Structure–function analysis of Lactiplantibacillus plantarum DltE reveals D-alanylated lipoteichoic acids as direct cues supporting Drosophila juvenile growth
Figures
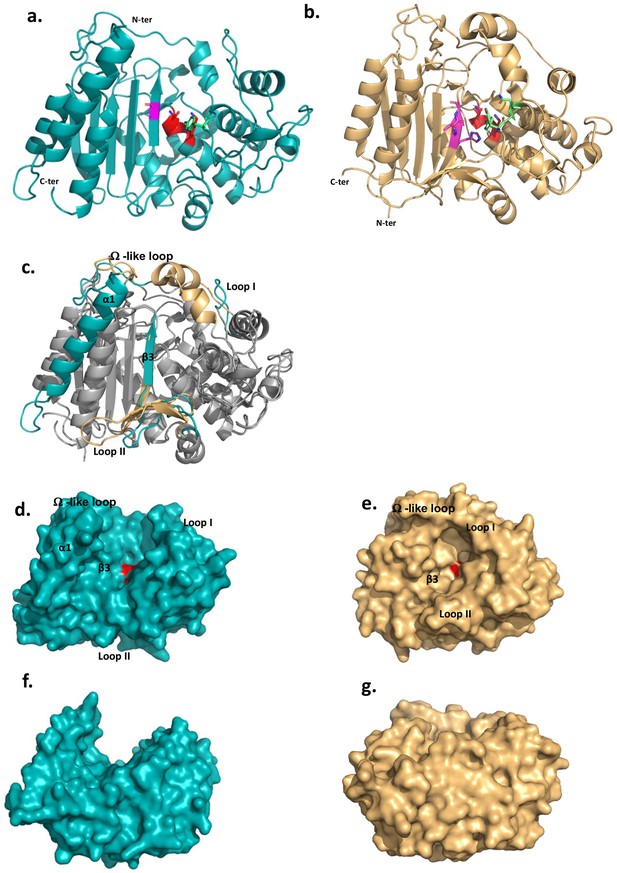
The 3D structures of L. plantarum DltEextra and its structural comparison with the Streptomyces R61 D-Ala-D-Ala carboxypeptidase structure.
(a) Cartoon representations of the 3D X-ray structure of L. plantarum DltEextra and (b) of the canonical D-Ala-D-Ala carboxypeptidase from Streptomyces sp. R61 (DDCP) (PDB ID 1HVB/1IKG). The N- and C-termini are indicated. The canonical DDCP conserved motifs 1 (SXXK, with S the catalytic Ser), 2 (YXN), and 3 ((K/H)(S/T)G) are highlighted in red, green, and pink, respectively. The first motif is strictly conserved in DltE (128SIQK131) and located as for DDCP at the beginning of the α-helix-rich region (α3 helix in DltE, α2 in DDCP) at the interface with the β-sheet. Only the Tyr and Gly residues are conserved in the motifs 2 and 3, respectively. The position of the catalytic Tyr213 from the second motif is also conserved and found in the loop connecting α5 and α6, close to the catalytic dyad. (c) Superimposition between DltEextra and DDCP (PDB ID 1HVB/1IKG) 3D structures. The common structural cores that exhibit a classical β-lactamase fold or penicillin binding (PB) fold are colored in gray. They are well superimposed with rms deviation calculated at 2.2 Å on around 300 residues. The main structural differences are highlighted in teal for DltE and wheat for DDCP and indicated on the figure: the N-terminal α1 helix of DltE, the β3-strand that contains the motif 3, the Loop I, the Loop II, and the Ω-like loop. (d, f) and (e, g) Surface representation of DltEextra and DDCP. (d, e) and (f, g) are shown in the same orientation. (f) and (g) are rotated by 90° along a horizontal axis compared to (d) and (e), respectively. The catalytic Ser, colored in red, is buried at the bottom of the catalytic cavity in DDCP and lying in the middle of a large cleft in DltE. The structural elements that define the active site architecture and differ between DltE and DDCP are indicated.
-
Figure 1—source data 1
DltE 3D structure.
- https://cdn.elifesciences.org/articles/84669/elife-84669-fig1-data1-v2.xlsx
-
Figure 1—source data 2
Raw SDS-PAGE analysis of the purity of DltEextra.
- https://cdn.elifesciences.org/articles/84669/elife-84669-fig1-data2-v2.pdf
-
Figure 1—source data 3
Labeled SDS-PAGE analysis of the purity of DltEextra.
- https://cdn.elifesciences.org/articles/84669/elife-84669-fig1-data3-v2.pdf
-
Figure 1—source data 4
Size-exclusion chromatography (SEC) of the Ni-affinity purified DltEextra on a Superdex 200 10/300 GL.
- https://cdn.elifesciences.org/articles/84669/elife-84669-fig1-data4-v2.xlsx
-
Figure 1—source data 5
Microscale thermophoresis (MST) normalized dose–response data for the binding interaction between penicillin and S. pneumoniae PBP2b.
The experiment was made in triplicate.
- https://cdn.elifesciences.org/articles/84669/elife-84669-fig1-data5-v2.xlsx
-
Figure 1—source data 6
Microscale thermophoresis (MST) normalized dose–response data for the binding interaction between penicillin and DltEextra.
The experiment was made in triplicate.
- https://cdn.elifesciences.org/articles/84669/elife-84669-fig1-data6-v2.xlsx
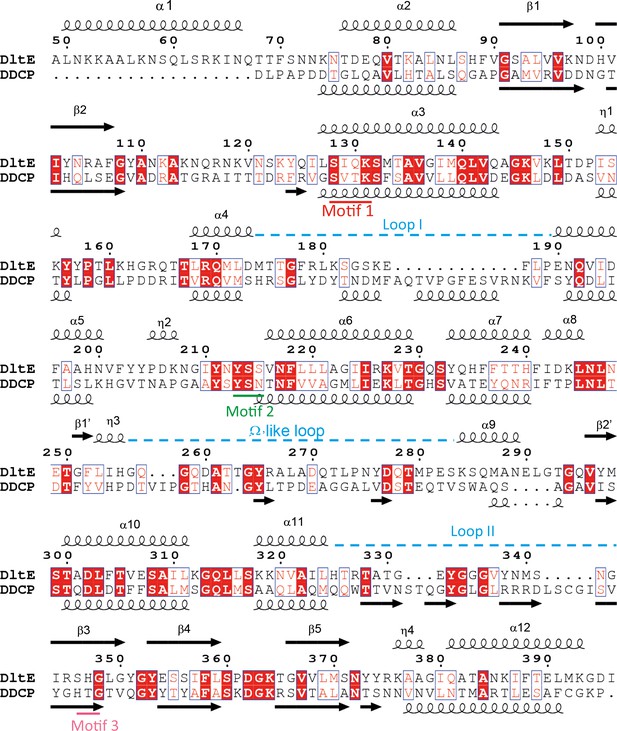
Sequence comparison of DltE with the Streptomyces R61 D-Ala-D-Ala carboxypeptidase (DDCP).
Sequence alignment between DltE (residues 49–396) from L. plantarum and DDCP. The two proteins share a relatively low-sequence identity of around 25%. The secondary structures extracted from the 3D X-ray structures are respectively depicted above (DltE, this study, PDB entry 8AJI) and below (DDCP, PDB entry 1HVB). Canonical serine-type D-alanyl-D-alanine carboxypeptidases sequences exhibit three conserved motifs that are highlighted on the figure. The S-X-X-K motif 1(in red) encompasses a nucleophilic Ser that forms a catalytic dyad with the Lys residue. The S/Y-X-N motif 2 (in green) and the K/H-T/S-G motif 3 (in pink) complete the active site. Three loop regions that define the catalytic cavity architecture and differ between the two sequences are indicated on the alignment: Loop I, Loop II, and the Ω-like loop. The figure was generated by ESPript (https://espript.ibcp.fr).
