Modeling corticotroph deficiency with pituitary organoids supports the functional role of NFKB2 in human pituitary differentiation
eLife Assessment
This important study examines the effects of NFKB2 mutations on pituitary gland development through hypothalamic-pituitary organoids. The evidence supporting the main conclusions is solid, although analysis of additional clones to exclude inter-clone variability would strengthen the conclusions. This is a revised study, but insight into the mechanism of action of NFKB2 during pituitary development is incomplete. This work will be of interest to endocrinologists and biologists working on pituitary gland development and disease.
https://doi.org/10.7554/eLife.90875.3.sa0Important: Findings that have theoretical or practical implications beyond a single subfield
- Landmark
- Fundamental
- Important
- Valuable
- Useful
Solid: Methods, data and analyses broadly support the claims with only minor weaknesses
Incomplete: Main claims are only partially supported
- Exceptional
- Compelling
- Convincing
- Solid
- Incomplete
- Inadequate
During the peer-review process the editor and reviewers write an eLife Assessment that summarises the significance of the findings reported in the article (on a scale ranging from landmark to useful) and the strength of the evidence (on a scale ranging from exceptional to inadequate). Learn more about eLife Assessments
Abstract
Deficient Anterior pituitary with common Variable Immune Deficiency (DAVID) syndrome results from NFKB2 heterozygous mutations, causing adrenocorticotropic hormone deficiency (ACTHD) and primary hypogammaglobulinemia. While NFKB signaling plays a crucial role in the immune system, its connection to endocrine symptoms is unclear. We established a human disease model to investigate the role of NFKB2 in pituitary development by creating pituitary organoids from CRISPR/Cas9-edited human induced pluripotent stem cells (hiPSCs). Introducing homozygous TBX19K146R/K146R missense pathogenic variant in hiPSC, an allele found in congenital isolated ACTHD, led to a strong reduction of corticotrophs number in pituitary organoids. Then, we characterized the development of organoids harboring NFKB2D865G/D865G mutations found in DAVID patients. NFKB2D865G/D865G mutation acted at different levels of development with mutant organoids displaying changes in the expression of genes involved on pituitary progenitor generation (HESX1, PITX1, LHX3), hypothalamic secreted factors (BMP4, FGF8, FGF10), epithelial-to-mesenchymal transition, lineage precursors development (TBX19, POU1F1) and corticotrophs terminal differentiation (PCSK1, POMC), and showed drastic reduction in the number of corticotrophs. Our results provide strong evidence for the direct role of NFKB2 mutations in the endocrine phenotype observed in patients leading to a new classification of a NFKB2 variant of previously unknown clinical significance as pathogenic in pituitary development.
Introduction
ACTHD is defined by an insufficient production of ACTH by the pituitary, and then low adrenal cortisol production. Proper diagnosis and management of ACTHD is crucial as it is a life-threatening condition in the neonatal period characterized by hypoglycemia, cholestatic jaundice, and seizures (Couture et al., 2012). Constitutional ACTHD, i.e., ACTHD diagnosed at birth or during the first years of life, can be isolated or associated with other pituitary hormones deficiencies, such as growth hormone (GH) or thyrotropin-stimulating hormone (TSH), then as part of combined pituitary hormone deficiency (CPHD). ACTHD can be due to mutations in genes coding for transcription factors responsible for pituitary ontogenesis, especially the T-box transcription factor TBX19 (also known as TPIT), NFKB2, LHX3, LHX4, PROP1, HESX1, SOX2, SOX3, OTX2, and FGF8.
Mutations of TBX19 account for approximately two-thirds of neonatal-onset complete isolated ACTHD (Couture et al., 2012). TBX19 is a T-box transcription factor restricted to pituitary pro-opiomelanocortin (POMC)-expressing cells in mice and humans. It is essential for POMC gene transcription and terminal differentiation of POMC-expressing cells (Lamolet et al., 2001). POMC is the precursor of ACTH. Tbx19-deficient mice have only a few pituitary Pomc-expressing cells, with very low ACTH and undetectable corticosterone levels (Pulichino et al., 2003). In humans with isolated ACTH deficiency, TBX19 mutations lead to loss-of-function by different mechanisms, such as the K146R (exon 2, c.437 A>G) pathogenic variant located in the T-box region, which results in a loss of DNA-binding ability (Couture et al., 2012).
Thanks to GENHYPOPIT (Reynaud et al., 2006; Jullien et al., 2019; Jullien et al., 2021), an international network aimed at identifying new genetic etiologies of combined pituitary hormone deficiency, we described the first cases of DAVID syndrome, a rare association of hypopituitarism (mainly ACTHD) and immune deficiency (hypogammaglobulinemia) (Quentien et al., 2012). DAVID syndrome is associated with variants in the nuclear factor kappa-B subunit 2 (NFKB2) gene (Chen et al., 2013; Brue et al., 2014). In particular, we reported that a pathogenic, heterozygous D865G (exon 23, c.2594 A>G) NFKB2 variant was found in a patient presenting with severe recurrent infections from 2 y of age, who at the age of 5 was diagnosed with ACTHD (Quentien et al., 2012). Mutations in the C-terminal region of NFKB2 lead to the disruption of both non-canonical and canonical pathways (Wirasinha et al., 2021; Kuehn et al., 2017; Lee et al., 2014). Full-length NFKB2 (p100) protein has two critical serines, S866 and S870, in the C-terminal domain. Phosphorylation of these sites yields the transcriptionally active NFKB2 (p52) through proteasomal processing of p100 protein. The D865G mutation, located adjacent to the critical S866 phosphorylation site, protects mutant protein from proteasomal degradation, causes defective processing of p100 to p52, and results in reduced translocation of p52 to the nucleus (Chen et al., 2013; Kuehn et al., 2017; Lee et al., 2014). NFKB2+/D865G resulted in 50% of normal processing to p52, whereas NFKB2D865G/D865G exhibited near-absence of p52 (Lee et al., 2014). The nuclear factor kappa B (NFKB) signaling pathway is a known key regulator of the immune system (Lee et al., 2014; Lindsley et al., 2014; Carragher et al., 2004), which likely explains the immune phenotype of patients with DAVID syndrome, including susceptibility to infections and auto-immune disorders (Mac et al., 2023). In contrast, the underlying mechanism causing pituitary disorders remains unknown, with two predominating hypotheses: an indirect autoimmune hypophysitis, preferentially affecting corticotroph function, or a primary developmental defect suggested by the expression of NFKB2 in the developing human pituitary (Zhang et al., 2020). However, a developmental role for NFKB2 was not confirmed in the mouse: the Lym1 mouse model carrying a homozygous nonsense variant Y868* presented an apparently normal pituitary development and function (Brue et al., 2014).
Over the last years, human induced pluripotent stem cell (hiPSC)-derived organoids have emerged as promising models to study many developmental mechanisms and their perturbation in disease (Ho et al., 2018). Pioneering work on human embryonic stem cells and later, hiPSC, has established that 3D organoid models can replicate aspects of pituitary development (Ozone et al., 2016; Kasai et al., 2020; Matsumoto et al., 2019), and could thus be of major interest in modeling pituitary disorders (Ozone et al., 2016; Suga et al., 2011; Sasai et al., 2012). In the present study, we applied recent progress in genome editing using CRISPR/Cas9 (Sun and Ding, 2017) to a refined protocol to derive 3D organoids from hiPSC in order to model ACTHD. We validated the recapitulation of corticotroph cell differentiation in vitro by introducing a TBX19 mutation known to induce isolated human ACTHD and documenting the subsequent deficiencies in corticotroph development. When our model was used to characterize the D865G NFKB2 variant found in patients with DAVID syndrome, it displayed dramatically altered corticotroph differentiation in the absence of immune cells, clearly demonstrating for the first time a direct role of NFKB2 in human pituitary development.
Results
Corticotroph deficiency can be modeled by directed differentiation of TBX19-mutant hiPSC in 3D culture
To first validate the pituitary organoid model and determine whether the TBX19 mutant can affect corticotrophs differentiation, we generated a TBX19 KI hiPSC line from the control line using CRISPR/Cas9 (Figure 1A–D; Figure 1—figure supplement 1). One KI clone carrying TBX19K146R/K146R was obtained after screening 100 clones by CAPS and confirmation by Sanger sequencing (Figure 1—figure supplement 2). This mutant clone was then amplified and differentiated into pituitary organoids, in parallel with the control line.
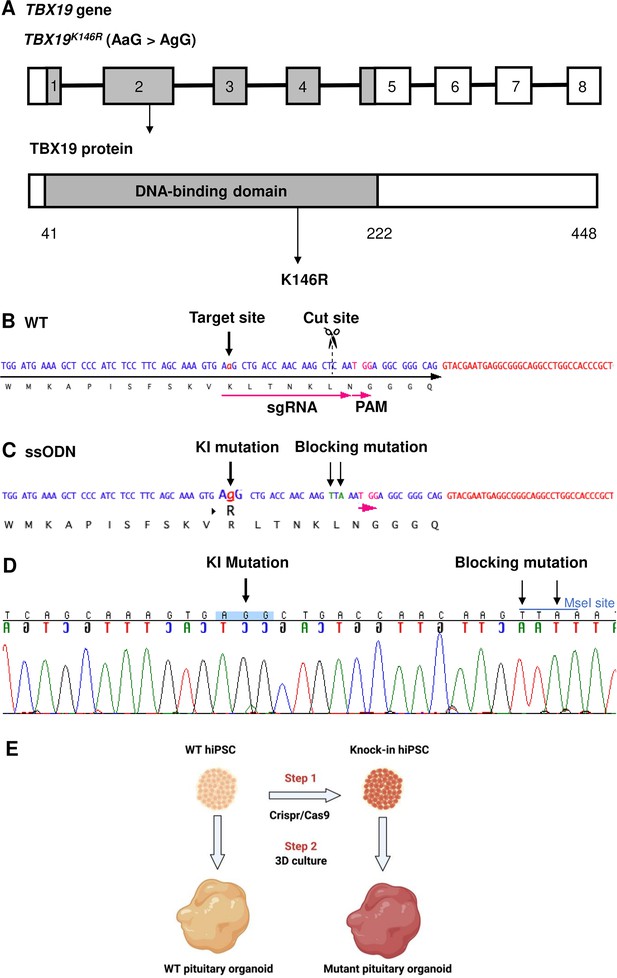
Design of single guide RNA (sgRNA) and single-stranded oligo DNA nucleotides (ssODN) to edit in the TBX19K146R mutation.
(A) Illustration of the TBX19 gene (HGNC: 11596; ENSEMBL: ENSG00000143178; Human GRCh38) and TBX19 protein. (B) Wild-type (WT) sequence containing target site, cut site, target, and protospacer-adjacent motif (PAM) sequences. (C) ssODN design to edit in a missense K146R mutant of TBX19 using CRISPR/Cas9. (D) Sequence analysis of a TBX19 KI human induced pluripotent stem cell (hiPSC) clone 63 obtained by Sanger sequencing after screening by cleaved amplified polymorphic sequences (CAPS). This clone was subsequently used in this work to differentiate into pituitary organoids (see below). (E) Summary of our strategy procedure. Step 1: Production of the knock-in hiPSC lines by CRISPR/Cas9 genome editing. Step 2: Differentiation into pituitary organoids from mutant hiPSC lines in parallel with the isogenic WT line using 3D culture, followed by the comparison of the development of organoids between the two groups.
The ability of the TBX19 KI line to differentiate into the hypothalamic-pituitary structure was compared to the control line (200 organoids for each line) using a 3D organoid culture method, in which pituitary-like and hypothalamus-like structures simultaneously develop (Figures 1E and 2A, Figure 2—figure supplement 1A–B). In this method, hiPSC differentiates into hypothalamic progenitors in the central part of the organoids, whereas the outer layer differentiates into oral ectoderm, that will in turn develop into anterior pituitary tissue (Figure 2A, Figure 2—figure supplement 1C). The expression of several key markers of pituitary development and differentiation was then compared in mutant TBX19 KI organoids over time, matched to control WT organoids using qRT-PCR (d0, d6, d18, d27, d48, d75, d105) and immunofluorescence (d48 and d105) (Figure 2A). Organoids grew in the culture medium with average sizes in their greatest dimension from 0.4 mm on day 6–1.9 mm on day 105 (Figure 2B).
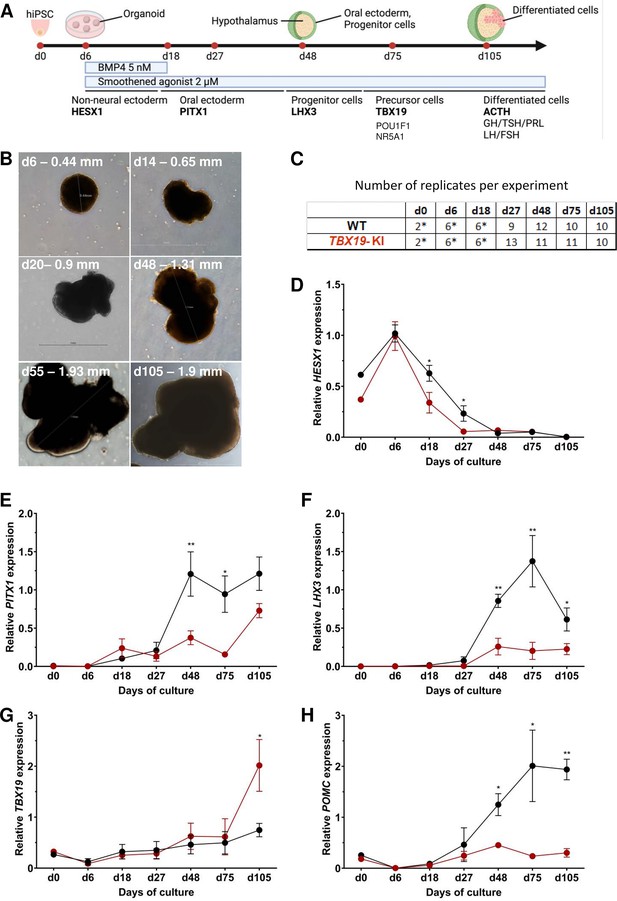
Time course of organoid growth and gene expression in wild-type (WT) and TBX19 KI organoids.
