Brain Communication: What calcium channels remember
Computers are often compared to brains. Both receive input from the outside world, which is then analyzed and transformed into a reaction. Yet, information processing in our brains and computers is fundamentally different (Nagarajan and Stevens, 2008). Unlike computers, which solely rely on digital signals to process information (i.e., values can only be 1 or 0), neurons in human brains use a mix of analogue (signals of varying values) and digital coding to communicate effectively.
Neurons receive input from other neurons through their cell bodies and dendrites at one end and transmit information through wirelike axons at the other end. In the classical view, information transfer in the form of neurotransmitters on the dendritic end would fluctuate continuously (representing a continuous but varying analogue signal), until a voltage threshold would be reached that causes axons to fire electrical impulses (also known as an all-or-none action potentials). This would then lead to the release of neurotransmitters onto the next neuron. So, while signals in the dendrites are represented in an analog fashion, they were thought to be transmitted in a digital way along the axon (Figure 1A left).
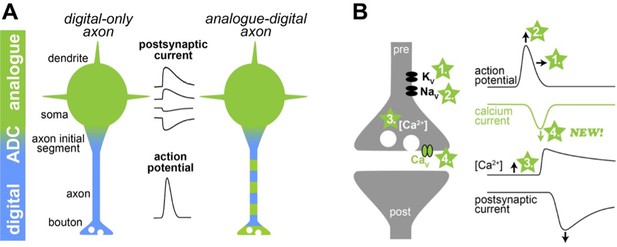
Illustration of a new mechanism of analogue-digital signaling.
(A) Left: Neurons were thought to receive analogue input signals (green) in the form of gradual, postsynaptic potentials. These were then converted into digital output signals (blue) in the form of action potentials via an analogue-digital converter (ADC). Right: However, previous research has shown that axons can use a combination of analogue-digital signaling in the axon (green-blue dashed color). (B) Left: Schematic illustration of a synapse (pre and post synapse in grey) with voltage-dependent potassium, sodium and calcium channels (Kv, Nav, and Cav, respectively). Four mechanisms mediating analogue-digital signaling are indicated. Right: Presynaptic action potential, calcium current, residual calcium concentration, and the postsynaptic current are shown versus time (modified from Geiger and Jonas, 2000). In the proposed fourth mechanism by Trigo and Kawaguchi, analogue signals directly affect the presynaptic calcium current.
However, it was later shown that subthreshold analogue signals propagate several hundreds of micrometers along an axon and modulate action potential-evoked transmitter release at the synapses (Alle and Geiger, 2006; Shu et al., 2006). This may enhance the computational abilities of neurons (Figure 1A right). However, the mechanisms mediating analogue-digital signaling in axons remain controversial.
Previous studies have suggested that analogue-digital signaling can modify neurotransmitter release in three ways (Figure 1B; for an extensive review and other potential mechanisms see Zbili and Debanne, 2019). First, analogue signals with a positive potential can increase the duration of the action potentials by inactivating voltage-dependent potassium channels. Second, positive analogue signals can increase the amplitude of the action potentials by keeping voltage-dependent sodium channels active under certain conditions. A longer and larger action potential opens voltage-dependent calcium channels more efficiently and thereby increases neurotransmitter release. Third, positive analogue signals can also increase the resting calcium concentration inside the synapse, leading to a higher probability that neurotransmitter will be released.
Now, in eLife, Federico Trigo (Instituto de Investigaciones Biológicas Clemente Estable, Uruguay) and Shin-ya Kawaguchi (Kyoto University, Japan) report on a new mechanism explaining analogue-digital signaling in the brain (Trigo and Kawaguchi, 2023). The researchers combined their expertise in sub-cellular patch-clamp recordings to analyze the mechanisms of analogue-digital signaling in both acutely prepared brain slices of rats and in neurons grown in cell culture. This enabled them to simultaneously record signals from the cell body of the neuron and from the axon terminals of a specific type of neuron, known as cerebellar molecular layer interneuron.
During the presynaptic patch-clamp recordings, the researchers applied recorded action potentials as voltage commands. They discovered that weak and short positive potentials (resembling the analogue signals of synapses) before an action potential increased both calcium influx into the axon and the release of neurotransmitters. This was surprising since the waveform of the presynaptic action potential was identical. Therefore, the first two mechanisms described above (which involved changes in the duration or amplitude of the action potential) cannot explain the results.
To address if the third mechanism involving an elevated resting calcium concentration can explain their results, Trigo and Kawaguchi interrupted the positive potentials before the action potentials for only a few milliseconds. Such a short interruption should not affect the resting calcium concentration. Yet, the analogue modulation was abolished, indicating that the third mechanism cannot explain the results either.
With the help of computer models, the researchers could demonstrate that analogue signals directly modulate calcium channels by increasing their readiness to open. This transition depends on voltage but is not mediated by the calcium-dependent modulation of calcium channels (Lin et al., 2012). In other words, the analogue signal switches the channels into a primed state, which facilitates opening once the action potential arrives. The calcium channels thus ‘remember’ the analogue signal until the action potential invades the synapse. However, when analogue signals are briefly interrupted, the channels rapidly revert to the resting conformation state, which prevents the analogue modulation of the calcium channels. A similar mechanism has previously been found in presynaptic potassium channels (Yang et al., 2014).