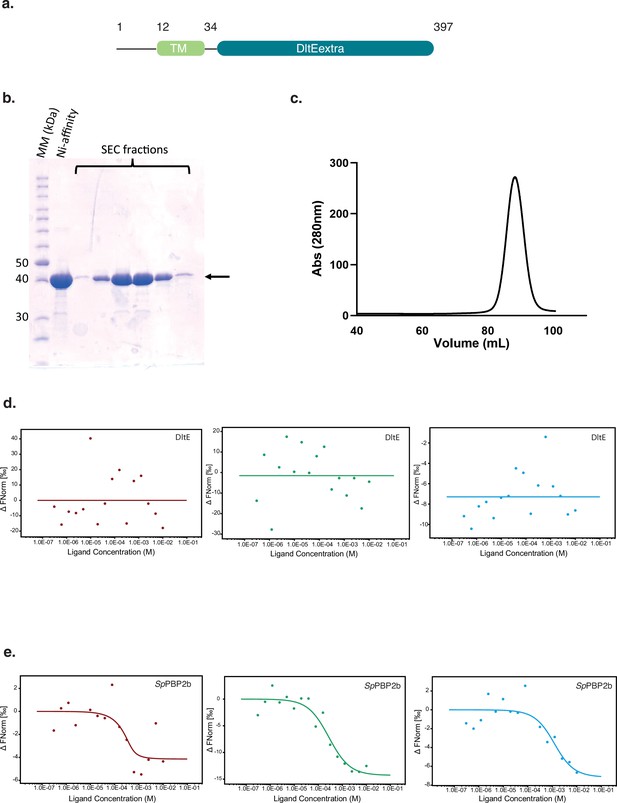
Production of DltEextra used for structure determination and biochemical assays.
(a) The dltE gene encodes for a 397 residues protein composed of a transmembrane segment predicted between residues 12 and 34 and a larger C-terminal extracellular region from residues 34–397 and named DltEextra. (b) SDS-PAGE analysis of the purity of DltEextra after a two-step purification procedure, including an Ni-Affinity and a size-exclusion chromatography (SEC). The band corresponding to DltEextra (41 kDa) is shown by an arrow. (c) SEC and elution profile after injection of the Ni-affinity-purified DltEextra on a Superdex 200 10/300 GL. (d, e) Microscale thermophoresis (MST) normalized dose–response curves for the binding interaction between penicillin and DltEextra (d) and S. pneumoniae PBP2b used as a positive control (e) were obtained by plotting ∆Fnorm against the ligand concentration. The data are representative of experiments made in triplicate. As expected from proteins from the PBP family, S. pneumoniae PBP2 binds to penicillin while no binding was detected for DltE.
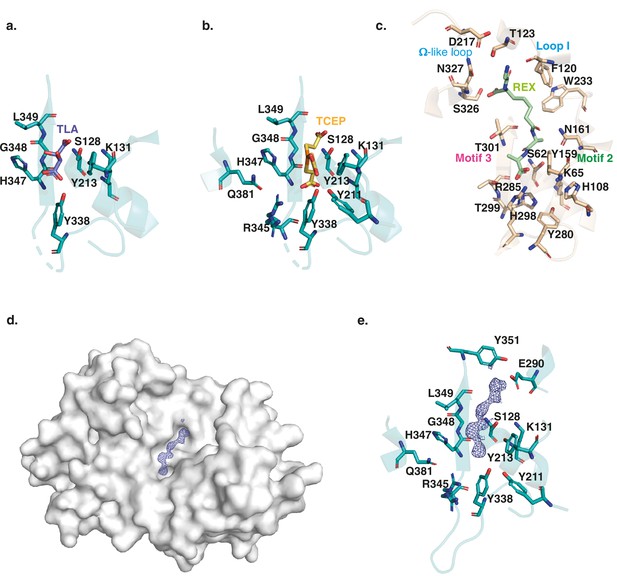
The substrate binding cleft of L. plantarum DltE.
Close-up on the ligand binding site of DltEextra crystallized in complex with a tartare (TLA) (a), or TCEP (Tris(2-carboxyethyl)phosphine hydrochloride) (b), molecule. The residues involved in the interactions are shown as sticks. The catalytic Ser128, with the hydroxyl group of its chain side is positioned at only 2.7 Å away from a carboxylic group of the ligand is itself hydrogen bonded to the catalytic Lys131 of the motif 1 and to Tyr213, the only conserved residue of the motif 2. The three residues of the β3-strand (347H-G-L349) including Gly348 from motif 3 delineate one side of the active site. Tyr338 establishes a strong, almost covalent, interaction with ligand carboxyl group that is also bound to Ser128. Three additional interactions specific to DltE are observed in the TCEP-bond structure and involved Tyr211, Arg345, and Gln381. (c) Close-up on the ligand binding site of DDCCP in complex with fragment of the cell wall precursor (REX – glycyl-L-alpha-amino-epsilon-pimelyl-D-Ala-D-Ala) (PDB ID 1IKG). The residues involved in the interactions are shown as sticks. The catalytic Ser62 and Lys65 from motif 1 and Tyr159 from motif 2 are located in the vicinity of the last D-Ala moiety in positions similar to those observed in DltE. The rest of the substrate binding site differs significantly. The Arg285 conserved in DDCP and responsible for the carboxypeptidase activity, recognizes the terminal carboxylate of the substrate. The interactions involving motif 2 and 3 stabilize the penultimate D-Ala residue. The hydrophobic subsite composed of Trp233 and Phe120 recognizes the aliphatic portion of the peptide and is also notably absent in DltE. Finally, H-bond interactions are established with the N-terminus of the peptide substrate at the level of the loop I and Ω-like-loop. (d) Surface representation of DltE structure obtained from crystal soaked with LTA molecules. A long electron density compatible with a 2-mer polyglycerol phosphate was observed lying in the catalytic cleft. (e) Close-up on the interaction network around the backbone of the ligand modeled in the catalytic cleft. The interaction network that stabilizes the first half of the ligand is the same as the one described with the TCEP molecules. The second half of the electron density extends further on the catalytic cleft of DltE in the vicinity of Glu290 (in helix α9) and Tyr351 (between β3 and β4) that are not conserved in canonical DDCP.
-
Figure 2—source data 1
DltE 3D structure in complex with tartare (TLA) or TCEP (Tris(2-carboxyethyl)phosphine hydrochloride).
- https://cdn.elifesciences.org/articles/84669/elife-84669-fig2-data1-v2.xlsx
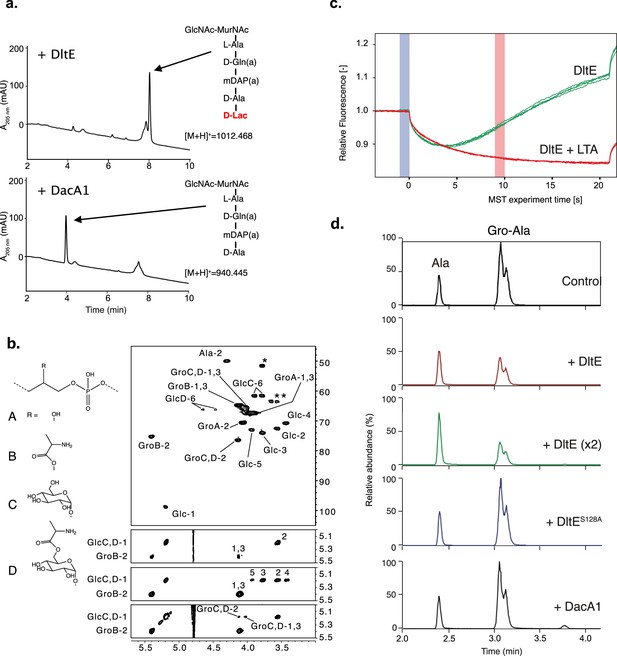
DltE is not active on peptidoglycan (PG) stem peptide but has D-Ala esterase activity on lipoteichoic acid (LTA).