(A) Culture protocol and outline to generate pituitary organoids in three-dimensional (3D) culture from human induced pluripotent stem cell (hiPSC). Organoids were collected at days (d) 0, 6, 18, 27, 48, 75, and 105 during differentiation to analyze. (B) Brightfield microscopy views of WT organoid examples at different time points throughout differentiation. Scale bars are indicated in each image. (C) Number of replicates per time point and per genotype in experiments depicted in the following graphs. Asterisks indicate that 7–8 organoids were grouped for each sample. For other points, each sample consists of a single organoid. (D–H) Relative quantification (RQ) mRNA expression analysis for key markers of pituitary organoids during differentiation: WT (in black line) and TBX19 KI organoids (in red line). Relative quantification of each target gene was obtained by the 2-ΔΔCt method from qRT-PCR results (see Methods). Data show means ± standard error of the mean (SEM, Mann-Whitney t-test [unpaired, two-tailed, nonparametric]). p<0.05 (*), p<0.01 (**). (D) Relative quantification of HESX1 expression, the earliest pituitary placode marker assessed. The expression of HESX1 is significantly downregulated in TBX19 KI organoids vs. WT at d18 and d27. (E) Relative quantification expression of PITX1, a pituitary progenitor marker. PITX1 was significantly downregulated in TBX19 KI organoids by d48 and d75. (F) Relative quantification expression of LHX3, a pituitary progenitor marker. LHX3 was significantly lower in TBX19 KI organoids as compared to WT from d48 onwards. (G) Relative quantification expression of TBX19, a critical transcriptional determinant for corticotroph differentiation. TBX19 expression is higher in TBX19 KI organoids at d105. (H) Relative quantification expression of pro-opiomelanocortin (POMC), a corticotroph marker. POMC was significantly downregulated in TBX19 KI organoids from d48 onwards.
Successful differentiation was validated in WT organoids by qRT-PCR, at early stages by the expression of a set of pituitary transcription factors, including HESX1, PITX1, and LHX3, and at the latest stage of corticotroph cell differentiation by the expression of TBX19 and POMC (Figure 2A and C–H). HESX1 is the first specific marker of pituitary primordium and its expression is important for the early determination of the gland. In WT organoids, HESX1 peaked around day 6 of culture, and was rapidly downregulated from day 18 (Figure 2D). Expression of PITX1 (oral ectoderm marker) and LHX3 (pituitary progenitor marker) increased from day 27, then PITX1 reached a plateau from d48 (Figure 2E) whereas LHX3 peaked at d75 and was then slightly downregulated (Figure 2F). Immunofluorescence confirmed the presence of oral ectoderm cells expressing PITX1 and CDH1 (E-cadherin, Figure 2—figure supplement 1C), therefore, resembling Rathke’s pouch progenitors. The effective generation of pituitary progenitors was confirmed by the presence of LHX3 + cells in WT organoids on day 48 (Figure 3A, Figure 2—figure supplement 1A). These cells were located in the outermost layer of WT organoids, surrounding the hypothalamic progenitor layer that expressed NKX2.1 (Figure 2—figure supplement 1B). By day 105, WT organoids contained many differentiated corticotrophs co-expressing TBX19 protein in the nucleus and ACTH in the cytoplasm, as seen in confocal microscopy, a critical feature for the rest of our investigations (n=8, Figure 3B, Figure 2—figure supplement 1D). Consistent with these images, qRT-PCR results confirmed the highest levels of TBX19 and POMC expression after at least 75 d of culture in WT organoids (Figure 2G–H). Taken together, these data showed that pituitary organoids in 3D culture mimic human pituitary ontogenesis, and were characterized in our conditions by the ability to differentiate into pituitary progenitors by day 48 and into corticotroph cells by day 105.
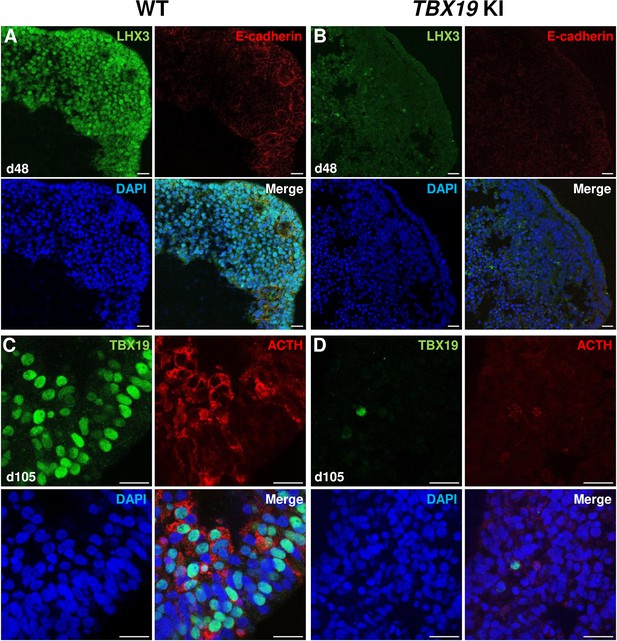
Impairment of corticotroph development in TBX19 KI organoids as compared with controls at the protein level.
(A, B) Immunostaining of LHX3 and CDH1 (E-cadherin) expression in epithelial cells, typical of Rathke’s pouch ectoderm in early pituitary primordia, was reduced in TBX19 KI organoid vs wild-type (WT) on day 48 (n=10 organoids for each group). Scale bars: 10 μm. (C, D) Immunostaining showed that adrenocorticotropic hormone (ACTH) and TBX19 expressions were reduced in TBX19 KI organoid vs WT on day 105 (n=10 organoids for each group). Scale bars: 10 μm.
We then tested whether ACTHD could be modeled by TBX19-mutant pituitary organoids. To this end, the development of pituitary organoids carrying TBX19 KI was matched to WT organoid development and analyzed using qRT-PCR and immunofluorescence for pituitary ontogenesis markers as described above. Our data showed a significant decrease in the expression of HESX1 at d18 and d27 in the mutant (Figure 2D). Both PITX1 and LHX3 transcript levels were significantly decreased by day 48 and day 75 in mutant organoids (Figure 2E and F), with only partial recovery for PITX1 by d105, suggesting an impairment of pituitary progenitor generation. Although TBX19 transcript levels were unchanged until d75 (Figure 2G), we observed a decrease in POMC expression in the TBX19 KI organoids that was significant from day 48 onwards (Figure 2H). TBX19 expression was significantly higher in mutant organoids at d105, but this had no influence on POMC expression, which remained very low. These results confirmed that our organoid model can recapitulate the need for a fully functional TBX19 protein to achieve proper POMC gene transcription during human pituitary development.
Next, we checked several pituitary markers by immunofluorescence (Figure 3A–D). In line with qRT-PCR results, TBX19 KI organoid immunostaining on day 48 showed that LHX3 protein expression was decreased in TBX19 KI organoids (n=10 organoids for each group, Figure 3A and B). By day 105, we observed that there were significantly fewer corticotroph cells expressing ACTH and TBX19 proteins in TBX19K146R/K146R organoids (n=10 organoids for each group, Figure 3C and D).
Finally, in order to take into account the possibility of regionalized sampling in the section, we performed a quantitative analysis of ACTH by transparizing organoids immunostained for the hormone and imaging them by light-sheet confocal microscopy. 3D reconstruction confirmed that ACTH was nearly absent from TBX19 KI organoids on day 105 compared to control organoids (Figure 4A–B and Figure 4—video 1 and Figure 4—video 2). We observed that corticotroph cell distribution was not uniform throughout the organoids, but was rather concentrated in one or a few buds. In summary, there was a significant decrease in the number of ACTH + corticotroph cells with a TBX19 KI genotype on day 105 versus control organoids (Figure 4C).
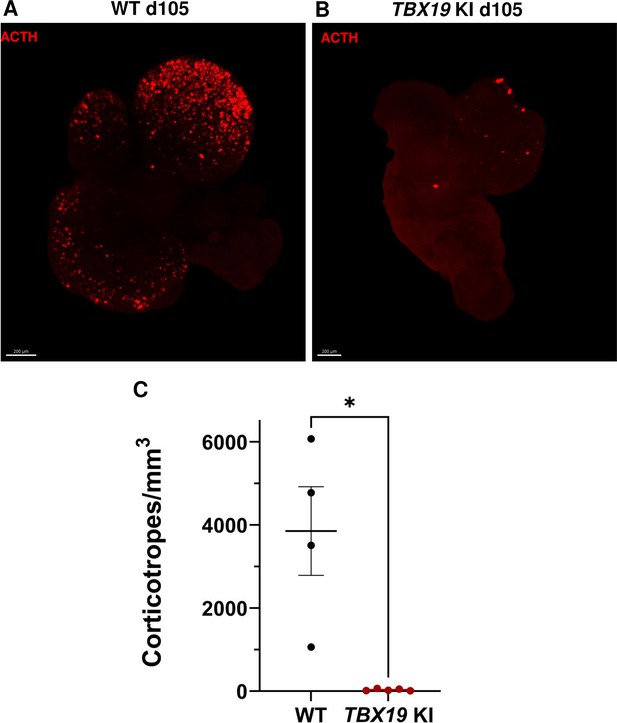
3D reconstruction of whole wild-type (WT) and TBX19 KI organoids on day 105.
(A) A representative image of whole-mount immunostaining against adrenocorticotropic hormone (ACTH) in a cleared WT organoid on d105 using light-sheet microscopy. Scale bar: 200 μm. (B) A representative image of whole-mount immunostaining against ACTH in a cleared TBX19 KI organoid on d105 as above, showing impaired corticotroph differentiation. Scale bar: 200 μm. (C) The number of corticotroph cells per mm3 was significantly decreased in TBX19 KI organoids (* p=0.0159). Means ± SEM for n=4 WT, n=5 TBX19 KI organoids. Mann-Whitney test (unpaired, two-tailed, nonparametric).
Together, this first set of experiments demonstrated that genome editing of hiPSC with a KI homozygous pathogenic variant of TBX19 effectively prevented their differentiation into ACTH-producing corticotrophs.
NFKB2 signaling is vital for corticotroph development
As our organoid model for the study of ACTHD was validated with the TBX19 mutant line, we then used the same approach to investigate the potential role of NFKB2 in human hypothalamic-pituitary development. To do so, we first established a NFKB2 KI mutant hiPSC line using CRISPR/Cas9 (Figure 5) from an isogenic control. The NFKB2D865G homozygous mutation was chosen because it has been shown to severely affect NFKB2 p52 processing (Lee et al., 2014). Two homozygous KI clones carrying NFKB2D865G/D865G were obtained after screening by CAPS and confirmation by Sanger sequencing (Figure 5—figure supplement 1). We selected one homozygous missense mutation NFKB2 KI clone (#7) to amplify and generate 200 mutant organoids in parallel with 200 WT organoids in 3D culture.
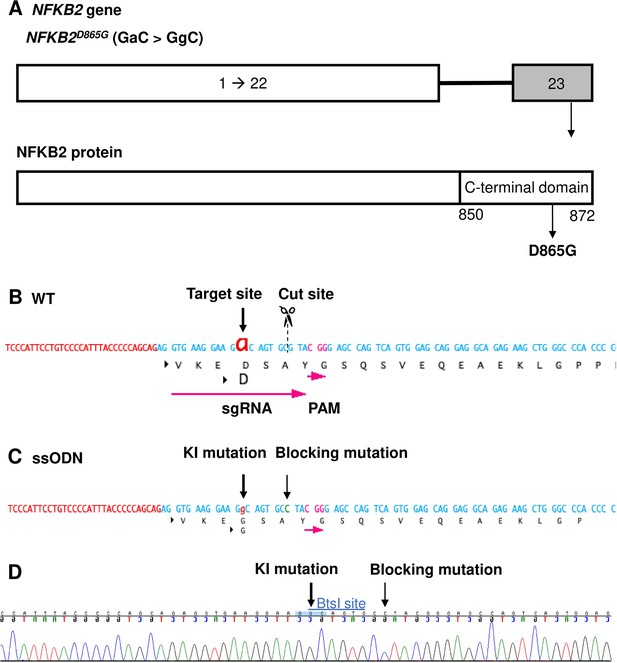
Design of sgRNA and single-stranded oligo DNA nucleotides (ssODN) to introduce a nuclear factor kappa-B subunit 2 (NFKB2)D865G mutation.
(A) Illustration of the NFKB2 gene (HGNC: 7795; ENSEMBL: ENSG00000077150; Human GRCh38). (B) Wild-type (WT) sequence containing target site, cut site, target, and protospacer-adjacent motif (PAM) sequence. (C) ssODN design to introduce the missense mutation D865G into NFKB2 using CRISPR/Cas9. (D) Sequence analysis of the NFKB2 KI hiPSC clone 7, obtained by Sanger sequencing, after screening by cleaved amplified polymorphic sequences (CAPS). This clone was subsequently used in this work to differentiate into pituitary organoids (see below).
