Metagenomics: Social behavior and the microbiome
Why did social interactions evolve? Complex rules govern how we interact when we meet, and while these principles are often bewildering, they are also somehow intrinsic to the fabric of any human society. Now, in eLife, Elizabeth Archie, Jenny Tung and co-workers have provided compelling evidence to support the theory that physical interaction evolved in vertebrates to help share potentially health-promoting bacteria (Tung et al., 2015).
Tung et al. sequenced the genomes of the various microbes found in the faeces of 48 baboons from two different social groups in Amboseli in Kenya. They found that these ‘microbiomes’ differed between the two social groups, despite them living in overlapping areas and eating similar foods. It has been shown that diet influences the composition and structure of the microbial communities living in the human gut (David et al., 2013). So why were the microbiomes of these groups of baboons not more similar?
The answer lies in the fact that baboons interact by grooming each other (Figure 1). Baboons that groomed each other more frequently had more similar microbiomes, which suggests that physical interactions with others are very important in shaping the microbial communities in these individuals. Baboons don't tend to groom baboons from different social groups (much like humans), so social activities within a group act to consolidate shared communities of microbes.
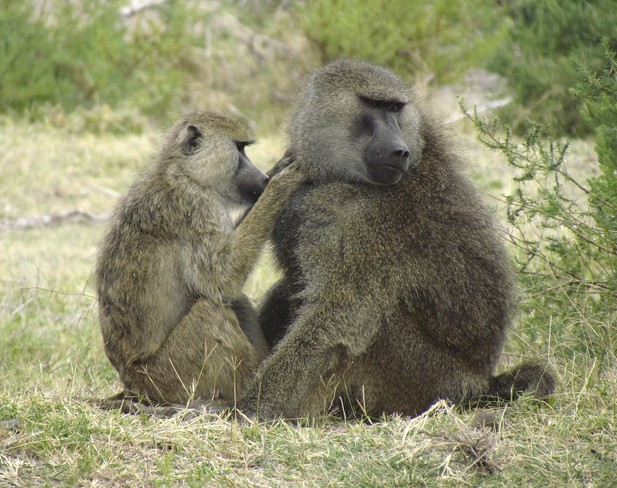
Baboons that groom each other more often have more similar gut microbiomes.
Image credit: Elizabeth A. Miller.
The need for physical interaction or the presence of others is central to human cognitive psychology. Embodied cognition is the study of how the presence of others affects thoughts, feelings, and behaviors (Meier et al., 2012); for example, we tend to distance ourselves physically from those we don't like or trust, whereas we tend to be more physically close and have more physical interactions with our friends and family.
It is highly probable that evolution has shaped social behavior. Physical interactions with those we love feels good; hugs, kisses, and even basic skin-to-skin contact have positive influences on our thoughts and feelings. The hand-shake—which is the standard mode of introduction in many societies—represents this concept. But why did these interactions evolve? Are they purely social constructs, or is physical interaction instinctive, driven by some selective advantage? Sharing microbes in this way can be beneficial: for example, bumblebees living in the same hive share bacteria through fecal pellets (Koch and Schmid-Hempel, 2011). This protects the bees from the virulent parasite Crithidia bombi, and therefore gives these bees a selective advantage over other bees that don't share bacteria.
Acquiring a beneficial microbiome may actually have shaped the evolution of the immune system, whose primary role is to maintain the balance of ‘good’ and ‘bad’ microbes in the microbiome. The immune system aims to control the exposure of host tissues to the microbiome, which it does in part by directly interacting with the bacteria. For example, the protein immunoglobulin A is released into the gut, where it binds to certain species of bacteria: this reduces the ability of the bacteria to move and keeps them away from the cells of the intestine (Hooper et al., 2012). It is likely that this mechanism is also used to capture certain beneficial microbes and retain them in the gut.
Sharing of microbes through physical interaction has been shown to happen in several vertebrate species. For example, my own work in humans demonstrates that family relationships and co-housing can influence the similarity of the microbiomes of individuals (Lax et al., 2014). In fact, in one of the groups examined, a young couple was shown to have much more similar microbiomes on their skin and in their nose than a lodger living with them in their home: however, the lodger had more microbes in common with the couple than he did with anyone else in the study.
Unlike the baboons—who only share significant amounts of microbes due to physical grooming activity—humans can also share microbes through the artificial environment we have constructed for ourselves. Over the last 100 years or so, these indoor environments have become increasingly isolated from the natural world outside. The microbiome of individuals living in the same indoor space can be shared through the air and via surfaces because humans are the main source of the microbes, and therefore most of the microbes in the space are readily able to colonize the human occupants.