(a) Test of carboxylesterase activity of DltE on disaccharide-depsipentapeptide substrate. Purified enzymes (DltEextra or DacA1) were incubated with purified muropeptide, and the mixture was analyzed by ultra-high-pressure liquid chromatography (UHPLC). DacA1 taken as a control is able to release terminal D-Lac, generating disaccharide-tetrapeptide. Muropeptides were identified by mass spectrometry. (b) Multidimensional NMR analysis of LTA isolated from WT L. plantarum established the presence of four major repeating units made of phospho-glycerol (Gro) differently substituted at C-2 position by -OH group (A), Ala- residue (B), αGlc residues (C), or Ala-6-αGlc group (D). Individual spin systems of Glc residues C and D, Gro associated to A-D and Ala associated to GroB and GlcD were established from 1H-13C HSQC (top), 1H-1H COSY (second from top), and 1H-1H TOCSY (third from top) spectra in agreement with literature (Sánchez Carballo et al., 2010). 1H and 13C chemical shifts are reported in Supplementary file 2. Ala residue was typified according to the 1H/13C chemical shifts of C1 at δ -/172.5, C2 at δ 4.29/49.9, and C3 at δ 1.63/16.36, out of which only Ala-2 is visible on the presented region of 1H-13C HSQC spectrum and Ala-1 was identified on 1H-13C HMBC spectrum (not shown). Substitution of Gro by Ala in C-2 position is established owing to the very unshielded GroB-2 signal at δ 5.40/75.3. Substitution of GroC,D in C-2 position was established owing to the deshielding of GroC,D-2 13C at δ 76.4 compared to unsubstituted GroA-2 13C at δ 70.6 and the 1H-1H NOESY cross signal between GlcC,D-1 and GroC,D-2 (bottom spectrum). Finally, substitution of GlcD in C-6 position is observable on the 1H-13C HSQC spectrum by the strong deshielding of GlcD-6 at δ 4.45–4.63/66.2 compared to unsubstituted GlcC-6 at δ 3.77–3.88/61.556. Such LTAs have never been identified in L. plantarum, but very similar repetition units were previously characterized in LTA and wall teichoic acid (WTA) isolated from Lactobacillus brevis (Sánchez Carballo et al., 2010). However, in contrast to L. brevis in which WTA and LTA showed very similar Gro-based sequences, WTA and LTA from L. plantarum showed very different structures with repetition units based either on Rbo-phosphate for the former and on Gro-phosphate for the latter. (c) Microscale thermophoresis (MST) traces for DltEextra without and with purified LTA from LpNC8 added at a concentration of 500 µM. Relative fluorescence change reveals binding of LTA to DltEextra (Fhot: fluorescence at the region defined as hot 10 s after IR laser heating, Fcold: fluorescence at the region defined as cold at 0 s). (d) Test of D-Ala esterase activity of DltE on LTA from LpNC8. Purified LTA (100 µg) was incubated with purified enzymes: DltEextra (100 µg or 200 µg [×2]), DltEextraS128A (100 µg) or DacA1 (100 µg). Control corresponds to LTA incubated in the same conditions without enzyme. After LTA depolymerization by HF treatment, hydrolysis products were analyzed by LC-MS/MS. Ala and Gro-Ala were identified by their m/z values (90.05 and 164.09, respectively) and MS-MS spectra (Figure 3—figure supplement 5). Gro-Ala was detected as a double peak, corresponding possibly to the migration of D-Ala from C2 to C1 of Gro (Morath et al., 2001). A chromatogram of the combined extracted ion chromatograms of each target ion species was generated for each condition. Similar results were obtained in two independent experiments.
-
Figure 3—source data 1
Microscale thermophoresis (MST) data for the binding of DltEextra with purified lipoteichoic acid (LTA) from LpNC8.
- https://cdn.elifesciences.org/articles/84669/elife-84669-fig3-data1-v2.xlsx
-
Figure 3—source data 2
DltE has D-Ala esterase activity on lipoteichoic acid (LTA).
- https://cdn.elifesciences.org/articles/84669/elife-84669-fig3-data2-v2.xlsx
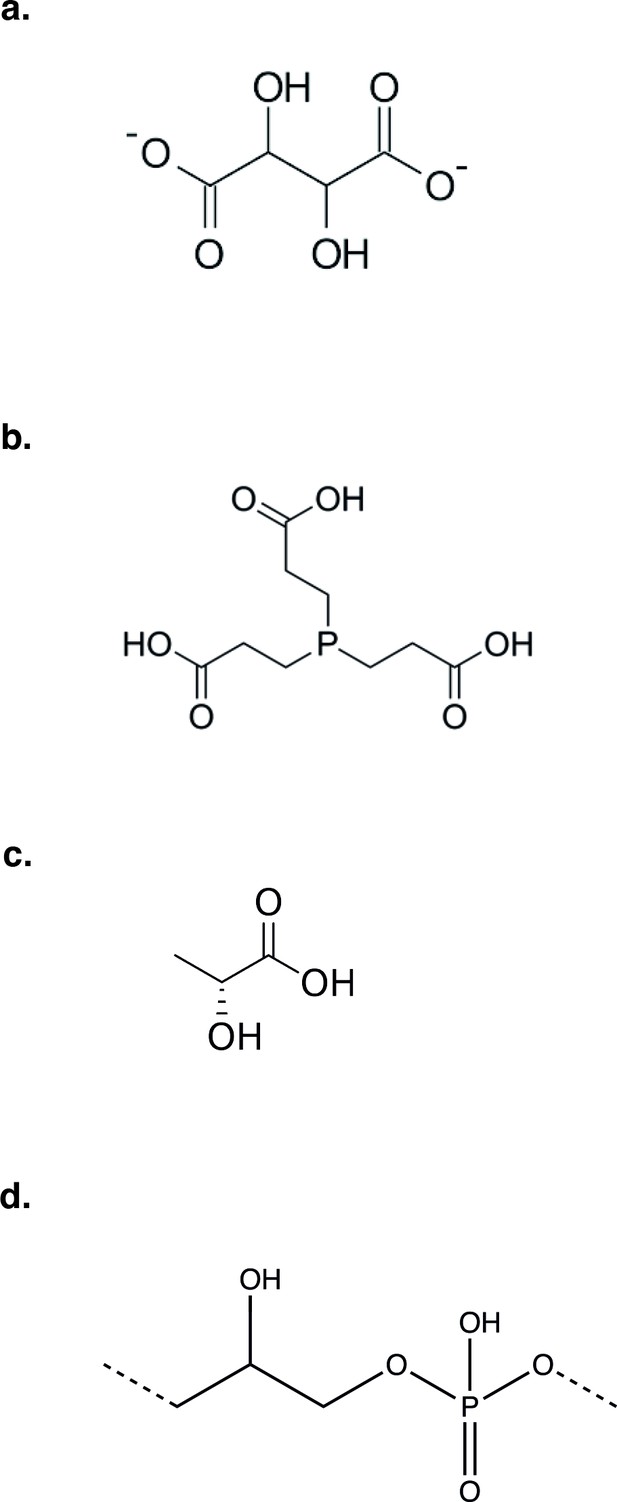
Chemical structures of (a) tartrate, (b) TCEP Tris(2-carboxyethyl)phosphine hydrochloride, (c) D-Lac, and (d) subunit of Lp lipoteichoic acid (LTA) molecule without any substitution.