Next, we compared the development and differentiation of the NFKB2 KI organoids versus WT organoids during the culture by qRT-PCR and immunofluorescence as described above. The results of qRT-PCR showed no significant difference in the peak expression level of HESX1. However, higher HESX1 expression in mutant organoids from d27 to d75 suggested impaired downregulation of the gene (Figure 6B). Concomitantly, a significantly decreased expression of PITX1 and LHX3 in NFKB2 KI organoids was found from d48 onwards, suggesting reduced differentiation of pituitary progenitors (Figure 6C–D). Similar to what we observed in TBX19 KI mutants, TBX19 expression was increased in NFKB2 KI organoids at d105 (Figure 6E). However, this had no impact on POMC expression levels, which remained significantly lower in NFKB2 KI organoids (Figure 6F), suggesting a role for NFKB2 in corticotroph terminal differentiation.
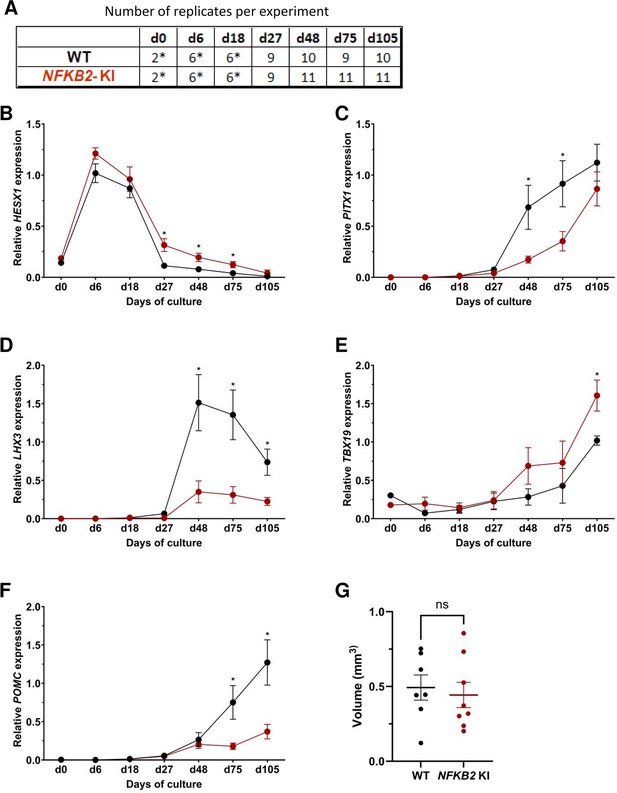
Time course of organoid growth and gene expression in wild-type (WT) and nuclear factor kappa-B subunit 2 (NFKB2) KI organoids.
(A) Number of replicates per time point and per genotype in experiments depicted in the following graphs. Asterisks indicate that 7–8 organoids were grouped for each sample. For other point, each sample consists of a single organoid. (B–F) Relative quantification (RQ) mRNA expression analysis for key markers of pituitary organoids during differentiation: WT (black line and dots) and NFKB2 KI organoids (red line and dots). Data show means ± standard error of the mean (SEM);. Mann-Whitney t-test (unpaired, two-tailed, nonparametric). p<0.05 (*), p<0.01 (**). (B) Relative quantification expression of HESX1, the earliest pituitary placode marker assessed. The expression of HESX1 was upregulated in NFKB2 KI organoids vs. WT between d27 and d75. (C) Pituitary progenitor marker PITX1 was significantly downregulated in NFKB2 KI organoids at d48 and d75. (D) Pituitary progenitor marker LHX3 was significantly lower in NFKB2 KI organoids as compared to WT from d48 onwards. (E) Relative quantification expression of TBX19, a corticotroph marker. TBX19 was significantly increased in NFKB2 KI organoids by d105. (F) Relative quantification expression of pro-opiomelanocortin (POMC), a corticotroph marker. POMC was significantly downregulated in NFKB2 KI organoids from d75. (G) Volume of organoids (mm3) on d105, calculated using Imaris software (see in methods). There was no significant difference in volume between WT and NFKB2 KI organoids (p=0.6126). Data show means ± SEM; n=7 in the WT group, n=8 in the mutant group. Mann-Whitney test (unpaired, two-tailed).
As MRI had revealed pituitary hypoplasia in 43% of patients with DAVID syndrome (Mac et al., 2023), we investigated whether mutant organoids might be smaller than WT organoids. Comparing the volume of both types of organoids on day 105, we found no significant difference (p=0.6, WT n=7, mutant n=8, Figure 6G). In line with qRT-PCR results, immunostaining showed less expression of LHX3 in NFKB2 KI organoids on day 48 compared to the WT (Figure 7A–B). NFKB2 (the antibody did not discriminate between p100/p52 isoforms) was robustly expressed in WT LHX3 + pituitary progenitors on day 48 (Figure 7A). Unexpectedly, NFKB2 p100/p52 immunostaining was weaker in NFKB2 KI organoids than in WT organoids (Figure 7A–B). Immunostaining at d105 in NFKB2 KI organoids showed the presence of many TBX19 + nuclei, in line with the qRT-PCR results, but few of them were surrounded by cytoplasmic ACTH signal (Figure 7C–D). Quantification after 3D reconstruction of transparent whole organoids showed significantly fewer ACTH + cells in NFKB2 KI organoids on day 105 compared to WT organoids in both qualitative (Figure 8A–B and Figure 8—video 1 and Figure 8—video 2) and quantitative (Figure 8C) assessments. In the absence of an immune system, this is strong evidence in support of a direct role for NFKB2 signaling in pituitary differentiation.
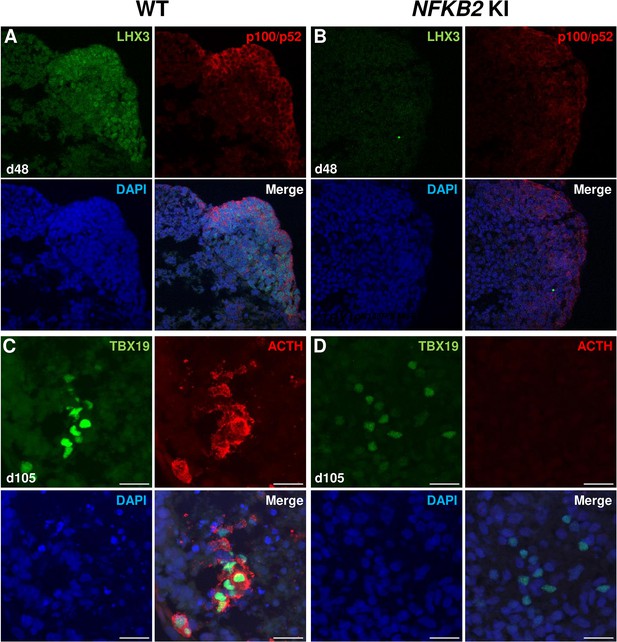
Impairment of pituitary development in nuclear factor kappa-B subunit 2 (NFKB2) KI organoids.
(A, B) Immunostaining for LHX3 (green) and p100/p52 (red) in the early pituitary-type epithelium was reduced in NFKB2 KI organoids vs wild-type (WT) on day 48 (n=10 organoids for each group). Stronger expression of p100/p52 was observed in pituitary progenitors, but expression in the hypothalamic part of the organoid cannot be excluded. Scale bar: 10 μm (C, D) by day 105, although nuclear TBX19 was detectable in both mutants, these cells failed to co-express ACTH in NFKB2 KI organoids (n=10 organoids for each group). Scale bars: 10 μm.
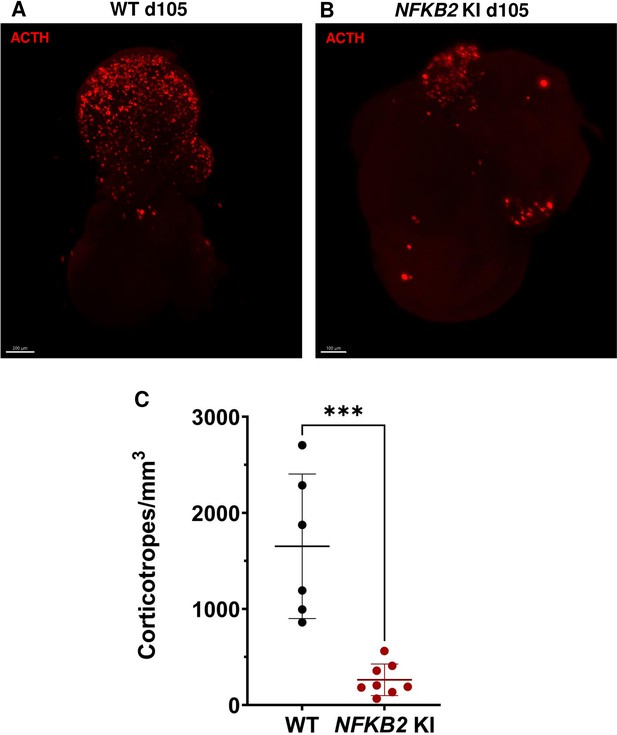
3D reconstruction of whole wild-type (WT) and nuclear factor kappa-B subunit 2 (NFKB2) KI organoids on day 105.
(A) A representative image of whole-mount immunostaining against adrenocorticotropic hormone (ACTH) in a cleared WT organoid on d105 using light-sheet microscopy. Scale bar: 200 μm. (B) A representative image of whole-mount immunostaining against ACTH in a cleared NFKB2 KI organoid on d105 as above, showing impaired corticotroph differentiation. Scale bar: 100 μm. (C) The number of corticotroph cells per mm3 was significantly decreased in NFKB2 KI organoids (*p=0.0007). Means ± SEM for n=6 organoids WT, n=8 NFKB2 KI organoids. Mann-Whitney test (unpaired, two-tailed, nonparametric).
To further investigate other downstream pathways altered in NFKB2 KI pituitary organoids, we performed whole RNA-seq of five distinct organoids on day 48 from the NFKB2 KI line versus five organoids derived from its corresponding hiPSC control line. As we found that only 60–70% of organoids show signs of pituitary cell differentiation, we chose to perform a preselection of organoids, based on RT-qPCR expression of selected markers (SOX2, HESX1, PITX1, LHX3, TBX19, POU1F1, and POMC) in order to avoid having ‘empty’ HPOs sent for bulk RNA-seq. Differential expression (DE) gene analysis identified 2559 significantly upregulated and 2260 significantly downregulated genes at adjusted p-value (pAdj) <0.05. The global results are depicted as a heatmap to show the similarities across organoids of similar genotypes (Figure 9A), and a volcano plot highlights the magnitudes of DE between the two groups (Figure 9B, Figure 9—source data 1).
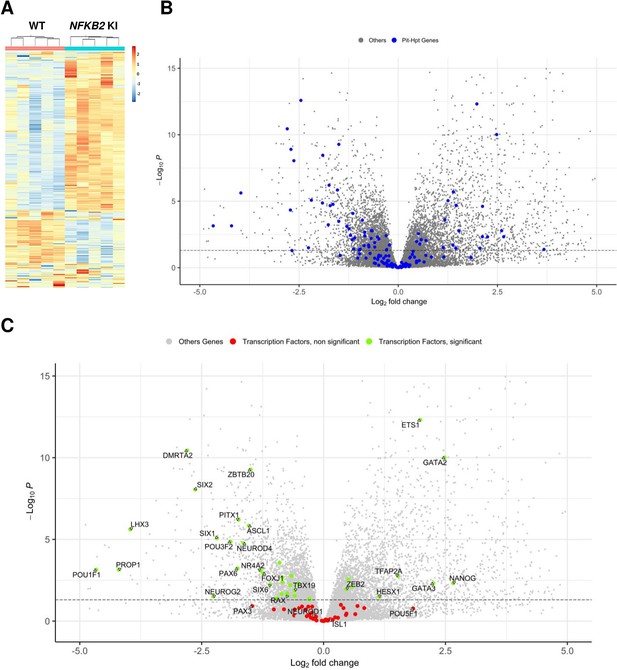
Whole-transcriptome profiles of wild-type (WT) vs nuclear factor kappa-B subunit 2 (NFKB2) knock-in (KI) organoids on day 48.
(A) Heat map showing global differential gene expression between WT vs NFKB2 KI organoids (n=5 for each group). (B) Volcano plot of genes showing differential gene expression between WT vs NFKB2 KI organoids (expressed in Log2 fold-change). Blue dots in the left panel indicate 144 genes involved in hypothalamic-pituitary development. Gray dots indicate all other genes detected in RNA-seq. (C) Volcano plot of genes showing differential gene expression between WT vs NFKB2 KI organoids. Green dots indicate genes coding for 39 key transcription factors whose expression is significantly changed (padj <0.05). Red dots indicate key transcription factors with non-significant changes (padj >0.05). Gray dots indicate all other genes detected in RNA-seq.
-
Figure 9—source data 1
Nuclear factor kappa-B subunit 2 (NFKB2) nock-in (KI) vs wild-type (WT) gene expression at d48 Excel spreadsheet showing NFKB2 KI vs WT expression ratios measured by RNA-seq (n=5 organoids of each genotype).
- https://cdn.elifesciences.org/articles/90875/elife-90875-fig9-data1-v1.xlsx
NFKB signaling did not seem to be affected in NFKB2 KI organoids, as most genes involved in that pathway had fold-change values between –0.5 and 0.5 when significant (Figure 9—figure supplement 1). Among a list of 144 genes known to have a functional influence on pituitary-hypothalamic development as curated from the published literature, 67 were found to be differentially expressed in NFKB2 KI organoids (pAdj <0.05) (Figure 9B, and Table 1), of which 39 encode transcription factors (Figure 9C). Expression of these transcription factors was mostly downregulated, with a marked decrease in expression of pituitary progenitor markers such as PITX1 and LHX3. In contrast, the earliest Rathke’s pouch marker, HESX1, showed a twofold increased expression in NFKB2 KI organoids, suggesting impaired progression of pituitary progenitors towards more differentiated stages.
RNA-seq expression data for a list of 144 genes known from the literature to have a functional influence on pituitary-hypothalamic development.