The study by Trigo and Kawaguchi raises several interesting points. Their recordings were mainly performed in immature neurons, but the properties of presynapses change profoundly as neurons mature (Taschenberger and von Gersdorff, 2000). It remains to be seen if analogue-digital signaling changes during maturation. Synapses are also extremely diverse, and more research is needed to see if the new mechanism is a specialized feature of interneurons in the cerebellar circuitry or would also apply to other neurons (Nusser, 2018). Interestingly, some synapses in the auditory pathway have remarkable stable presynaptic action potentials in vivo, which would allow analogue signaling to be mediated by intermediate gating states of calcium channels (Sierksma and Borst, 2017). Moreover, axons can sometimes emerge from dendrites, and it is unknown if analogue-digital signaling would be increased in these cases (Kole and Brette, 2018). Finally, intermediate gating states could be an important modulation of the complete failure of action potential propagation (Kawaguchi and Sakaba, 2015).
In summary, Trigo and Kawaguchi have performed a series of technically challenging experiments that revealed a new mechanism of analogue-digital signal transmission in the axon. This demonstrated that axons are more than simple digital conduction devices. Instead, past analogue signals in the axon modulate synaptic output to which the intermediate gating states of calcium channels contribute.
References
-
The electrical significance of axon location diversityCurrent Opinion in Neurobiology 51:52–59.https://doi.org/10.1016/j.conb.2018.02.016
-
How does the speed of thought compare for brains and digital computers?Current Biology 18:R756–R758.https://doi.org/10.1016/j.cub.2008.06.043
-
Creating diverse synapses from the same moleculesCurrent Opinion in Neurobiology 51:8–15.https://doi.org/10.1016/j.conb.2018.01.001
-
Past and future of analog-digital modulation of synaptic transmissionFrontiers in Cellular Neuroscience 13:160.https://doi.org/10.3389/fncel.2019.00160
Article and author information
Author details
Publication history
Copyright
© 2023, Kaas et al.
This article is distributed under the terms of the Creative Commons Attribution License, which permits unrestricted use and redistribution provided that the original author and source are credited.
Metrics
-
- 705
- views
-
- 75
- downloads
-
- 0
- citations
Views, downloads and citations are aggregated across all versions of this paper published by eLife.
Download links
Downloads (link to download the article as PDF)
Open citations (links to open the citations from this article in various online reference manager services)
Cite this article (links to download the citations from this article in formats compatible with various reference manager tools)
Further reading
-
- Neuroscience
Gamma oscillations in brain activity (30–150 Hz) have been studied for over 80 years. Although in the past three decades significant progress has been made to try to understand their functional role, a definitive answer regarding their causal implication in perception, cognition, and behavior still lies ahead of us. Here, we first review the basic neural mechanisms that give rise to gamma oscillations and then focus on two main pillars of exploration. The first pillar examines the major theories regarding their functional role in information processing in the brain, also highlighting critical viewpoints. The second pillar reviews a novel research direction that proposes a therapeutic role for gamma oscillations, namely the gamma entrainment using sensory stimulation (GENUS). We extensively discuss both the positive findings and the issues regarding reproducibility of GENUS. Going beyond the functional and therapeutic role of gamma, we propose a third pillar of exploration, where gamma, generated endogenously by cortical circuits, is essential for maintenance of healthy circuit function. We propose that four classes of interneurons, namely those expressing parvalbumin (PV), vasointestinal peptide (VIP), somatostatin (SST), and nitric oxide synthase (NOS) take advantage of endogenous gamma to perform active vasomotor control that maintains homeostasis in the neuronal tissue. According to this hypothesis, which we call GAMER (GAmma MEdiated ciRcuit maintenance), gamma oscillations act as a ‘servicing’ rhythm that enables efficient translation of neural activity into vascular responses that are essential for optimal neurometabolic processes. GAMER is an extension of GENUS, where endogenous rather than entrained gamma plays a fundamental role. Finally, we propose several critical experiments to test the GAMER hypothesis.
-
- Medicine
- Neuroscience
The advent of midazolam holds profound implications for modern clinical practice. The hypnotic and sedative effects of midazolam afford it broad clinical applicability. However, the specific mechanisms underlying the modulation of altered consciousness by midazolam remain elusive. Herein, using pharmacology, optogenetics, chemogenetics, fiber photometry, and gene knockdown, this in vivo research revealed the role of locus coeruleus (LC)-ventrolateral preoptic nucleus noradrenergic neural circuit in regulating midazolam-induced altered consciousness. This effect was mediated by α1 adrenergic receptors. Moreover, gamma-aminobutyric acid receptor type A (GABAA-R) represents a mechanistically crucial binding site in the LC for midazolam. These findings will provide novel insights into the neural circuit mechanisms underlying the recovery of consciousness after midazolam administration and will help guide the timing of clinical dosing and propose effective intervention targets for timely recovery from midazolam-induced loss of consciousness.