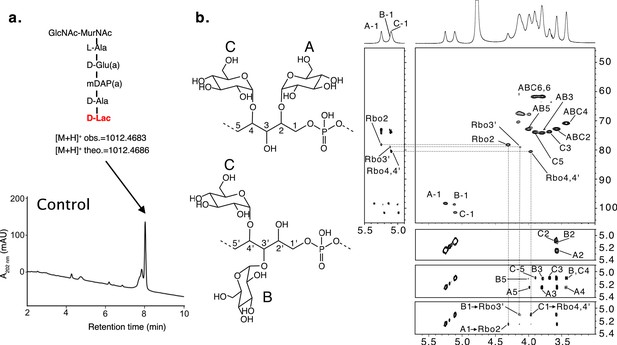
Purification of the disaccharide-depsipentapeptide from Lp NC8 dacA1dacA2 mutant and determination of Lp NC8 WTA chemical structure by NMR.
(a) Ultra-high-pressure liquid chromatography (UHPLC) analysis of the disaccharide-depsipentapeptide purified from peptidoglycan (PG) of LpNC8 ∆dacA1A2 mutant digested by mutanolysin. The muropeptide was identified by MS analysis. This muropeptide was used in activity tests of purified enzymes (DltE and DacA1) as shown in Figure 3a. (b) Multidimensional NMR analysis of wall teichoic acid (WTA) isolated from WT Lp established the presence of two major repeating units made of phospho-ribitol (Rbo) differently substituted by Glc residues in C-2 position (residue A), C-3 position (residue B), and C-4 position (residue C) as shown on the left side. Individual spin systems of Glc residues A-C and Rbo positions 1–5 and 1’–5’ were established from1H-13C HSQC (top left right), 1H-1H COSY (second from top right), and 1H-1H TOCSY (third from top right) spectra in agreement with literature that identified similar compounds from the cell wall of several strains of Lp (Tomita et al., 2013; Tomita et al., 2009). Linkage of αGlc residues A, B, and C to positions 2, 3’, and 4,4’ of Rbo, respectively, was assigned from 1H-1H NOESY (bottom, right) and 1H-13C HMBC (top left) spectra as shown by the cross-correlation signals.
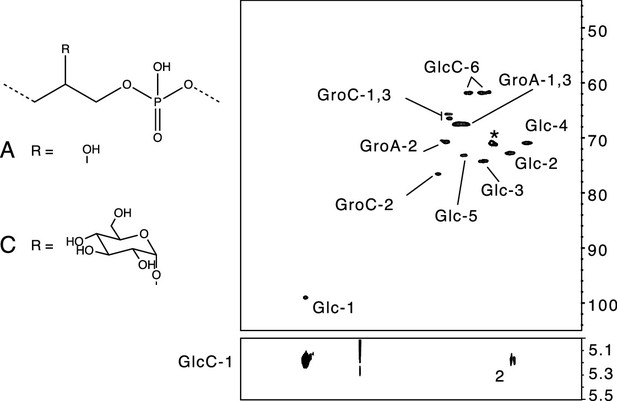
Lipoteichoic acid (LTA) extracted from ΔdltXABCD mutant is devoid of Ala.
Multidimensional NMR analysis of LTA isolated from ΔdltXABCD mutant of L. plantarum established the presence of two major repeating units made of phospho-glycerol (Gro) differently substituted by at C-2 position either by -OH group (A) or by αGlc residues (C). Comparison of 1H-13C HSQC (top) and 1H-1H COSY (bottom) spectra of LTA from WT (Figure 3b) and ΔdltXABCD mutant showed a total absence of NMR signals associated to Ala-substituted LTA in the latter.
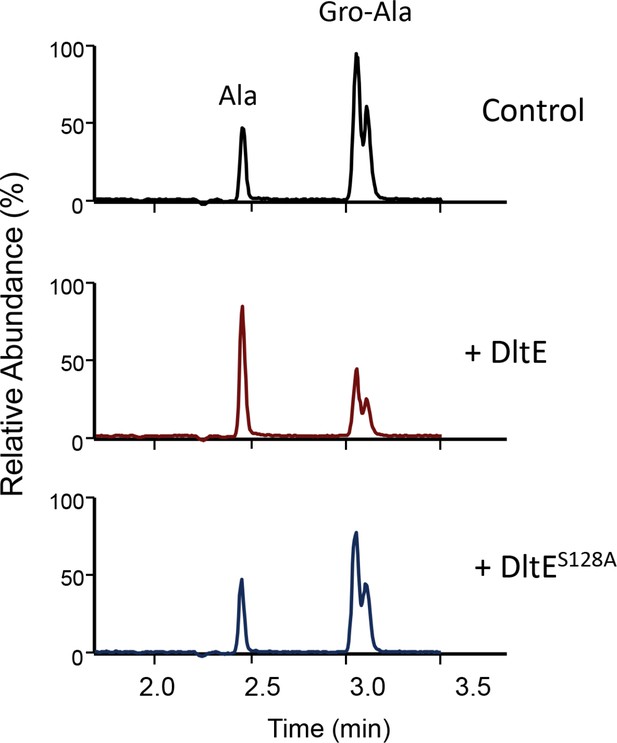
Experimental replicates from Figure 3d.
Test of D-Ala esterase activity of DltE on lipoteichoic acid (LTA) from LpNC8. Purified LTA (100 µg) was incubated with purified enzymes: DltEextra (100 µg) or DltEextraS128A (100 µg). Control corresponds to LTA incubated in the same conditions without enzyme. After LTA depolymerization by HF treatment, hydrolysis products were analyzed by LC-MS/MS. Ala and Gro-Ala were identified by their m/z values (90.05 and 164.09, respectively) and MS-MS spectra (Figure 3—figure supplement 5). Gro-Ala was detected as a double peak, corresponding possibly to the migration of D-Ala from C2 to C1 of Gro (Morath et al., 2001). A chromatogram of the combined extracted ion chromatograms of each target ion species was generated for each condition.
-
Figure 3—figure supplement 4—source data 1
Identification of Ala and Gro-Ala by MS and MS/MS.
- https://cdn.elifesciences.org/articles/84669/elife-84669-fig3-figsupp4-data1-v2.xlsx
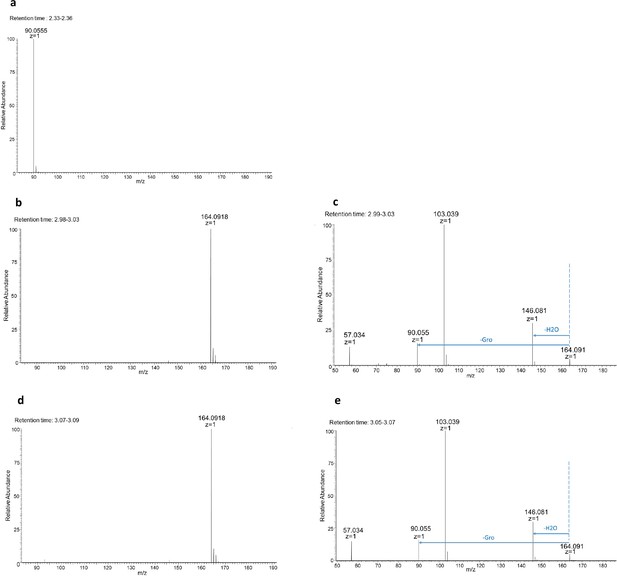
MS and MS/MS specta of Ala and Gro-Ala.
(a, b, d) MS spectra of (a) Ala (retention time 2.33–2.36) (calculated [M + H] + 90.0550), (b, d) Gro-Ala (retention time 2.98–3.03 and 3.07–3.09) (calculated [M + H] + 164.0918). fcCorresponding chromatograms are shown in Figure 3d. (c, e), MS/MS spectra for peaks at retention time 2.98–3.03 and 3.07–3.09, confirming that both correspond to Gro-Ala.
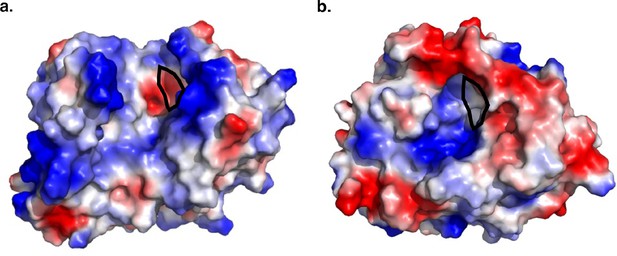
Molecular surfaces of DltEextra (a) and DDCP (b), colored according to the electrostatic potential.