Differentially expressed genes (padj <0.05) in nuclear factor kappa-B subunit 2 (NFKB2) KI organoids are highlighted in green when upregulated and in orange when downregulated. FC; Fold change.
Gene name | FC | padj | Gene name | FC | padj | Gene name | FC | padj |
---|---|---|---|---|---|---|---|---|
ADCYAP1 | 0,447 | 0,00789 | GLI3 | 1,279 | 0,10094 | PITX2 | 1,072 | 0,86688 |
AIP | 0,898 | 0,53442 | GNAS | 1,058 | 0,39519 | POLR3A | 1,014 | 0,90718 |
ALDH1A1 | 0,153 | 1,3E-09 | GNRHR | 0,453 | 8,3E-05 | POMC | 0,434 | 0,0036 |
ALDH1A2 | 0,152 | 4,6E-05 | GNRHR2 | 0,698 | 0,23938 | POU1F1 | 0,039 | 0,00072 |
ALDH1A3 | 0,182 | 2,6E-13 | GPR101 | 1,209 | 0,83045 | POU3F1 | 1,374 | 0,42093 |
AR | 0,634 | 0,00168 | GREB1 | 1,007 | 0,97784 | POU3F2 | 0,266 | 1,4E-05 |
ARNT2 | 0,534 | 0,00027 | GSX1 | 0,659 | 0,45012 | POU5F1 | 3,573 | 0,17018 |
ASCL1 | 0,345 | 1,4E-06 | HES1 | 0,663 | 0,02805 | PROKR2 | 1,056 | 0,97365 |
AXIN2 | 1,296 | 0,05889 | HESX1 | 2,193 | 0,03121 | PROP1 | 0,054 | 0,00071 |
BMP2 | 0,750 | 0,34278 | HEY1 | 0,804 | 0,13046 | RAX | 0,599 | 0,02697 |
BMP4 | 4,357 | 2,4E-05 | HMGA2 | 0,775 | 0,19471 | RBPJ | 0,814 | 0,04665 |
BMPR1A | 1,019 | 0,91991 | INHBB | 0,458 | 0,03974 | S100B | 0,304 | 2,2E-05 |
BRAF | 0,892 | 0,48183 | ISL1 | 1,097 | 0,75812 | SALL1 | 1,106 | 0,76122 |
CDH1 | 1,626 | 0,009 | KRT8 | 2,617 | 2E-06 | SHH | 0,786 | 0,51179 |
CGA | 12,756 | 0,04234 | LATS1 | 0,857 | 0,01997 | SIX1 | 0,219 | 8,4E-06 |
CREB1 | 0,850 | 0,12901 | LATS2 | 1,460 | 0,01663 | SIX2 | 0,161 | 9E-09 |
CRH | 4,347 | 0,00469 | LHX2 | 0,622 | 0,00625 | SIX3 | 0,859 | 0,69976 |
CRHR1 | 0,156 | 0,05131 | LHX3 | 0,064 | 2,4E-06 | SIX6 | 0,467 | 0,00586 |
CRHR2 | 0,823 | 0,73602 | LHX4 | 0,557 | 0,00398 | SLC15A2 | 0,624 | 0,00164 |
CTNNB1 | 1,009 | 0,95542 | MEN1 | 1,030 | 0,80309 | SLC6A3 | 2,215 | 0,15797 |
CYP11A1 | 6,086 | 0,00166 | MSX1 | 0,904 | 0,90909 | SMO | 0,957 | 0,70401 |
DIO2 | 1,114 | 0,71885 | NANOG | 6,301 | 0,00472 | SMOC2 | 4,163 | 0,03488 |
DISP1 | 1,142 | 0,47708 | NEUROD1 | 0,883 | 0,50966 | SOX11 | 0,736 | 0,12757 |
DLK1 | 0,594 | 0,01988 | NEUROD2 | 1,137 | 0,84178 | SOX2 | 0,815 | 0,42151 |
DMRTA2 | 0,144 | 3,6E-11 | NEUROD4 | 0,320 | 1,7E-05 | SOX3 | 0,787 | 0,50152 |
DRD2 | 2,753 | 2E-05 | NEUROG1 | 0,493 | 0,19209 | SOX9 | 0,557 | 0,00214 |
DUOX2 | 2,595 | 0,01961 | NEUROG2 | 0,208 | 0,03165 | SRD5A1 | 0,961 | 0,79794 |
DZIP1 | 0,818 | 0,04264 | NFKB2 | 1,236 | 0,16144 | SST | 0,994 | 0,9921 |
EGR1 | 1,784 | 0,15768 | NKX2-1 | 0,707 | 0,15518 | SSTR1 | 0,509 | 0,09008 |
EPCAM | 1,128 | 0,72728 | NKX2-2 | 1,569 | 0,37322 | SSTR2 | 0,769 | 0,43599 |
ETS1 | 3,947 | 4,8E-13 | NOG | 1,376 | 0,31897 | TAZ | 0,967 | 0,85318 |
EYA1 | 0,514 | 0,03962 | NOTCH1 | 1,006 | 0,98289 | TBX19 | 0,663 | 0,01294 |
FGF10 | 0,267 | 3,5E-09 | NOTCH2 | 0,937 | 0,60691 | TBX3 | 0,997 | 0,98911 |
FGF2 | 1,224 | 0,18237 | NR0B1 | 1,062 | 0,92893 | TCF4 | 1,015 | 0,95769 |
FGF3 | 0,611 | 0,08445 | NR3C1 | 0,846 | 0,30279 | TCF7L2 | 1,178 | 0,58844 |
FGF8 | 0,506 | 0,04827 | NR4A1 | 1,227 | 0,62126 | TFAP2A | 2,887 | 0,00173 |
FOLR1 | 2,729 | 0,03443 | NR4A2 | 0,407 | 0,00078 | TGIF1 | 1,422 | 0,00278 |
FOXA1 | 0,550 | 0,02193 | NR5A1 | 0,834 | 0,61396 | THRA | 0,714 | 0,00712 |
FOXC1 | 1,619 | 0,11818 | NUPR1 | 1,384 | 0,3477 | THRB | 2,260 | 0,00025 |
FOXJ1 | 0,424 | 0,00117 | OLFM1 | 0,830 | 0,29166 | TIPRL | 0,810 | 0,00393 |
FOXO1 | 1,494 | 0,00782 | OTP | 0,663 | 0,19349 | TLE5 | 0,695 | 0,00502 |
FST | 2,384 | 9,1E-06 | OTX1 | 0,863 | 0,72226 | TSHR | 0,998 | 0,99753 |
GATA2 | 5,579 | 9,7E-11 | OTX2 | 0,976 | 0,94713 | USP39 | 1,035 | 0,7908 |
GATA3 | 4,758 | 0,00483 | PAX3 | 0,362 | 0,11963 | WNT4 | 0,712 | 0,11665 |
GHRH | 0,712 | 0,65053 | PAX6 | 0,294 | 0,00059 | WNT5A | 1,442 | 0,29543 |
GHRHR | 0,672 | 0,48512 | PAX7 | 0,570 | 0,1882 | YAP1 | 1,091 | 0,56585 |
GLI1 | 1,050 | 0,84104 | PCSK1 | 0,354 | 0,00033 | ZBTB20 | 0,354 | 5,3E-10 |
GLI2 | 1,328 | 0,1593 | PITX1 | 0,299 | 6,3E-07 | ZEB2 | 1,378 | 0,01078 |
During pituitary development, progenitors undergo epithelial-to-mesenchymal transition (EMT). Data from human fetuses (Zhang et al., 2020) suggest that pituitary stem cells/progenitors are in a hybrid state, as they simultaneously express ‘stemness’ as well as epithelial and mesenchymal-associated genes, and expression of all these gene sets decreases in concert during the course of differentiation. While the overall number of anterior pituitary-type cells in NFKB2 KI organoids was not affected, as confirmed by RT-qPCR of the pan-pituitary cell adhesion marker EPCAM, there was a significant decrease in the expression of stemness markers such as HES1, SOX2, and SOX9 (Table 2, left panel), accompanied by increased expression of stem cell-associated epithelial markers CDH1 and KRT8 (Table 2, middle panel) and decreased expression of stem cell-associated mesenchymal markers CDH2 and VIM. Other mesenchymal markers expressed in both stem cells and differentiating cells (COL1A1 and COL1A2) were upregulated (Table 2, right panel). CLDN6, which is expressed in early pituitary progenitors (Zhang et al., 2020), was upregulated (fold change = 2.3; p<0.0001) whereas CLDN9, widely expressed in the fetal human pituitary (Zhang et al., 2020), was decreased (fold change = 0.34; p<0.0001). Overall, these results suggest that progenitors initiate EMT, but stall during the process. Of note, the expression of ZEB2, SNAI1, and SNAI2, key initiators of EMT through the downregulation of CDH1, is significantly increased (fold changes of 1.37, 3.37, and 5.36, respectively; p<0.01), suggesting that NFKB2 plays an important role downstream from these effectors.
Differential expression analysis for genes associated with different stages of epithelial to mesenchymal transition in nuclear factor kappa-B subunit 2 (NFKB2)D865G/D865G vs wild-type (WT) organoids.
Significantly decreased and increased expressions are highlighted in orange and green, respectively. FC, Fold change.
Stemness markers | Epithelial markers | Mesenchymal markers | ||||||
---|---|---|---|---|---|---|---|---|
Gene name | FC | padj | Gene name | FC | padj | Gene name | FC | padj |
SOX2 | 0,815 | 0,4215 | CDH1 | 1,626 | 0,0090 | CDH2 | 0,616 | 0,0003 |
SOX4 | 0,784 | 0,0245 | EPCAM | 1,128 | 0,7273 | SNAI1 | 3,379 | 7,14E-06 |
SOX9 | 0,557 | 0,0021 | KRT8 | 2,617 | 1,98E-06 | VIM | 0,663 | 0,0366 |
NOTCH2 | 0,937 | 0,6069 | CLDN4 | 1,203 | 0,3140 | COL2A1 | 1,662 | 0,0849 |
HES1 | 0,663 | 0,0280 | GRHL2 | 1,360 | 0,1920 | COL1A1 | 10,939 | 7,76E-06 |
TBX19 KI and NFKB2 KI alter hypothalamic-pituitary organoid development through distinct mechanisms
The transcription factor RAX, which is physiologically expressed in the hypothalamus, also showed a moderate but significant decrease in its expression in NFKB2 KI organoids (Figure 9C and Table 1), suggesting that the phenotype could at least partially be due to a hypothalamic defect. Indeed, among the growth factors known to mediate the interaction between the hypothalamus and oral ectoderm during pituitary development in animal models, BMP4, FGF8, and FGF10 had fold-changes of 4.35, 0.5, and 0.26 in NFKB2 KI organoids respectively, whereas SHH, WNT5A, and FGF8 expression levels were not modified (Table 1).
In comparing the results of RT-qPCR to those obtained by RNA-seq, we found good correlations (R2=0.7595; p<0.0001) between the NFKB2 KI to WT ratios measured with both techniques on the same samples, except for TBX19 (Figure 9—figure supplement 2). As we obtained similar results with 2 sets of TBX19 RT-qPCR primers, targeting different exons, and with at least one primer hybridizing over the junction of two exons, we believe the RNA-seq results for this gene to be likely biased, though we have not identified the reason.
Comparing gene expression levels between TBX19 KI and NFKB2 KI organoids of developmentally important transcription and growth factors shows their differences. As mentioned before, HESX1 was upregulated at d48 only in NFKB2 KI organoids, whereas both mutants had decreased levels of PITX1 and LHX3, the latter being more severely impacted in NFKB2 KI organoids (Figure 10A). In these, we confirmed the increase in BMP4 and decrease in FGF8/FGF10 expression seen in RNA-seq. We did not find any significant differences in these transcripts between WT and TBX19 KI organoids (Figure 10B), suggesting that the impaired development of pituitary progenitors in this model has a different mechanism.
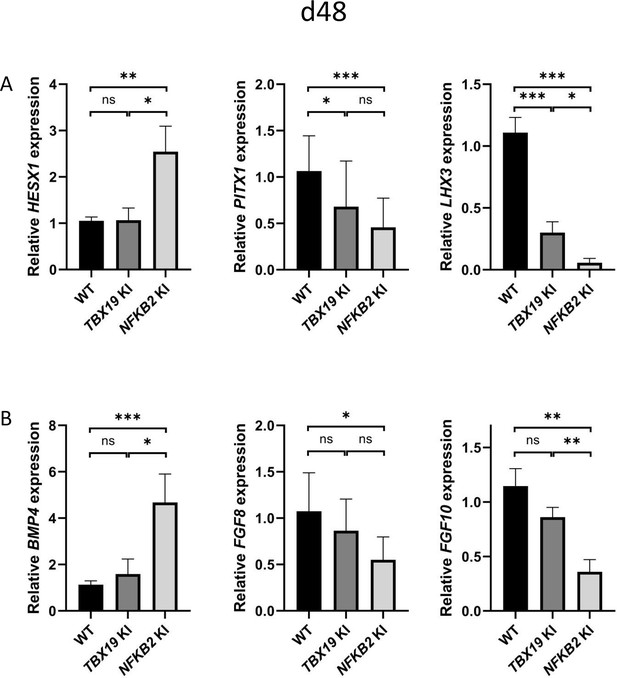
Impaired pituitary progenitor development is correlated with changes in growth factor expression in nuclear factor kappa-B subunit 2 (NFKB2) knock-in (KI) organoids.
