletonJournal of Systematic Palaeontology 16:927–965.https://doi.org/10.1080/14772019.2017.1354936
-
A new eosauropterygian from Middle Triassic of eastern Yunnan Province, southwestern ChinaVertebrata PalAsiatica 49:155–171.
-
Anatomy and relationships of Corosaurus alcovensis (Diapsida: Sauropterygia) and the Triassic Alcova limestone of WyomingBulletin of the Peabody Museum of Natural History 44:1–151.
-
ConferenceSedimentary characteristics of the second member of Jialingjiang formation in the Lower Triassic of the Middle Yangtze Area and its reflection on the burial environment of marine reptilesAbstract Volume of the 26th Annual Academic Meeting of the Palaeontological Society of China.
-
An adult specimen of Sinocyamodus xinpuensis (Sauropterygia: Placodontia) from Guanling, Guizhou, ChinaZoological Journal of the Linnean Society 185:910–924.https://doi.org/10.1093/zoolinnean/zly080
-
Missing data and the design of phylogenetic analysesJournal of Biomedical Informatics 39:34–42.https://doi.org/10.1016/j.jbi.2005.04.001
-
An aff. Eusaurosphargis vertebra associated with an isolated dentaryStaringia 16:273–277.
-
New information on Wumengosaurus delicatomandibularis Jiang et al., 2008 (Diapsida: Sauropterygia), with a revision of the osteology and phylogeny of the taxonJournal of Vertebrate Paleontology 31:70–83.
-
ConferenceStratigraphic characterization and paleoenvironmental analysis of Early Triassic Nanzhang-Yuan’an Fauna, western Hubei ProvinceAbstract Volume of Joint Meetings on the 12th National Congress and 29th Annual Academic Meeting of the Palaeontological Society of China. pp. 237–238.
-
Strata characteristics of the Early Triassic Nanzhang-Yuan’an Fauna in Western Hubei ProvinceEarth Science 46:122–135.
-
On the new nothosaurs from Hupeh and Kweichou, ChinaVertebrata PalAsiatica 9:337–356.
-
A marine lizard from Nanchang, Hupeh Province. Memoirs of the Institute of Vertebrate Paleontology and PaleoanthropologyAcademia Sinica 9:17–28.
-
ConferenceNew research progress on a specimen of Chaohusaurus zhangjiawanensis (diapsida: ichthyosauromorpha)Acta Geologica Sinica. pp. 769–782.
Article and author information
Author details
Funding
National Natural Science Foundation of China (42172026)
- Jun Liu
National Natural Science Foundation of China (41772003)
- Jun Liu
National Natural Science Foundation of China (42202006)
- Andrzej S Wolniewicz
National Natural Science Foundation of China (41902104)
- Yuefeng Shen
National Natural Science Foundation of China (41807333)
- Yuanyuan Sun
Chengdu Center of China Geological Survey (Liu Baojun Funding)
- Yuanyuan Sun
China Postdoc Council (International Postdoctoral Exchange Fellowship Program)
- Andrzej S Wolniewicz
The funders had no role in study design, data collection and interpretation, or the decision to submit the work for publication.
Acknowledgements
We thank PM Sander for early discussions, W Lin for sharing photographs of the Hanosaurus holotype, BY Xiang, XJ Liu and the HFUT Paleontology Lab members for assistance in the field, and the Willi Hennig Society for making the programme TNT publicly available. F Huang, L Li and T Sato skilfully prepared the fossil specimen. T Hollmann produced the skeletal reconstruction in Figure 2B and the sauropterygian cartoons in Figures 9 and 10, and Z Han provided the life reconstruction in Figure 11. The senior editor GH Perry, reviewing editor N Ibrahim, reviewer MJ Benton and another annoymous reviewer provided constructive comments that helped to improve the manuscipt.
Copyright
© 2023, Wolniewicz et al.
This article is distributed under the terms of the Creative Commons Attribution License, which permits unrestricted use and redistribution provided that the original author and source are credited.
Metrics
-
- 2,367
- views
-
- 514
- downloads
-
- 11
- citations
Views, downloads and citations are aggregated across all versions of this paper published by eLife.
Download links
Downloads (link to download the article as PDF)
Open citations (links to open the citations from this article in various online reference manager services)
Cite this article (links to download the citations from this article in formats compatible with various reference manager tools)
Further reading
-
- Evolutionary Biology
Wolbachia are maternally transmitted bacterial symbionts that are ubiquitous among arthropods. They can hijack host reproduction in various ways, including male-killing (MK), where the sons of infected mothers are killed during development. The recent discovery of MK-associated Wolbachia genes, i.e., oscar in Ostrinia moths and wmk in Drosophila flies, stimulates our interest in the diversity and commonality of MK mechanisms, which remain largely unclear. We recently discovered that a Wolbachia symbiont of the moth Homona magnanima carries an MK-associated prophage region encoding homologs of oscar (Hm-oscar) and wmk (wmk-1–4). Here, we investigated the effects of these genes in the native host. Upon transient overexpression, Hm-oscar, but not wmk, induced male lethality in H. magnanima, in contrast to our observations in Drosophila, where the wmk homologs, but not Hm-oscar, killed the males. Hm-oscar disrupted sex determination in male embryos by inducing a female-type doublesex splicing and impaired dosage compensation, recapitulating the Wolbachia phenotype. Cell-based transfection assays confirmed that Hm-oscar suppressed the function of masculinizer, the primary male sex determinant involved in lepidopteran dosage compensation. Our study highlights the conserved roles of oscar homologs in Wolbachia-induced lepidopteran MK and argues that Wolbachia have evolved multiple MK mechanisms in insects.
-
- Evolutionary Biology
The phenomenon of parallel evolution, whereby similar genomic and phenotypic changes occur across replicated pairs of populations or species, is widely studied. Nevertheless, the determining factors of parallel evolution remain poorly understood. Theoretical studies have proposed that pleiotropy, the influence of a single gene on multiple traits, is an important factor. In order to gain a deeper insight into the role of pleiotropy for parallel evolution from standing genetic variation, we characterized the interplay between parallelism, polymorphism, and pleiotropy. The present study examined the parallel gene expression evolution in 10 replicated populations of Drosophila simulans, which were adapted from standing variation to the same new temperature regime. The data demonstrate that the parallel evolution of gene expression from standing genetic variation is positively correlated with the strength of pleiotropic effects. The ancestral variation in gene expression is, however, negatively correlated with parallelism. Given that pleiotropy is also negatively correlated with gene expression variation, we conducted a causal analysis to distinguish cause and correlation and evaluate the role of pleiotropy. The causal analysis indicated that both direct (causative) and indirect (correlational) effects of pleiotropy contribute to parallel evolution. The indirect effect is mediated by historic selective constraint in response to pleiotropy. This results in parallel selection responses due to the reduced standing variation of pleiotropic genes. The direct effect of pleiotropy is likely to reflect a genetic correlation among adaptive traits, which in turn gives rise to synergistic effects and higher parallelism.