Residues are colored in blue and red according to positive and negative electrostatic potential, respectively. The apolar residues are colored white. The hydrophobic subsite suggested in DDCP to recognize the aliphatic portion of the peptide substrate is encircled in black (b). The corresponding region is marked as well in DltE (a) but is not conserved. It is replaced by mainly charged residues from the Ω-like loop and helix α9.
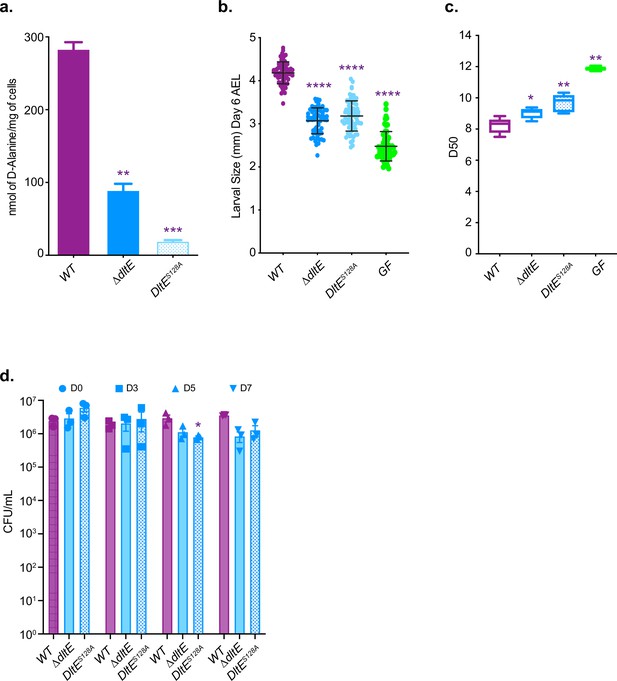
The D-Ala esterase activity of DltE contributes to D-alanylation of the cell envelope and is required to sustain Drosophila juvenile growth.
(a) Amount of D-Ala released from whole cells of WT, ∆dltE, and DltES128A by alkaline hydrolysis and quantified by HPLC. Mean values were obtained from three independent cultures with two injections for each. Purple asterisks illustrate statistically significant difference with D-Ala release from WT. ***0.0001<p<0.001; **0.001<p<0.01. (b) Larval longitudinal length after inoculation with WT, ∆dltE, and DltES128A strains and PBS (for the germ-free [GF] condition). Larvae were collected 6 d after association and measured as described in the ‘Materials and methods’ section. Purple asterisks illustrate statistically significant difference with larval size of WT; ****p<0.0001. Center values in the graph represent means and error bars represent SD. Representative graph from one out of three independent experiments. (c) Time to pupation: day when 50% of pupae emerge during a developmental experiment (D50) for GF eggs associated with strains WT, ∆dltE, and DltES128A or PBS (for the GF condition). Center values in the graph represent means. Purple asterisks illustrate statistically significant difference with WT larval size; **0.001<p<0.01; *p<0.05. (d) Abundance of colony-forming units (CFUs) on fly food and larvae at days 3, 5, and 7 after inoculation with WT, ∆dltE, and DltES128A. Purple asterisks illustrate statistically significant difference with WT within each day. *p<0.05.
-
Figure 4—source data 1
The D-Ala esterase activity of DltE contributes to D-alanylation of the cell envelope and is required to sustain Drosophila juvenile growth.
- https://cdn.elifesciences.org/articles/84669/elife-84669-fig4-data1-v2.xlsx
-
Figure 4—source data 2
The D-Ala esterase activity of DltE is required to sustain Drosophila juvenile growth – replicates.
- https://cdn.elifesciences.org/articles/84669/elife-84669-fig4-data2-v2.xlsx
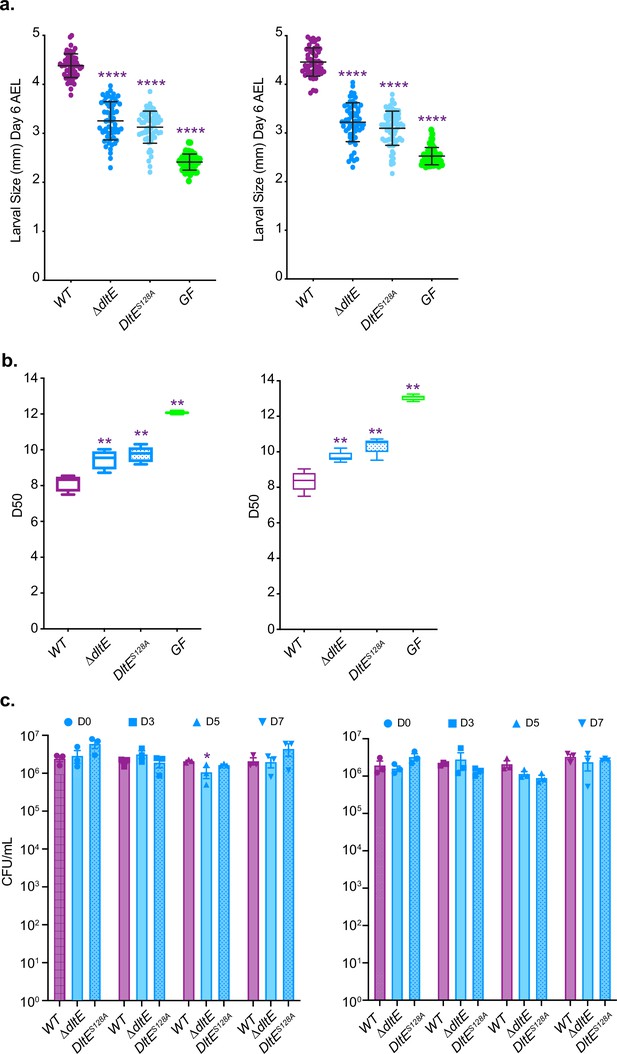
The D-Ala esterase activity of DltE is required to sustain Drosophila juvenile growth.
(a) Experimental replicates from Figure 4b: larval longitudinal length after inoculation with WT, ∆dltE, and DltES128A strains and PBS (for the germ-free [GF] condition). Larvae were collected 6 d after association and measured as described in the ‘Materials and methods’ section. Purple asterisks illustrate statistically significant difference with larval size of WT; ****p<0.0001. Center values in the graph represent means and error bars represent SD. (b) Experimental replicates from Figure 4c: day when 50% of pupae emerge during a developmental experiment (D50) for GF eggs associated with strains WT, ∆dltE and DltES128A or PBS (for the GF condition). Center values in the graph represent means. Purple asterisks illustrate statistically significant difference with WT larval size; **0.001<p<0.01. (d) Experimental replicates from Figure 4d: abundance of colony-forming units (CFUs) on fly food and larvae at days 3, 5, and 7 after inoculation with WT, ∆dltE, and DltES128A. Purple asterisks illustrate statistically significant difference with WT within each day. *p<0.05.