(A) RT-qPCR experiments at d48 show that HESX1 expression is upregulated NFKB2 KI organoids, compared to wild-type (WT) and TBX19 KI, whereas PITX1 and LHX3 are downregulated in both mutants. (B) BMP4 expression is increased whereas, and FGF8 and FGF10 are decreased in NFKB2 mutants, but unchanged in TBX19 KI organoids. Data show means ± SEM; n=17, 12, and 11 for WT, TBX19 KI, and NFKB2 KI, respectively. Mann-Whitney t-test (unpaired, two-tailed, nonparametric). p<0.05 (*), p<0.01 (**), p<0.005 (***),.
We then explored markers of different stages of corticotroph maturation that were recently identified in human fetal pituitary (Zhang et al., 2020). RNA-seq on d48 NFKB2 KI organoids showed increased expression of FST, a gene expressed in immature corticotropes, and concomitant decreased levels of fully mature corticotrope markers AR, NR4A2, PCSK1, and POMC (0.63, 0.4, 0.35, and 0.43-fold changes, respectively) (Table 1). RT-qPCR at d48, d75, and d105 showed that TBX19 was upregulated at later stages in both models, suggesting that both TBX19 and p52 are involved in the control of TBX19 expression (Figure 11A). The NR4A2 transcription factor was downregulated at d75 in both mutants, but strongly upregulated in TBX19 KI organoids and downregulated in NFKB2 KI organoids at d105 (Figure 11B). PCSK1 expression, necessary for prohormone processing, also decreased in both mutants, but this phenomenon started earlier in NFKB2 KI organoids (Figure 11C). Finally, we found that POMC expression was as strongly impaired in NFKB2 KI organoids as in TBX19 KI organoids. These results, combined with normal expression levels for NEUROD1, corroborate the hypothesis that TBX19-positive precursors fail to achieve terminal differentiation in NFKB2 KI organoids.
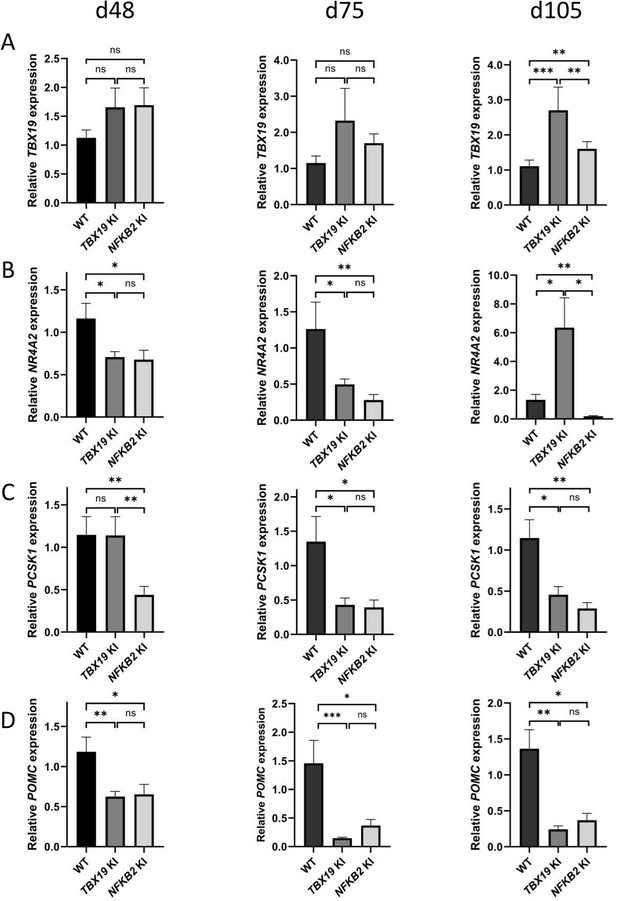
Impaired differentiation of corticotrophs in TBX19 KI and nuclear factor kappa-B subunit 2 (NFKB2) knock-in (KI) organoids.
(A) RT-qPCR measurements of TBX19 expression show a late (d105) increase in both mutants, with a stronger effect in TBX19 KI organoids. (B) Corticotroph terminal differentiation marker NR4A2 was decreased in both mutants at d48 and d75, but only in NFKB2 mutants at d105. (C) PCSK1 downregulation is only observed in NFKB2 KI at d48, and in both models at d75 and d102. (D) A decrease in pro-opiomelanocortin (POMC) expression is observed in both mutants from d48 onwards. Data show means ± SEM; d48: n=17, 12, and 11; d75, n=9, 7, and 6; d105: n=9, 5, and 5 for WT, TBX19 KI, and NFKB2 KI organoids, respectively. Mann-Whitney t-test (unpaired, two-tailed, nonparametric). p<0.05 (*), p<0.01 (**), p<0.005 (***).
Interestingly, other lineages also appeared to be affected, both in TBX19 KI and NFKB2 KI organoids. RNA-seq at d48 showed drastic downregulation of PROP1 and POU1F1 transcription factors in NFKB2 mutants. Although we could not accurately measure POU1F1 expression at d48 due to its too low expression, RT-qPCR (Figure 12) showed that PROP1 downregulation was also occurring in TBX19 KI organoids at d48 (Figure 12A, left), and both PROP1 and POU1F1 levels were barely detectable at d75 in the two models (Figure 12A and D). This is likely to be the consequence of the impaired generation of pituitary progenitors described above. Nonetheless, other transcription factor markers of POU1F1-dependent lineages were expressed, as shown by normal expression of NEUROD4 and ZBTB20 in TBX19 KI organoids at d48 (Figure 12B and C, left). However, both genes had lower expression levels at 75 compared to WT (Figure 12B and C, right). In NFKB2 KI organoids, NEUROD4 was downregulated at both time points (Figure 12B), and ZBTB20 at d48 only (Figure 12C, left), with signs of recovery at d75 (Figure 12C, right) and d105 (data not shown). Again, these results suggest that different mechanisms converge on impaired POU1F1-dependent lineage development.

POU1F1-dependent lineages are affected in TBX19 KI and nuclear factor kappa-B subunit 2 (NFKB2) KI organoids.
(A) Strong downregulation of PROP1 expression is observed at d48 in both mutants and persists at d75. (B) Expression of POU1F1-dependant lineage progenitors and mature somatotrophs marker NEUROD4 is decreased at d48 inNFKB2 organoids, and in both models at d105. (C) ZBTB20, a marker for POU1F1-dependant lineage progenitors and mature lactotrophs, is less expressed in NFKB2 KI organoids at d48, but at normal levels by d75. In TBX19 KI mutants, its expression is normal at d48 but decreased by d75. (D) On d75, POU1F1 expression is barely detectable in either model. Data show means ± SEM; d48: n=17, 12, and 11; d75: n=9, 7, and 6 for WT, TBX19 KI and NFKB2 KI organoids, respectively. Mann-Whitney t-test (unpaired, two-tailed, nonparametric). p<0.05 (*), p<0.01 (**), p<0.005 (***).
Finally, the glycoprotein hormone common alpha subunit CGA was strongly upregulated at d48 (Table 1) and d75 (data not shown) in NFKB2 KI organoids. The concomitant increase in GATA2, CYP11A1, and FOLR1 may suggest a redirection of some progenitors towards a gonadotroph/thyrotroph cell fate. However, the lack of changes in the expression of NR5A1, NEUROD1, and SOX11, and the undetectable transcripts for the specific beta subunits FSHB, LHB, and TSHB before d105, do not favour this hypothesis.
A few years ago, ChIP-seq experiments on Hodgkin lymphoma cells (de Oliveira et al., 2016) identified 10,893 DNA-binding regions for NFKB2 p52, within or in the close vicinity of 5,497 genes. About half of the p52 recruitments occurred in transcribed regions, with a high percentage within introns, and the other half in intergenic regions close to a transcriptional start site (TSS). Among the 4819 differentially regulated genes identified in NFKB2 KI organoids at d48, 1398 of them had p52 binding regions nearby (Figure 13), of which 23 belong to our curated list of pituitary genes of interest. Among them, we found genes crucial for progenitor development (SOX9, PITX1), EMT (CDH1, ZEB2), POU1F1-dependent lineages (PROP1, NEUROD4), and most importantly, the principal genes of corticotroph development and maturation (TBX19, NR4A2, PCSK1, POMC). Thus, these changes in the expression of these genes we observed in NFKB2 KI organoids may be due to a direct effect on transcriptional regulation.
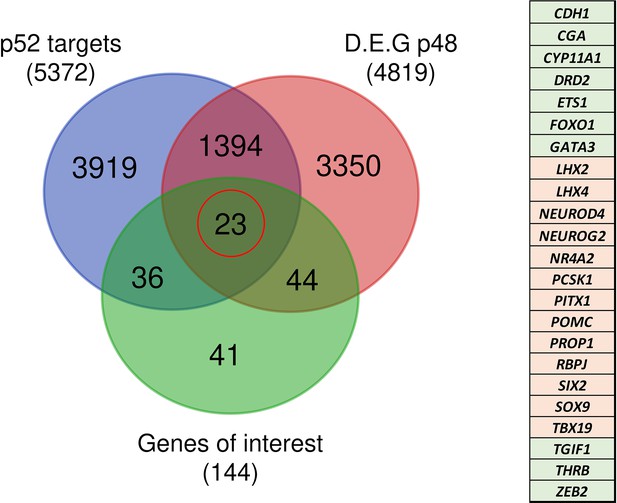
Identification of genes potentially under direct p52 regulation.
Venn diagram showing the total number and intersections of genes identified as p52 targets, genes differentially expressed at d48 between wild-type (WT) and nuclear factor kappa-B subunit 2 (NFKB2) KI organoids, and a literature-curated list of genes particularly implicated in hypothalamus-pituitary development. The table on the right shows the identity of the 23 genes (circled in red), with color code indicating whether they are upregulated (green) or downregulated (pink) in NFKB2 KI mutants at d48.
Altogether, our data identify the NFKB2 signaling pathway as an important factor acting at multiple levels on human pituitary development.
Discussion
After the description of DAVID syndrome as a rare combination of anterior pituitary deficit, mostly ACTHD, with common variable immunodeficiency, we and others found that it is associated with NFKB2 gene mutations affecting specific C-terminal residues of the NFKB2 protein, known to play important roles in immunity and also expressed in the pituitary (Quentien et al., 2012; Chen et al., 2013; Brue et al., 2014). As the mouse model harboring a similar alteration of the ortholog gene lacked an endocrine phenotype (Brue et al., 2014), we wanted to investigate the mechanism of ACTHD in this syndrome. Currently, available in vitro models to study ACTHD and CPHD have strong limits. For instance, studies based on transfections and luciferase-coupled hormone promoters can only give indirect evidence of the effect of a variant of unknown significance as they are based on partly arbitrary amounts of DNA and promoters in an artificial context (Jullien et al., 2019). Murine models only partially replicate human CPHD, as shown for PROP1 mutations and the occurrence of ACTHD (absent in mice but present in 40% of humans) (Castinetti et al., 2016). In the past decade, the reprogramming of differentiated adult cells into induced pluripotent stem cells and advancements in genome editing technologies using CRISPR/Cas9 systems have broadened possibilities for modeling novel aspects of human disease ex vivo (Randolph et al., 2017). Organoid culture has become a powerful tool for modeling otherwise inaccessible congenital human disorders in many organs such as brain (Arnaud et al., 2022), intestine (Schwank et al., 2013), kidney (Takasato et al., 2015), liver (Guan et al., 2017), pancreas (Moreira et al., 2018), ovary (Nanki et al., 2020), and lung (Kong et al., 2021). In the present study, we established a human in vitro model of congenital ACTHD using 3D pituitary organoids differentiated from TBX19 KI and NFKB2 KI hiPSC generated by CRISPR/Cas9 genome editing, in order to compare them with their WT equivalents on the same genetic background. After confirming that our model could replicate pituitary development, we then determined whether it could also replicate a disease, namely ACTHD. As the TBX19K146R homozygous variant led to ACTHD in humans, we designed a human ‘KI’ organoid model derived from TBX19K146R/K146R hiPSC. In line with the Tbx19-/- mouse model (Pulichino et al., 2003), TBX19 KI organoids displayed markedly defective corticotroph differentiation, as compared to WT organoids, thereby validating the methodological approach. Interestingly, in organoids carrying a TBX19K146R/K146R missense mutation that affects DNA binding, a few corticotrophs were still present, suggesting either a role for TBX19 independent of its DNA binding activity or persistence of residual DNA binding activity with this mutation. This model also raises the possibility for a previously undescribed role of TBX19 during early development and the generation of pituitary progenitors in human. However, as TBX19 mutations have only rarely been associated with other pituitary deficits - especially transient GHD (McEachern et al., 2011), further investigations will be needed.
Using this same approach with NFKB2D865G/D865G human organoids generated by CRISPR/Cas9 genome edited hiPSC, we demonstrated for the first time a role for NFKB2 in pituitary development. Heterozygous mutations in the C-terminal region of NFKB2, such as D865G, were reported in DAVID syndrome, leading to the disruption of both non-canonical and canonical pathways (Chen et al., 2013; Kuehn et al., 2017; Lee et al., 2014; Lindsley et al., 2014; Liu et al., 2014; Maccari et al., 2017). Even though NFKB2D865G was identified in a heterozygous state in patients with ACTHD, we decided to use a homozygous model of NFKB2 KI to determine whether NFKB2 was involved in pituitary development and could explain the defect in ACTH production in DAVID syndrome (OMIM#, 615577) (Quentien et al., 2012; Chen et al., 2013; Mac et al., 2023). Indeed, while concomitant common variable immune deficiency (CVID) can be explained by the key roles of NFKB signaling in the immune system, the mechanism of the endocrine deficits caused by NFKB2 mutants was unknown. In mouse models, deletion of Nfkb2 causes abnormal germinal center B-cell formation and differentiation (Carragher et al., 2004). However, the Lym1 mouse model, which carries a truncating variant Y868* in the Nfkb2 orthologue that prevents its cleavage into a transcriptionally active form, had apparently normal corticotroph function and ACTH expression in the pituitary in both heterozygotes and homozygotes (Brue et al., 2014), despite reduced fertility and more severe immune abnormalities in mutant NFKB2 homozygotes (Tucker et al., 2007). Using pituitary organoids differentiated from a NFKB2 KI hiPSC line in comparison with its unmutated control and in the absence of an immune response, we have demonstrated that human corticotroph development is directly disrupted by the missense mutation found in DAVID syndrome. The absence of global alteration of the NFKB signaling pathway shows that mutant p100/p52 protein is directly responsible for the observed phenotype.