This sharing of microbes might seem like a good idea; it worked for the bees. However, there is now mounting evidence to suggest that the over-sharing of the microbiome may be reducing our exposure to richer microbiomes from other sources, thereby limiting the development of our immune system (Lax et al., 2015). Wild baboons are exposed to many different sources of microbes in their environment, and—while their physical interactions may indeed help to share beneficial bacteria—these other microbes are also likely to support their physical, immunological and neurological development.
One of my colleagues, the eminent microbiologist and microbial ecologist Norman Pace, says that his exploration of the microbial world has made him reluctant to share other people's microbiomes (Personal Communication). In fact, Norman now only ‘fist bumps’ when he meets other people, and never shakes their hands. Is this reduced microbial exposure potentially detrimental to his health? Or could it be that in our modern world, Norman has the right idea?
References
-
Socially transmitted gut microbiota protect bumble bees against an intestinal parasiteProceedings of the National Academy of Sciences of USA 108:19288–19292.https://doi.org/10.1073/pnas.1110474108
-
Our interface with the built environment: immunity and the indoor microbiotaTrends in Immunology 36:121–123.https://doi.org/10.1016/j.it.2015.01.001
-
Embodiment in social psychologyTopics in Cognitive Science 4:705–716.https://doi.org/10.1111/j.1756-8765.2012.01212.x
Article and author information
Author details
Publication history
Copyright
© 2015, Gilbert
This article is distributed under the terms of the Creative Commons Attribution License, which permits unrestricted use and redistribution provided that the original author and source are credited.
Metrics
-
- 2,933
- views
-
- 387
- downloads
-
- 19
- citations
Views, downloads and citations are aggregated across all versions of this paper published by eLife.
Download links
Downloads (link to download the article as PDF)
Open citations (links to open the citations from this article in various online reference manager services)
Cite this article (links to download the citations from this article in formats compatible with various reference manager tools)
Further reading
-
- Developmental Biology
- Ecology
Organisms require dietary macronutrients in specific ratios to maximize performance, and variation in macronutrient requirements plays a central role in niche determination. Although it is well recognized that development and body size can have strong and predictable effects on many aspects of organismal function, we lack a predictive understanding of ontogenetic or scaling effects on macronutrient intake. We determined protein and carbohydrate intake throughout development on lab populations of locusts and compared to late instars of field populations. Self-selected protein:carbohydrate targets declined dramatically through ontogeny, due primarily to declines in mass-specific protein consumption rates which were highly correlated with declines in specific growth rates. Lab results for protein consumption rates partly matched results from field-collected locusts. However, field locusts consumed nearly double the carbohydrate, likely due to higher activity and metabolic rates. Combining our results with the available data for animals, both across species and during ontogeny, protein consumption scaled predictably and hypometrically, demonstrating a new scaling rule key for understanding nutritional ecology.
-
- Ecology
Advances in tracking technologies have revealed the diverse migration patterns of birds, which are critical for range mapping and population estimation. Population trends are usually estimated in breeding ranges where birds remain stationary, but for species that breed in remote areas like the Arctic, these trends are often assessed in over-wintering ranges. Assessing population trends during the wintering season is challenging due to the extensive movements of birds in these ranges, which requires a deep understanding of the movement dynamics. However, these movements remain understudied, particularly in the mid-latitudes, where many Arctic breeders overwinter, increasing uncertainty in their ranges and numbers. Here, we show that the Arctic breeding raptor Rough-legged buzzard, which overwinters in the mid-latitudes, has a specific wintering strategy. After migrating ca. 1500 km from the Arctic to mid-latitudes, the birds continue to move throughout the entire over-wintering period, traveling another 1000 km southwest and then back northeast as the snowline advances. This continuous movement makes their wintering range dynamic throughout the season. In essence, this movement represents an extension of the quick migration process, albeit at a slower pace, and we have termed this migration pattern ‘foxtrot migration’, drawing an analogy to the alternating fast and slow movements of the foxtrot dance. These results highlight the potential errors in range mapping from single mid-winter surveys and emphasize the importance of this migration pattern in assessing the conservation status of bird species. Understanding this migration pattern could help to correctly estimate bird populations in over-wintering ranges, which is especially important for species that nest in hard-to-reach regions such as the Arctic.