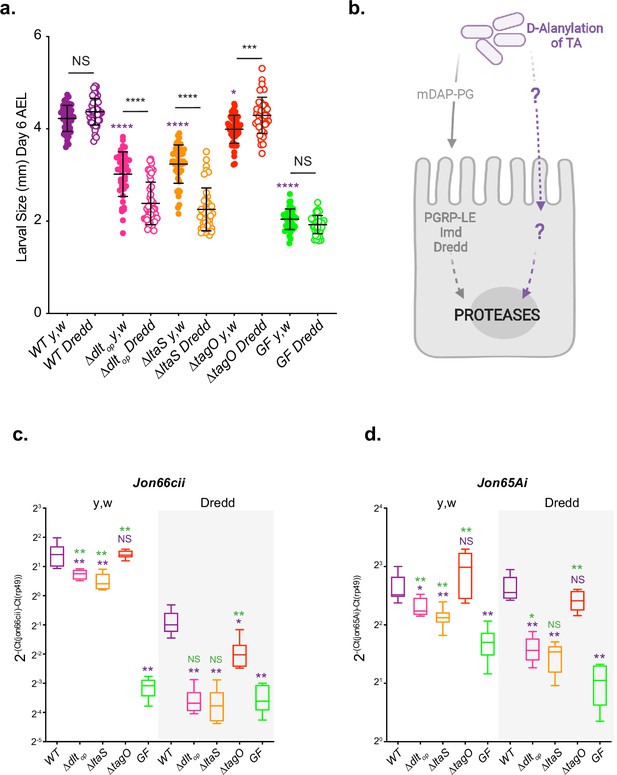
D-Ala-LTAs are necessary bacterial cues supporting Drosophila intestinal response and juvenile growth.
(a) y,w and y,w,Dredd larvae longitudinal length after inoculation with 108 colony-forming units (CFUs) of WT, ∆dltop, ∆ltaS, and PBS, for the germ-free condition. Larvae were collected 6 d after association and measured as described in the ‘Materials and methods’ section. The purple asterisks represent a statistically significant difference compared with WT larval size. The bars in the graph represent means and SD. NS represents the absence of a statistically significant difference; ****p<0.000; ***0.0001<p<0.001; *p<0.05. Representative graph from one out of three independent experiments. (b) Working model for Lp detection in Drosophila enterocytes: Drosophila sense and signal the presence of Lp cells through: (1) PGRP-LE-mediated mDAP-PG fragment recognition triggering Imd/Dredd signaling and (2) sensing of bacterial cell envelope bearing D-alanylated teichoic acids and signaling by unknown host mechanisms. Both signals were reported to be important for maximal intestinal peptidase expression. (c, d) Mean ± SD of 2-∆Ctgene/∆Ctrp49 ratios for Jon66Cii and Jon65Ai detected in dissected guts of y,w and y,w,Dredd associated with WT, ∆dltop, ∆ltaS or the GF condition from five biological replicates. Representative graphs from one out of three independent experiments are shown. The purple asterisks represent a statistically significant difference compared with LpNC8 proteases expression. The green asterisks represent a statistically significant difference compared with the GF condition. NS represents the absence of a statistically significant difference compared to the GF condition or LpNC8. **0.001<p<0.01; *p<0.05.
-
Figure 5—source data 1
D-Ala-LTAs are necessary bacterial cues supporting Drosophila intestinal response and juvenile growth.
- https://cdn.elifesciences.org/articles/84669/elife-84669-fig5-data1-v2.xlsx
-
Figure 5—source data 2
D-Ala quantification and persistence on fly niche of WT, ∆dltop, ∆ltaS, and ∆tagO strains.
- https://cdn.elifesciences.org/articles/84669/elife-84669-fig5-data2-v2.xlsx
-
Figure 5—source data 3
D-Ala-LTAs are necessary bacterial cues supporting Drosophila intestinal response and juvenile growth – replicates.
- https://cdn.elifesciences.org/articles/84669/elife-84669-fig5-data3-v2.xlsx
-
Figure 5—source data 4
Replicate western blot detection of lipoteichoic acid (LTA) in wild-type LpNC8 and mutant derivatives.
- https://cdn.elifesciences.org/articles/84669/elife-84669-fig5-data4-v2.pdf
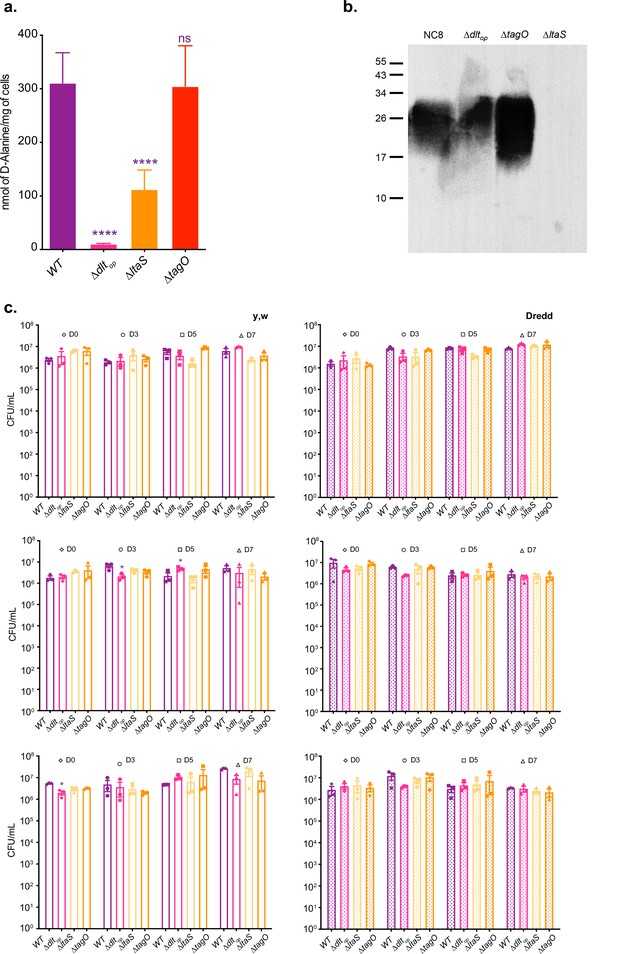
D-Ala content and colony forming units of strains used on Figure 5.
(a) Amount of D-Ala released from whole cells of WT, ∆dltop, ∆ltaS, and ∆tagO by alkaline hydrolysis and quantified by HPLC. Mean values were obtained from three independent cultures with two injections for each. Purple asterisks illustrate statistically significant difference with D-Ala released from WT. NS represents the absence of a statistically significant difference compared to WT. ****p<0.0001. (b) Western blot detection of lipoteichoic acid (LTA) in wild-type LpNC8 and mutant derivatives. (c) Abundance of colony-forming units (CFUs) on fly food and larvae at days 3, 5, and 7 after inoculation with WT, ∆dltop, ∆ltaS, and ∆tagO in y,w and y,wDredd flies. Each graph represents an independent experiment. Purple asterisks illustrate statistically significant difference with WT within each day. *p<0.05.
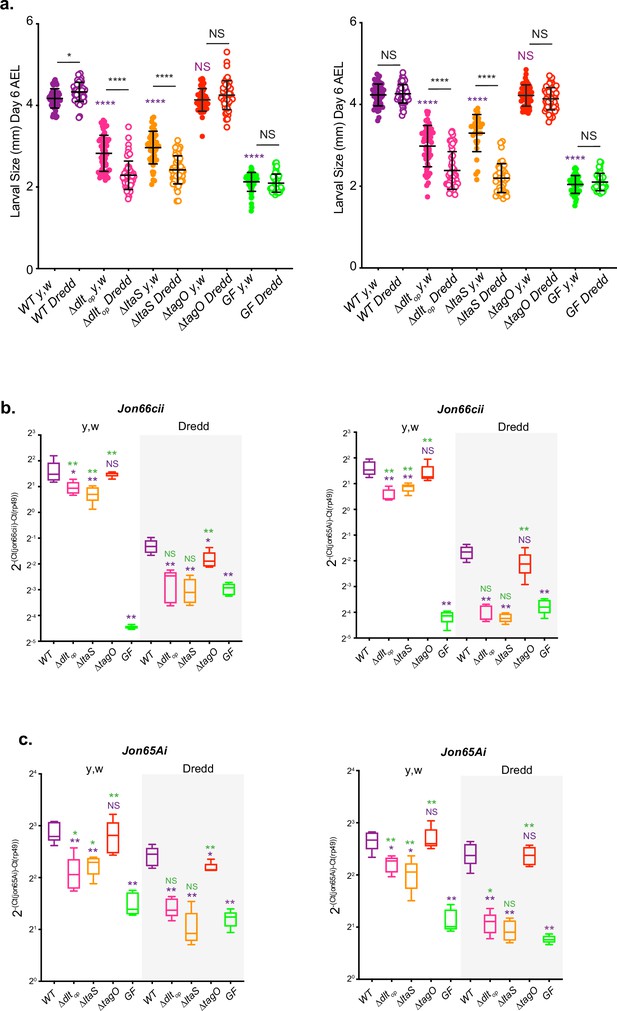
D-Ala-LTAs are necessary bacterial cues supporting Drosophila intestinal response and juvenile growth.