The precise molecular mechanisms by which NFKB2 mutation impairs corticotroph development remain to be determined. However, our results (summarized in Figure 14) suggest both early effects on the maturation of pituitary progenitors, on epithelial to mesenchymal transition, and late effects on corticotroph differentiation. First, The NFKB2 gene product p52 could be involved in the early steps of pituitary development. RNA-seq data on day 48 of NFKB2 KI organoid development showed increased HESX1 and BMP4 transcription, in favor of a blockade in an undifferentiated state evocative of the Rathke’s pouch epithelial primordium: indeed, down-regulation of HESX1 is necessary for proper pituitary development (Cohen et al., 2003). This is also suggested by RNA-seq results showing downregulation of PITX1, HES1, LHX3, and FGF10 in mutant organoids, whereas their expression is necessary for progression to a progenitor state (Ohuchi et al., 2000; Ericson et al., 1998). The shift in the BMP4/FGF10 balance favors the idea that the impaired differentiation of pituitary progenitors is at least in part of hypothalamic origin. Our results also suggest a disorganized EMT process, and the need for a functional p100/p52 to mediate the action of EMT inducers on CDH1 downregulation. Finally, concordant with the hypothesis of a differentiation blockade, upregulation of epithelial markers such as CDH1 and KRT8 was observed in mutant NFKB2 KI organoids on day 48. In contrast, RNA-seq showed no change in Wnt and Hedgehog signaling pathways in NFKB2 KI organoids.
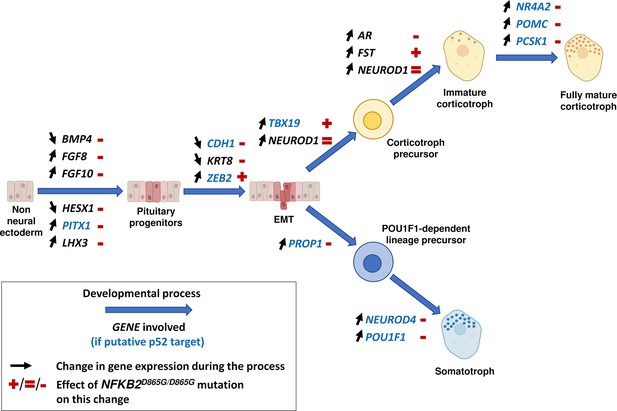
Changes in gene expression induced by nuclear factor kappa-B subunit 2 (NFKB2)D865G/D865G mutation during hypothalamic-pituitary organoid development.
Diagram illustrating changes in gene expression (black arrows) associated with different stages of normal hypothalamic-pituitary organoids development and cell differentiation (large blue arrows). The NFKB2D865G/D865G mutation perturbs these changes (+/=/- signs in red), possibly through direct transcriptional control for genes indicated in blue.
The NFKB2 gene product p52 could also be involved in the final steps of corticotroph differentiation. While qRT-PCR showed increased levels of TBX19 mRNA in mutant organoids, this did not lead to the expected increase in POMC mRNA transcripts. Moreover, some NFKB2 mutants had TBX19 + cells without ACTH expression. This suggests that p52 is necessary for POMC synthesis, which NFKB2D865G may prevent. This could be due to an abnormal DNA binding of the NFKB heterodimer to the POMC promoter (Giraldi et al., 2011; Drouin, 2016), but as other NFKB binding sites are found in intronic regions, it may also be involved in changes in chromatin structures required for proper transcription. The same possibilities apply to PCSK1, the gene coding for the enzyme necessary for POMC to ACTH conversion, and to other genes involved in corticotroph maturation. Besides, NFKB2D865G could also block TBX19 + cells in a pre-differentiated state of corticotroph cells expressing FST through its derepression of chromatin remodeling factors such as EZH2 (De Donatis et al., 2016).
GH deficiency has been reported in some patients with heterozygous NFKB2 mutations (Mac et al., 2023). The drastic effect on POU1F1 expression we observed is likely to be the consequence of different phenomena. First, the impaired development of progenitors, and the disruption of PROP1 expression, possibly due to the absence of p52. Second, both FOXO1 and NEUROD4 have p52 binding regions and were differentially expressed. Alongside POU1F1, these two genes have been described as crucial members of a feedforward loop controlling somatotroph function (Stallings et al., 2024). The 12-fold increase in CGA expression and increased expression of pre-gonadotropes or mature thyrotropes (GATA2) and mature gonadotropes (GATA2, CYP11A1, and FOLR1) could be an argument in favor of a redirection of pituitary progenitors towards these lineages. However, the lack of differential expression of NR5A1 contradicts this hypothesis. A more likely explanation is that CGA repression by TBX19 may directly require p52.
The organoid model described in this article presents several advantages over other approaches. It is a true human model of ACTHD, emphasizing the limits of mouse models, as exemplified by the Lym1 model, which showed that Nfkb2 p100/p52 function was not essential for pituitary development in mice, but did not provide insights for humans. Nfkb2Lym1/Lym1 mice and our NFKB2 KI model have different but functionally very similar mutations, as they both lead to an abnormal processing of p100 with its accumulation leading to a strong reduction of p52 content. The phenotype of Nfkb2Lym1/Lym1 being more severe than in mice lacking the complete NFKB2 protein (Nfkb2-/-), these dominant-negative mutations have been called ‘super repressors’ (Tucker et al., 2007). In light of our results, it is surprising that no pituitary deficiencies have been observed in Nfkb2-/- or Nfkb2Lym1/Lym1. This suggests that in humans, NFKB2 plays an important role during pituitary development, therefore, constituting a major inter-species difference. Studying p52 binding regions across different species may help understand how this role appeared during evolution.
All patients with DAVID syndrome harbour heterozygous NFKB2 mutations. Whether this reflects embryonic lethality of the mutation at the homozygous state or just the rarity of this genotype is still an unresolved question. However, ‘super repressor’ mutations have been shown to disturb both canonical and non-canonical signalling pathways even when heterozygous (Wirasinha et al., 2021; Kuehn et al., 2017). As our results show impaired POU1F1-dependent lineage development in addition to the severe corticotroph deficit, further experiments on organoids derived from NFKB2WT/D865G hiPSCs should tell if there are differences in the sensitivity of different lineages to the dominant-negative protein. If so, this could give us clues about why DAVID patients suffer from isolated ACTHD in their vast majority rather than CPHD.
Despite some technical limits, CRISPR/Cas9-based genome editing of hiPSC is a powerful approach to elucidate gene function in congenital pituitary deficiency patients, as was shown for the role of hypothalamic OTX2 regulation of pituitary progenitor cells in congenital pituitary hypoplasia (Matsumoto et al., 2019). While the prevalence of pathogenic variants of known genes in primary hypopituitarism is currently estimated at below 10% (Jullien et al., 2021), exome and whole-genome sequencing regularly identify new genes and variants, for which pathogenicity remains uncertain (Dobin et al., 2013). This model could thus become in the following years the gold standard to confirm the involvement of a given gene in pituitary development, or to classify new variants of unknown significance as likely benign or pathogenic. Finally, pituitary organoids derived from human embryonic stem cells have been transplanted subcutaneously into mice with hypopituitarism; grafted cells were able to secrete ACTH and respond to corticotropin-releasing hormone stimulation (Sasaki et al., 2023), paving the way for innovative substitutive treatments in patients with hypopituitarism (Taga et al., 2023). Conversely, a limitation of this model is the long duration of the differentiation period (approximately 3 months) and the fact that not all hiPSC clones lead to full differentiation of hypothalamic-pituitary organoids despite similar conditions of culture. For these reasons, we could not include confirmation of our results on an independent clone in the present paper.
In conclusion, we have designed and validated a model replicating human constitutive ACTHD using a combination of CRISPR/Cas9 and directed differentiation of hiPSC into 3D hypothalamic-pituitary organoids. Not only has this proof-of-concept reproduced the known prerequisite for the TBX19 transcription factor in human corticotroph differentiation, but it has also allowed a new classification of a NFKB2 variant of previously unknown clinical significance as pathogenic in pituitary development.
From a clinical viewpoint, this organoid model could provide evidence for the pathogenic role of new candidate genes or variants investigated in patients where none other is available, especially in a rare disease affecting a poorly accessible organ like the pituitary gland. From a physiological viewpoint, modeling corticotroph deficiency using hiPSC allows us to find evidence for a functional role for NFKB2 in human pituitary differentiation.
Future studies investigating the direct role of p52 on transcription and changes in chromatin landscape during pituitary development will be necessary to elucidate its precise mechanism of action, as well as the level of pathogenicity of the NFKB2D865G mutations in its heterozygous form.
Materials and methods
Reagent type (species) or resource | Designation | Source or reference | Identifiers | Additional information |
---|---|---|---|---|
Cell line (Homo sapiens) | Human induced pluripotent stem cells | MaSC Platform, MMG UMR1251, INSERM-AMU | 10742 L | Reprogrammed from fibroblasts (Coriell, female, 82yo) |
Other | Synthemax II-SC Substrate | Corning | #3335 | Cell culture matrix |
Other | StemMACS hiPSC-Brew XF human medium | MACS Miltenyi Biotec | #130-104-368 | Cell culture medium |
Other | Knockout Serum Replacement | Thermo Fisher Scientific | #10828028 | Cell culture reagent |
Recombinant protein | Human BMP-4 | Peprotech | #AF-120-05ET | |
Drug | Smoothened Agonist | Sigma-Aldrich/Merck | #SML 1314 | |
Peptide | AlbumiNZ Bovine Albumin Low IgG | MP Biomedicals | #0219989790 | |
Sequence-based reagent | ligonucleotides used for CRISPR/Cas9 experiments to target TBX19K146R | This paper | TBX19 sgRNA | AAGCTGACCAACAAGCTCAA |
Sequence-based reagent | ligonucleotides used for CRISPR/Cas9 experiments to target TBX19K146R | This paper | TBX19 sgRNA oligo up | 5’-CACCGAAGCTGACCAACAAGCTCAA-3’ |
Sequence-based reagent | ligonucleotides used for CRISPR/Cas9 experiments to target TBX19K146R | This paper | TBX19 sgRNA oligo down | 5’-AAACTTGAGCTTGTTGGTCAGCTTC-3’ |
Sequence-based reagent | ligonucleotides used for CRISPR/Cas9 experiments to target TBX19K146R | This paper | TBX19 ssODN | TGGATGAAAGCTCCCATCTCCTTCAG CAAAGTGAGGCTGACCAACAAGTTaAA TGGAGGCGGGCAGGTACGAATGAGG CGGGCAGGCCTGGCCACCCGCT |
Sequence-based reagent | primers used for PCR analyses, CAPS assay, and Sanger Sequencing | This paper | TBX19 fwd | CCCCTGGACAAGGTGAGAGTT |
Sequence-based reagent | primers used for PCR analyses, CAPS assay, and Sanger Sequencing | This paper | TBX19 rev | GACTCCCGGGAATAATTGGCTTC |
Sequence-based reagent | ligonucleotides used for CRISPR/Cas9 experiments to target NFKB2D865G | This paper | NFKB2 sgRNA | GTGAAGGAAGACAGTGCGTA |
Sequence-based reagent | ligonucleotides used for CRISPR/Cas9 experiments to target NFKB2D865G | This paper | NFKB2 sgRNA oligo up | 5’-CACCGTGAAGGAAGACAGTGCGTA-3’ |
Sequence-based reagent | ligonucleotides used for CRISPR/Cas9 experiments to target NFKB2D865G | This paper | NFKB2 sgRNA oligo down | 5’-AAACTACGCACTGTCTTCCTTCAC-3’ |
Sequence-based reagent | ligonucleotides used for CRISPR/Cas9 experiments to target NFKB2D865G | This paper | NFKB2 ssODN | TCCCATTCCTGTCCCCATTTACCCCC AGCAGAGGTGAAGGAAGGCAGTGCC TACGGGAGCCAGTCAGTGGAGCAGG AGGCAGAGAAGCTGGGCCCACCCC |
Sequence-based reagent | primers used for PCR analyses, CAPS assay, and Sanger Sequencing | This paper | NFKB2 fwd | CCCTAACCATGACTCAGACCTCA |
Sequence-based reagent | primers used for PCR analyses, CAPS assay, and Sanger Sequencing | This paper | NFKB2 rev | CCTCCCCTTCCCATGAGAATCC |
Antibody | Anti-human NFKB2 (p100/p52) Rabbit polyclonal | Sigma, | HPA008422 RRID:AB_1854434 | (1/500) |
Antibody | Anti-human LHX3 Mouse monoclonal | DSHB | 67.4E12 RRID:AB_2135805 | (1/500) |
Antibody | Anti-human TBX19 Rabbit polyclonal | Sigma, | HPA072686 RRID:AB_2732209 | (1/300) |
Antibody | Anti-human ACTH Guinea pig polyclonal | NIDDK | (1/2000) | |
Antibody | Anti-human E-human cadherin Rat monoclonal | Millipore, | MABT26 RRID:AB_10807576 | (1/500) |
Antibody | Anti-human NKX2.1 Mouse monoclonal | Millipore, | MAB5460 RRID:AB_571072 | (1/500) |
Antibody | Anti-human PITX1 Rabbit polyclonal | Sigma, | HPA008743 RRID:AB_1855413 | (1/500) |
Culture and maintenance of hiPSC lines
Request a detailed protocolThe 10742 L healthy individual-derived hiPSC line was used in the study as the WT control (provided by the Cell Reprogramming and Differentiation Facility [MaSC], Marseille Medical Genetics, Marseille, France). Information about this hiPSC line is indicated in Supplementary file 1a. Two ‘knock-in’ mutant lines carrying TBX19K146R/K146R (thereafter also designated as TBX19 KI) and NFKB2D865G/D865G (thereafter also abridged as NFKB2 KI) were generated using CRISPR/Cas9 editing from the isogenic control line. All hiPSC lines were cultured on six-well plates (Corning, #3335, New York, USA) coated with Synthemax II-SC Substrate (working concentration at 0.025 mg/mL, Corning, New York, USA) and maintained undifferentiated in a chemically defined growth medium (StemMACS hiPSC-Brew XF human medium; MACS Miltenyi Biotec, Paris, France) (Giobbe et al., 2015). hiPSC lines were maintained in a humidified incubator under conditions of 37 °C, 5% CO2, with a daily change of medium, and passaged when cells reached 60–80% confluency using enzyme-free ReLeSR according to manufacturer’s recommendations (StemCell Technologies, Canada, #05872).
CRISPR/Cas9 mediated genome editing of hiPSC
Request a detailed protocolCRISPR/Cas9 gene editing was used to create the TBX19K146R/K146R and the NFKB2D865G/D865G mutations using the method as previously described (Arnaud et al., 2022).
CRISPR/Cas9 was used to generate two mutant hiPSC lines from 10742 L hiPSC:
The TBX19K146R/K146R line, harboring the c.437A>G, p.Lys146Arg TBX19 (NM_005149) pathogenic missense variant (Couture et al., 2012).
The NFKB2D865G/D865G line, harboring the c.2594A>G, p.Asp865Gly NFKB2 (NM_001322934) pathogenic missense variant.
Preparation of the CRISPR/Cas9 sgRNA plasmid
Request a detailed protocolFor each targeted gene, sgRNA plasmid was prepared as previously described (Ran et al., 2013). Briefly, we designed a sgRNA sequence within 20 nucleotides from the target site selected with the open-source CRISPOR tool (http://crispor.tefor.net/) (Concordet and Haeussler, 2018).
The sgRNA sequence used to generate TBX19K146R was (5’>3’): AAGCTGACCAACAAGCTCAA (see also Supplementary file 1b).
The sgRNA sequence used to generate NFKB2D865G was (5’>3’): GTGAAGGAAGACAGTGCGTA (see also Supplementary file 1c).
We used the cAB03 (also known as pX459-pEF1alpha) vector as previously described (Arnaud et al., 2022). Briefly, pSpCas9 (BB)–2A-Puro (PX459) V2.0 (Addgene plasmid # 62988, Addgene, Teddington, UK) was modified by the EF1 alpha promoter (Arnaud et al., 2022). The chosen sgRNA was cloned into the cAB03 open plasmid vector using the method previously described by Arnaud et al., 2022. The final vector expressed the corresponding sgRNA under the control of the U6 promoter, as well as Cas9 under the control of the EF-1alpha promoter, and a puromycin resistance gene.
Single-stranded oligo DNA nucleotides (ssODN) design
Request a detailed protocolWe also designed donor sequences to generate TBX19K146R and NFKB2D865G missense mutations. A donor sequence is used for homology-directed repair, allowing the introduction (‘knock-in,’ KI) of single nucleotide variations, with low efficiency. To optimize the efficiency of KI editing, the donor sequences were designed as ssODN, carrying the mutation of interest, and also included a silent (or blocking) mutation that mutates the protospacer-adjacent motif (PAM) or disrupts the sgRNA binding region to prevent re-cutting by Cas9 after successful editing (Paquet et al., 2016; Edmondson et al., 2021; Arnaud et al., 2022; Kwart et al., 2017). The ssODN contained 100 nucleotides with homology arms on each side of the target region. The ssODN sequence was as follows (see also Supplementary file 1b and Supplementary file 1c):
For TBX19K146R (5’–3’): TGGATGAAAGCTCCCATCTCCTTCAGCAAAGTGAGGCTGACCAACAAGTTAAATGGAGGCGGGCAGGTACGAATGAGGCGGGCAGGCCTGGCCACCCGCT.
For NFKB2D865G (5’–3’):
TCCCATTCCTGTCCCCATTTACCCCCAGCAGAGGTGAAGGAAGGCAGTGCCTACGGGAGCCAGTCAGTGGAGCAGGAGGCAGAGAAGCTGGGCCCACCCC.
The ssODN were synthesized by Integrated DNA Technologies (Coralville, Iowa, US) at Ultramer quality.
Electroporation
Request a detailed protocolhiPSC were transfected with constructed sgRNA/Cas9 vectors by electroporation using the Neon Transfection System 100 µL kit (Invitrogen). A summary of the procedure is described in Figure 1—figure supplement 1.
Clone isolation and screening
Request a detailed protocolOn day 3 post-transfection, we used a cleaved amplified polymorphic sequences (CAPS) assay to estimate the efficacy of CRISPR/Cas9 in bulk transfected hiPSC populations to choose the number of colonies to be picked (Zischewski et al., 2017). A restriction enzyme recognition site is inserted in the donor template. CAPS primers and restriction enzymes were determined with the help of AmplifX software (version 2.0.0b3). CAPS primers were listed in Supplementary file 1d. The CAPS products were separated and visualized by electrophoresis on a 2% agarose gel and analyzed for densitometry with ImageJ (Arnaud et al., 2022; Kwart et al., 2017).
After 10 d, the CAPS assay was also used to screen and identify the possible KI clones. KI clones were screened by CAPS assay and confirmed by Sanger sequencing (Genewiz, Leipzig, Germany). Analyses of Sanger traces were done by Sequencher DNA sequence analysis software (version 5.4.6, Gene Codes Corporation, Ann Arbor, MI USA, http://www.genecodes.com) to align sequences of KI clones with WT sequence to confirm the successful edition. Sanger sequencing primers are described Supplementary file 1d. KI clones were kept, amplified, and then cryopreserved.
In vitro pituitary organoids differentiated from hiPSC
Request a detailed protocolPituitary organoids were produced at the same time, using the same protocol for WT and edited hiPSC lines. Two batches of differentiation with two hundred organoids for each hiPSC line were generated:
Batch 1: WT organoids versus TBX19 KI organoids
Batch 2: WT organoids versus NFKB2 KI organoids
The differentiation protocol was based on a protocol from Matsumoto et al. with some modification (Matsumoto et al., 2019; Sasai et al., 2012). hiPSCs were first dissociated into single cells using Accutase. Ten thousand cells per organoid were plated in 20 µL hanging drop under the lid of a 127.8×85.5 mm single-well plate (Greiner bio-one) in growth factor-free chemically defined medium (gfCDM) supplemented with 10% (vol/vol) Knockout Serum Replacement (KSR; Thermo Fisher Scientific) and 20 µM Y-27632. The lid was inverted over the bottom chamber (filled with 10 mL of sterile water to avoid the evaporation of droplets) and incubated at 37 °C/5% CO2 for 2 d. Once aggregates formed, organoids were transferred to non-adhesive 100x20 mm culture dishes (Corning) containing 10 mL of gfCDM medium supplemented with 10% KSR without Y-27632 and incubated at 37 °C and 5% CO2 until day 6. From days 6 to days 17, the medium was supplemented with 10% KSR, 5 nM recombinant human bone morphogenetic protein 4 (BMP4; Peprotech, # AF-120-05ET, USA) and 2 μM Smoothened agonist (SAG; Sigma-Aldrich/Merck, # SML 1314). Half of the medium was renewed every 2–3 d.
From day 18, BMP4 was withdrawn and half of the medium was renewed every 2–3 d. At this point, organoids were maintained under high-O2 conditions (40%) and 5% CO2 in an MCO-5M incubator (Panasonic). From day 30, gfCDM medium supplemented with 20% KSR was used, and all medium was renewed every 2–3 d until at least day 105. Induction into pituitary progenitor cells (defined as LHX3+) and into pituitary hormone-producing cells was evaluated by qRT-PCR (on days 0, 6, 18, 27, 48, 75, 105) and immunofluorescence (on days 48 and 105) (see below).
Validating TBX19K146R targeting and assessing off-target mutations by whole genome sequencing (WGS)
Request a detailed protocolFor TBX19K146R, we used WGS to assess on-target and off-target mutations, because the edited site is far from the cut site (15 bases). DNA extracted from control and TBX19 KI (5 organoids each) was submitted for WGS to an average depth of 15 X (Integraged Genomics platform). PCR-free libraries were prepared with the NEBNext Ultra II DNA Library Prep Kits (New England BioLabs) according to supplier recommendations. Specific double-strand gDNA quantification and a fragmentation (300 ng of input with high-molecular-weight gDNA) sonication method were used to obtain approximately 400 bp fragments. Finally, paired-end adaptor oligonucleotides (xGen TS-LT Adapter Duplexes from Integrated DNA Technologies) were ligated and re-paired. Tailed fragments were purified for direct sequencing without a PCR step. DNA PCR-free libraries were sequenced on paired-end 150 bp runs on the NovaSeq6000 (Illumina) apparatus. Image analysis and base calling were performed using the Illumina Real Time Analysis (RTA) Pipeline with default parameters. Sequence reads were mapped on the Human Genome Build (GRCh38) using the Burrows-Wheeler Aligner (BWA) (Li and Durbin, 2009). Variant calling was performed via the GATK Haplotype Caller GVCF tool (GATK 3.8.1, Broad Institute) (Poplin et al., 2018). Mutation enrichment was determined using Fisher’s Exact Test. Variants were annotated with Ensembl’s Variant Effect Predictor, (VEP) by Integragen Genomics (McLaren et al., 2016). NGS analyses confirmed the presence of on-target mutation and the absence of off-target mutation in the top four predicted off-target sites.
Validating NFKB2D865G targeting, assessing off-target mutations, and differential expression analysis by bulk RNA sequencing (RNA-seq)
Request a detailed protocolFor NFKB2D865G, we used bulk RNA-seq for analysis of differential RNA expression, and to assess on-target and off-target mutations, because the target site is close to the cleavage site (6 bases). A total of 500 ng of total RNA was extracted from 10 individual organoids (five NFKB2 KI and five control organoids). RNA-seq libraries were generated using the KAPA mRNA HyperPrep kit (Roche). The quality and profile of the libraries were visualized on a Bioanalyzer using the High Sensitivity DNA assay (Agilent) and quantified on a Qubit using the dsDNA High Sensibility Kit (Thermo Fisher Scientific). Finally, we performed a 2×76 bp paired-end sequencing on a NextSeq500 (Illumina). After quality control using FastQC (Braham Institute), reads were aligned on the GRCh38 human reference genome using STAR (version 2.7.2b) (Dobin et al., 2013). BAM files were ordered and indexed using Samtools (Danecek et al., 2021). Read counts on genes were determined using StringTie (Pertea et al., 2015). We assessed the presence of desired NFKB2 edits according to the CRISPR sgRNA design by direct visualization of the BAM files and the absence of off-target coding variations by the GATK Haplotype Caller GVCF tool (Poplin et al., 2018). Genes differentially expressed between conditions were identified using the R package DESeq2 (version 1.34.0) (Love et al., 2014) and assigned as such with an adjusted p-value <0.05.
Total RNA isolation and quantitative real-time PCR (qRT-PCR) analysis for assessment of mRNA expression during pituitary organoid development
Request a detailed protocolOrganoids were harvested from the culture, placed on ice and then stored at –80 °C until RNA extraction. The total RNA was extracted from WT, TBX19 KI, and NFKB2 KI organoids using the NucleoSpin RNA Plus XS kit (Macherey-Nagel), and measured on a NanoDrop TM 1000 Spectrophotometer (Thermo Fisher Scientific). Organoid RNAs were extracted on days 0, 6, and 18 from 7 or 8 organoids, or on days 27, 48, 75, and 105 from single organoids (3–8 organoids per group for each time point). cDNA was synthesized from 200 ng total RNA using the mix of M-MLV reverse transcriptase (Invitrogen, UK, #28025013), dNTP 10 mM (Invitrogen, #10297018), RNAseOut Inhibitor (Invitrogen, #10777019) and random primers (Invitrogen, UK, #48190011). Quantitative PCR was performed using iTaq Universal SYBR Green Supermix (Bio-Rad Laboratories), on Quantstudio 5 Real-time PCR system (Thermo Fisher Scientific) and analysed using QuantStudio 5 Design & Analysis Software. The thermal cycling profiles were as follows: initial denaturation at 95 °C for 20 s, followed by 40 cycles of denaturation at 95 °C (3 s), annealing at 60 °C (45 s), and extension at 72 °C (45 s). All samples were assayed in duplicate. The beta-actin (ACTB), glyceraldehyde-3-phosphate dehydrogenase (GAPDH), and beta-tubulin (TUBB) transcript expressions were used as three endogenous reference controls. Target gene expressions were normalized relative to the mean of the housekeeping genes using the delta Ct method. For gene expression kinetics, these values were normalized relative to the highest mean values of WT during 105 d using the comparative 2-ΔΔCt method (Livak and Schmittgen, 2001). Primer sequences used in the experiments are shown in Supplementary file 1g.