(a) Experimental replicates from Figure 5a: y,w and y,w,Dredd larvae longitudinal length after inoculation with 108 colony-forming units (CFUs) of WT, ∆dltop, ∆ltaS, and PBS for the germ-free (GF) condition. Larvae were collected 6 d after association and measured as described in the ‘Materials and methods’ section. The purple asterisks represent a statistically significant difference compared with WT larval size. The bars in the graph represent means and SD. NS represents the absence of a statistically significant difference; ****p<0.0001; *p<0.05. (b, c) Experimental replicates from Figure 5c and d: Mean ± SD of 2-∆Ctgene/∆Ctrp49 ratios for Jon66Cii and Jon65Ai detected in dissected guts of y,w and y,w,Dredd associated with WT, ∆dltop, ∆ltaS or the GF condition from five biological replicates. The purple asterisks represent a statistically significant difference compared with WT proteases expression. The green asterisks represent a statistically significant difference compared with the GF condition. NS represents the absence of a statistically significant difference compared to the GF condition or WT. **0.001<p<0.01; *p<0.05.
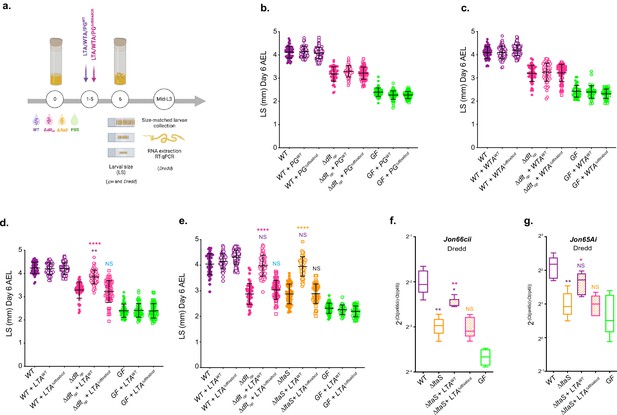
D-Ala lipoteichoic acids (LTAs) are bacterial cues supporting Drosophila intestinal response and juvenile growth.
(a) Experimental set-up to test the impact of cell envelope components on Drosophila growth and proteases expression: germ-free (GF) eggs were inoculated with WT, ∆dltop, ∆ltaS, or PBS for the GF condition and supplemented daily (for 5 d) with LTA, wall teichoic acid (WTA), and peptidoglycan (PG) extracted from WT or ∆dltXABCD strains. 1 µg of each component purified from WT was used. For comparison with components extracted ΔdltXABCD strain, the final PG, WTA, and LTA suspensions were adjusted to the same amount of Gro, Rbo, and Mur, respectively. At day 6 after inoculation, larvae were harvested and measured (see the ‘Materials and methods’ section for details). Mid-L3 sized-matched larvae were collected for each condition. Their guts were dissected followed by RNA extraction and RT-qPCR targeting proteases expression. (b–d) Larval longitudinal length after inoculation with strains WT, ∆dltop, PBS, and purified PG (PG) (b) WTA (c) or LTA (d) from WT or ∆dltXABCD. Larvae were collected 6 d after the first association and measured as described in the ‘Materials and methods’ section. The pink asterisks represent a statistically significant difference compared with ∆dltop larval size; purple asterisks represent a statistically significant difference compared with WT larval size. NS represents the absence of a statistically significant difference compared to ∆dltop. ****p<0.0001; **0.001<p<0.01. The bars in the graph represent means and SD. A representative graph from one out of three independent experiments is shown. (e) Larval longitudinal length after inoculation with strains WT, ∆dltop, ∆ltaS, or PBS and purified LTA from WT or ∆dltXABCD strains. Larvae were collected 6 d after the first association and measured as described in the ‘Materials and methods’ section. The pink asterisks represent a statistically significant difference compared with ∆dltop larval size; purple asterisks represent a statistically significant difference compared with WT larval size ****p<0.0001; **0.001<p<0.01. The bars in the graph represent means and SD. A representative graph from one out of three independent experiments is shown. (f, g), Mean ± SD of 2-∆Ctgene/∆Ctrp49 ratios for Jon66Cii and Jon65Ai detected in dissected guts of y,w,Dredd size-matched larvae, associated with LpNC8, ∆dltop, ∆ltaS or the GF and LTA from WT or ∆dltXABCD strains, from five biological replicates. Representative graphs from one out of three independent experiments are shown. The purple asterisks represent a statistically significant difference compared with LpNC8 protease expression. The pink asterisks represent a statistically significant difference compared with ∆ltaS supplemented with LTA from ∆dltXABCD strain. NS represents the absence of a statistically significant difference compared to ∆ltaS condition. **0.001<p<0.01; *p<0.05.
-
Figure 6—source data 1
D-Ala lipoteichoic acids (LTAs) are bacterial cues supporting Drosophila intestinal response and juvenile growth.
- https://cdn.elifesciences.org/articles/84669/elife-84669-fig6-data1-v2.xlsx
-
Figure 6—source data 2
Experimental replicates from Figure 6b–d.
- https://cdn.elifesciences.org/articles/84669/elife-84669-fig6-data2-v2.xlsx
-
Figure 6—source data 3
Experimental replicates from Figure 6f and g.
- https://cdn.elifesciences.org/articles/84669/elife-84669-fig6-data3-v2.xlsx
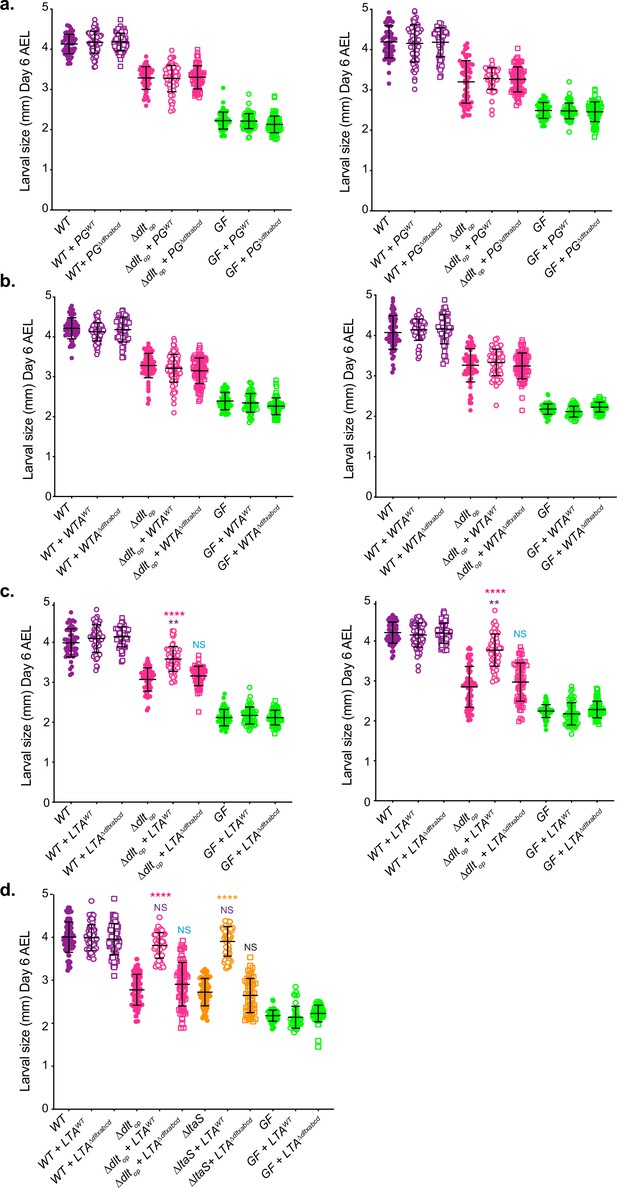
Experimental replicates from Figure 6b-d.