Immunofluorescence labeling experiments
Request a detailed protocolAt days 48 and 105, ten of each WT and mutant organoids were fixed using 4% buffered paraformaldehyde in standard phosphate-buffered saline (PBS) for an hour at RT before rinsing in PBS. After overnight incubation in 30% sucrose/PBS (w/v) at 4 °C, organoids were embedded in Surgipath medium (Leica) and snap frozen. Organoids were cryosectioned at 16 µm. After blocking with 0.01% Triton X100, 10% normal donkey serum, and 1% fish skin gelatin in PBS with 5 ng/mL 4',6-diamidino-2-phénylindole (DAPI) for an hour at RT, slides were incubated with primary antibody diluted in antibody solution (PBS, Triton as above, 1% normal donkey serum and 0.1% fish skin gelatin) overnight at 4 °C, washed and incubated in a 1/500 dilution of secondary antibody in PBS for 2 hr at RT. The primary antibodies and dilutions used in this study are summarized in Supplementary file 1h. Secondary antibodies were species-specific conjugates to Alexa Fluor 488, 555, or 647 (Thermo Fisher Scientific). Fluorescence images were obtained using LSM 800 confocal microscopy (Zeiss).
3D imaging of pituitary organoids
Request a detailed protocolWe used a whole-mount immunostaining and clearing protocol adapted from Belle et al., 2017. 4–8 organoids of each genotype (WT, TBX19 KI, or NFKB2 KI) were fixed by immersion in 4% buffered paraformaldehyde (PFA) in PBS overnight at 4 °C. All steps were carried out on a slowly rotating (70 rpm) agitator. After washing in PBS, organoids were either stored at 4 °C or blocked and permeabilized with 5 mL PBSGT for 2 d at RT (PBSGT: 0.2% fish skin gelatin, 0.5% Triton X100 in PBS, NaN3 0.01%). Organoids were incubated with primary antibodies in 3 mL PBSGT (at concentrations used on sections) over 5 d at 37 °C to increase penetration. Organoids were then washed 6 times in an excess of PBSGT at RT, before incubation with secondary antibodies in 3 ml PBSGT at RT overnight. The wash step was repeated. Organoids were then embedded in 1% low-melting temperature agarose in 1 X TAE after it had cooled to about 45 °C.
As adapted from the iDISCO+ protocol (Renier et al., 2016), organoids in agarose blocks were progressively dehydrated from 20%, 40%, 60%, and 80% to 100% methanol for 1 hr at each graded step. After overnight incubation in two parts dichloromethane (DCM, Sigma-Aldrich) to one part 100% methanol, organoids were immersed in 100% DCM for 30 min before changing to 100% dibenzyl ether (DBE; Sigma-Aldrich) for transparization over 2 hr. Samples were maintained in DBE at RT and protected from light in an amber glass vial. Light-sheet fluorescence microscopy (Miltenyi Biotec UltraMicroscope Blaze) was used to acquire z-stacks of optical sections of pituitary organoids at 4 µm intervals, in Ethyl cinnamate (Sigma-Aldrich). After 3D reconstruction with Imaris software (version 9.6, Bitplane), the number of corticotrophs in each organoid was defined by counting individualized cell-sized (7 µm) objects positive for ACTH immunoreactivity with the Spots automatic classifier after setting parameters in a region of interest containing positively labeled WT cells, then applied to all organoids. Using the Surfaces tool of the Imaris software, the volume of organoids was determined based on thresholds corresponding to their respective fluorescence intensities.
Statistical analysis
Request a detailed protocolStatistical analyses were performed and visualized with GraphPad Prism 9.5.0 software (GraphPad Software, Inc). Data are expressed as means ± SEM. Comparisons between the two groups (mutant versus WT) were performed by unpaired two-tailed t-tests (non-parametric Mann-Whitney test). n refers to the number of samples for each experiment outlined in the figure legends. p-values of <0.05 (*), <0.01 (**), and <0.001 (***) were considered statistically significant differences.
Data availability
RNA-seq results are included as a supporting file (Figure 9—source data 1).
References
-
Mechanisms in endocrinology: An update IN the genetic aetiologies of combined pituitary hormone deficiencyEuropean Journal of Endocrinology 174:R239–R247.https://doi.org/10.1530/EJE-15-1095
-
Germline mutations in NFKB2 implicate the noncanonical NF-κB pathway in the pathogenesis of common variable immunodeficiencyAmerican Journal of Human Genetics 93:812–824.https://doi.org/10.1016/j.ajhg.2013.09.009
-
Enhanced repression by HESX1 as a cause of hypopituitarism and septooptic dysplasiaThe Journal of Clinical Endocrinology & Metabolism 88:4832–4839.https://doi.org/10.1210/jc.2002-021868
-
CRISPOR: intuitive guide selection for CRISPR/Cas9 genome editing experiments and screensNucleic Acids Research 46:W242–W245.https://doi.org/10.1093/nar/gky354
-
Phenotypic homogeneity and genotypic variability in a large series of congenital Isolated ACTH-deficiency patients with TPIT gene mutationsThe Journal of Clinical Endocrinology & Metabolism 97:E486–E495.https://doi.org/10.1210/jc.2011-1659
-
Twelve years of SAMtools and BCFtoolsGigaScience 10:giab008.https://doi.org/10.1093/gigascience/giab008
-
STAR: ultrafast universal RNA-seq alignerBioinformatics 29:15–21.https://doi.org/10.1093/bioinformatics/bts635
-
60 years of POMC: Transcriptional and epigenetic regulation OF POMC gene expressionJournal of Molecular Endocrinology 56:T99–T112.https://doi.org/10.1530/JME-15-0289
-
The human POMC gene promoter: Where do we stand?Journal of Endocrinological Investigation 34:454–460.https://doi.org/10.1007/BF03346713
-
Disease modeling using 3D organoids derived from human induced pluripotent stem cellsInternational Journal of Molecular Sciences 19:E936.https://doi.org/10.3390/ijms19040936
-
Heterozygous LHX3 mutations may lead to a mild phenotype of combined pituitary hormone deficiencyEuropean Journal of Human Genetics 27:216–225.https://doi.org/10.1038/s41431-018-0264-6
-
Lung organoids, useful tools for investigating epithelial repair after lung injuryStem Cell Research & Therapy 12:95.https://doi.org/10.1186/s13287-021-02172-5
-
Combined immune deficiency in a patient with a novel NFKB2 mutationJournal of Clinical Immunology 34:910–915.https://doi.org/10.1007/s10875-014-0095-3
-
Novel NFKB2 mutation in early-onset CVIDJournal of Clinical Immunology 34:686–690.https://doi.org/10.1007/s10875-014-0064-x
-
Deficient anterior pituitary with common variable immune deficiency (DAVID syndrome): a new case and literature reportsJournal of Neuroendocrinology 35:e13287.https://doi.org/10.1111/jne.13287
-
Congenital pituitary hypoplasia model demonstrates hypothalamic OTX2 regulation of pituitary progenitor cellsJournal of Clinical Investigation 130:641–654.https://doi.org/10.1172/JCI127378
-
Severe cortisol deficiency associated with reversible growth hormone deficiency in two infants: What is the link?The Journal of Clinical Endocrinology & Metabolism 96:2670–2674.https://doi.org/10.1210/jc.2011-0129
-
The ensembl variant effect predictorGenome Biology 17:122.https://doi.org/10.1186/s13059-016-0974-4
-
Pancreas 3D organoids: Current and future aspects as a research platform for personalized medicine in pancreatic cancerCellular and Molecular Gastroenterology and Hepatology 5:289–298.https://doi.org/10.1016/j.jcmgh.2017.12.004
-
FGF10 acts as a major ligand for FGF receptor 2 IIIb in mouse multi-organ developmentBiochemical and Biophysical Research Communications 277:643–649.https://doi.org/10.1006/bbrc.2000.3721
-
StringTie enables improved reconstruction of a transcriptome from RNA-seq readsNature Biotechnology 33:290–295.https://doi.org/10.1038/nbt.3122
-
Human and mouse TPIT gene mutations cause early onset pituitary ACTH deficiencyGenes & Development 17:711–716.https://doi.org/10.1101/gad.1065603
-
Deficit in anterior pituitary function and variable immune deficiency (DAVID) in children presenting with adrenocorticotropin deficiency and severe infectionsThe Journal of Clinical Endocrinology & Metabolism 97:E121–E128.https://doi.org/10.1210/jc.2011-0407
-
Genome engineering using the CRISPR-Cas9 systemNature Protocols 8:2281–2308.https://doi.org/10.1038/nprot.2013.143
-
Stem cell engineering and differentiation for disease modeling and cell-based therapiesAIMS Cell and Tissue Engineering 1:140–157.https://doi.org/10.3934/celltissue.2017.2.140
-
Genetic screening of combined pituitary hormone deficiency: experience in 195 patientsThe Journal of Clinical Endocrinology and Metabolism 91:3329–3336.https://doi.org/10.1210/jc.2005-2173
-
Subcutaneous transplantation of human embryonic stem cells-derived pituitary organoidsFrontiers in Endocrinology 14:1130465.https://doi.org/10.3389/fendo.2023.1130465
-
FOXO1 regulates expression of Neurod4 in the pituitary glandMolecular and Cellular Endocrinology 583:112128.https://doi.org/10.1016/j.mce.2023.112128
-
A novel mutation in the Nfkb2 gene generates an NF-kappa B2 “super repressor.”Journal of Immunology 179:7514–7522.https://doi.org/10.4049/jimmunol.179.11.7514
-
Nfkb2 variants reveal a p100-degradation threshold that defines autoimmune susceptibilityThe Journal of Experimental Medicine 218:e20200476.https://doi.org/10.1084/jem.20200476
Article and author information
Author details
Funding
Pfizer France (ID#88979183)
- Thierry Brue
AFMTéléthon (MOTHARD Project N° 23408)
- Thierry Brue
The funders had no role in study design, data collection and interpretation, or the decision to submit the work for publication.
Acknowledgements
This study was supported by the ADEREM (Association pour le Développement de la Recherche Médicale au CHU de Marseille), the AFM-Telethon MoThARD grant to TB and by Pfizer (Grant ID#88979183). TTM received funding from France Excellence Scholarship of the French Embassy in Vietnam, from the French Society of Pediatric Endocrinology and Diabetology (SFEDP), and from the Marseille Rare Disease (MarMaRa) Institute of Aix-Marseille University. The authors wish to thank Frederique Magdinier, Natacha Broucqsault, and Claire El Yazidi from the Marseille Medical Genetics (MMG) cell reprogramming & differentiation facility (MaSC); Sébastien Courrier, from the MMG microscopy platform; Valerie Delague, Catherine Aubert, Christel Castro and Camille Humbert from MMG’s Genomics and Bioinformatics (GBiM) platform; Carole Siret, and Mathieu Fallet from the Centre d’Immunologie de Marseille-Luminy (CIML); Ivo Vanzetta and Alberto Lombardini from the Institut de Neurosciences de la Timone (INT) microscopy platform, and Laurie Arnaud and Emmanuel Nivet from the Institut de Neurophysiopathologie (INP), all in Marseille, for their expert assistance, advice or reagents.
Version history
- Sent for peer review:
- Preprint posted:
- Reviewed Preprint version 1:
- Reviewed Preprint version 2:
- Version of Record published:
Cite all versions
You can cite all versions using the DOI https://doi.org/10.7554/eLife.90875. This DOI represents all versions, and will always resolve to the latest one.
Copyright
© 2023, Mac et al.
This article is distributed under the terms of the Creative Commons Attribution License, which permits unrestricted use and redistribution provided that the original author and source are credited.
Metrics
-
- 274
- views
-
- 17
- downloads
-
- 0
- citations
Views, downloads and citations are aggregated across all versions of this paper published by eLife.
Download links
Downloads (link to download the article as PDF)
Open citations (links to open the citations from this article in various online reference manager services)
Cite this article (links to download the citations from this article in formats compatible with various reference manager tools)
Further reading
-
- Cell Biology
- Developmental Biology
Eukaryotic cells depend on exocytosis to direct intracellularly synthesized material toward the extracellular space or the plasma membrane, so exocytosis constitutes a basic function for cellular homeostasis and communication between cells. The secretory pathway includes biogenesis of secretory granules (SGs), their maturation and fusion with the plasma membrane (exocytosis), resulting in release of SG content to the extracellular space. The larval salivary gland of Drosophila melanogaster is an excellent model for studying exocytosis. This gland synthesizes mucins that are packaged in SGs that sprout from the trans-Golgi network and then undergo a maturation process that involves homotypic fusion, condensation, and acidification. Finally, mature SGs are directed to the apical domain of the plasma membrane with which they fuse, releasing their content into the gland lumen. The exocyst is a hetero-octameric complex that participates in tethering of vesicles to the plasma membrane during constitutive exocytosis. By precise temperature-dependent gradual activation of the Gal4-UAS expression system, we have induced different levels of silencing of exocyst complex subunits, and identified three temporarily distinctive steps of the regulated exocytic pathway where the exocyst is critically required: SG biogenesis, SG maturation, and SG exocytosis. Our results shed light on previously unidentified functions of the exocyst along the exocytic pathway. We propose that the exocyst acts as a general tethering factor in various steps of this cellular process.
-
- Cell Biology
- Developmental Biology
In most murine species, spermatozoa exhibit a falciform apical hook at the head end. The function of the sperm hook is not yet clearly understood. In this study, we investigate the role of the sperm hook in the migration of spermatozoa through the female reproductive tract in Mus musculus (C57BL/6), using a deep tissue imaging custom-built two-photon microscope. Through live reproductive tract imaging, we found evidence indicating that the sperm hook aids in the attachment of spermatozoa to the epithelium and facilitates interactions between spermatozoa and the epithelium during migration in the uterus and oviduct. We also observed synchronized sperm beating, which resulted from the spontaneous unidirectional rearrangement of spermatozoa in the uterus. Based on live imaging of spermatozoa-epithelium interaction dynamics, we propose that the sperm hook plays a crucial role in successful migration through the female reproductive tract by providing anchor-like mechanical support and facilitating interactions between spermatozoa and the female reproductive tract in the house mouse.