(a–d) Larval longitudinal length after inoculation with strains WT, ∆dltop, PBS, and purified peptidoglycan (PG) (a), wall teichoic acids (WTA) (b), or lipoteichoic acids (LTA) (c) from LpNC8 or ∆dltXABCD. Larvae were collected 6 d after the first association and measured as described in the ‘Materials and methods’ section. The pink asterisks represent a statistically significant difference compared with ∆dltop larval size; purple asterisks represent a statistically significant difference compared with WT larval size. NS represents the absence of a statistically significant difference compared to ∆dltop. ****p<0.0001; **0.001<p<0.01. The bars in the graph represent means and SD. (d) Experimental replicate from Figure 6e: larval longitudinal length after inoculation with strains WT, ∆dltop, ∆ltaS, or PBS and purified LTA from WT or ∆dltXABCD strains. Larvae were collected 6 d after the first association and measured as described in the ‘Materials and methods’ section. The pink asterisks represent a statistically significant difference compared with ∆dltop larval size; purple asterisks represent a statistically significant difference compared with WT larval size ****p<0.0001; **0.001<p<0.01. The bars in the graph represent means and SD.
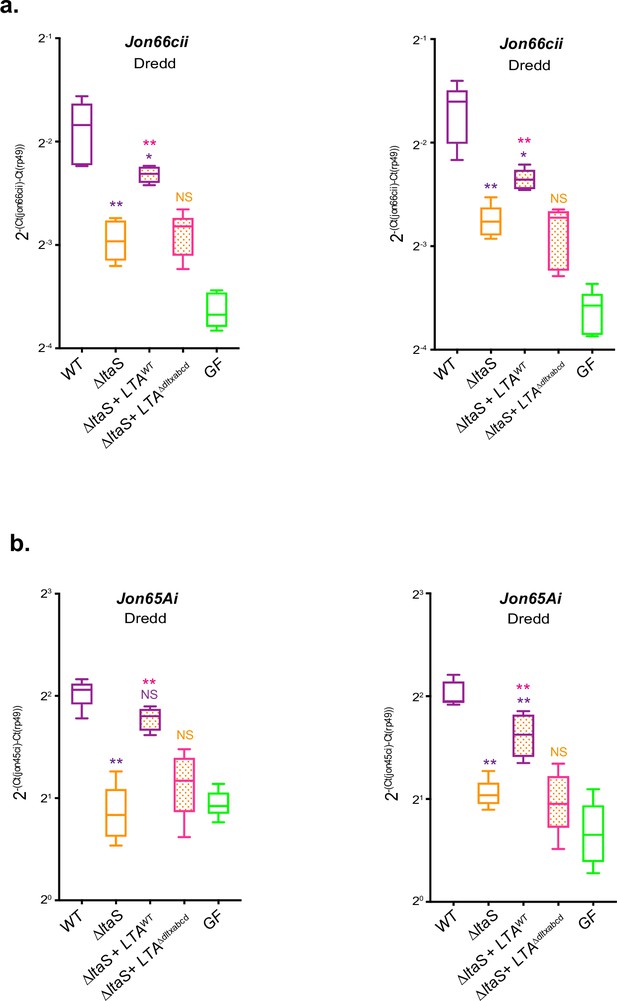
Experimental replicates from Figure 6f and g.
(a, b) mean ± SD of 2-∆Ctgene/∆Ctrp49 ratios for Jon66Cii and Jon65Ai detected in dissected guts of y,w,Dredd size-matched larvae, associated with WT, ∆dltop, ∆ltaS or the germ-free (GF) and lipoteichoic acid (LTA) from WT or ∆dltXABCD strains, from five biological replicates. The purple asterisks represent a statistically significant difference compared with WT protease expression. The pink asterisks represent a statistically significant difference compared with ∆ltaS supplemented with LTA from ∆dltXABCD strain. NS represents the absence of a statistically significant difference compared to ∆ltaS condition. **0.001<p<0.01; *p<0.05.
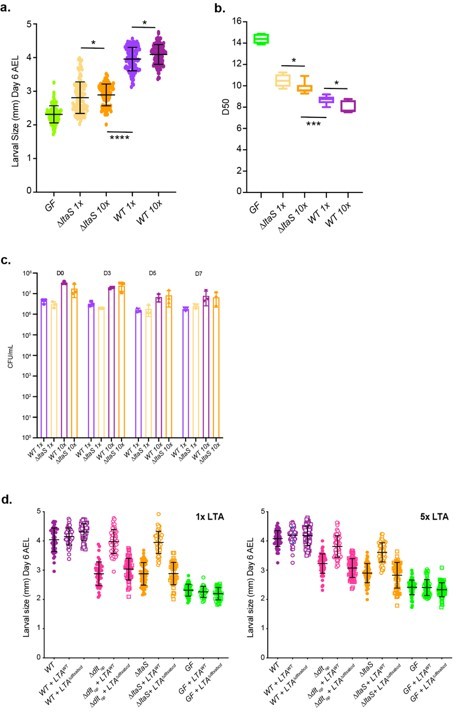
(a) Larval size (corresponding to larval longitudinal length at day 6 after egg laying) (b) time to pupation (D50, corresponding to the day in which 50% of the larvae pupate) of eggs associated with different concentrations of WT and DltaS strains.
1x corresponds to 108 CFUs while 10x corresponds to 109 CFUs. (c) Number of CFUs on fly food and larvae at days 3, 5, and 7 after inoculation with WT and ∆ltaS at different concentrations (D0) of L1 larvae: 1x corresponds to 108 CFUs while 10x corresponds to 109 CFUs. (d) Larval longitudinal length after inoculation with strains WT, ∆dltop, ∆ltaS or PBS and different amounts of purified lipoteichoic acid (LTA) from WT or ∆dltXABCD strains. On the right panel we used 5x more purified LTA than on the left panel (Panel from the main manuscript). Larvae were collected six days after the first association and measured as described in the Methods.
Additional files
-
Supplementary file 1
Data collection and refinement statistics.
- https://cdn.elifesciences.org/articles/84669/elife-84669-supp1-v2.docx
-
Supplementary file 2
Proton and carbon chemical shifts of Glc, Gro, and Ala constituents of lipoteichoic acid (LTA) purified from L. plantarum WT.
- https://cdn.elifesciences.org/articles/84669/elife-84669-supp2-v2.docx
-
Supplementary file 3
Primers used in this study for Lp genetic manipulation.
- https://cdn.elifesciences.org/articles/84669/elife-84669-supp3-v2.docx
-
Supplementary file 4
Bacterial strains and plasmids used in this study.
- https://cdn.elifesciences.org/articles/84669/elife-84669-supp4-v2.docx
-
Supplementary file 5
Primers used for E. coli plasmid constructions.
- https://cdn.elifesciences.org/articles/84669/elife-84669-supp5-v2.docx
-
Supplementary file 6
Plasmids used in this study for protein expression.
- https://cdn.elifesciences.org/articles/84669/elife-84669-supp6-v2.docx
-
MDAR checklist
- https://cdn.elifesciences.org/articles/84669/elife-84669-mdarchecklist1-v2.pdf