Cancer Metabolism: Partners in the Warburg effect
In 1918 Albert Einstein convinced Otto Warburg to leave the German infantry and fulfill his patriotic requirements in the first World War by performing research instead (Koppenol et al., 2011). Back in the lab at the Kaiser Wilhelm Institute, Warburg discovered that thin slices of tumors produced lactate much more rapidly than normal tissue. This rapid fermentation of glucose by tumors, even in the presence of ample oxygen, was the first biochemical trait assigned to cancer and is known as the Warburg effect.
When oxygen is present, most human cells rely on a process called oxidative phosphorylation inside mitochondria to convert lactate into carbon dioxide and usable energy. Warburg proposed that the rapid glucose fermentation and associated lactate secretion by the cancer cells was due to mitochondrial dysfunction. However, subsequent studies have shown that most cancer cells do have working mitochondria and, moreover, depend heavily upon them to produce energy (Zu and Guppy, 2004; Moreno-Sánchez et al., 2007). Instead of causing mitochondrial dysfunction, it was found that the mutations that cause cancer also promote the breakdown of glucose in a process called glycolysis. The most striking example involves the PI3K-Akt signaling pathway, which both transduces the signal from the hormone insulin to drive glucose uptake, and is one of the most frequently mutated pathways in cancer. One way this pathway can be activated is by the loss of a tumor suppressing enzyme called PTEN (Shaw and Cantley, 2006). The observation of oncogene-driven glucose uptake seemed to neatly explain the Warburg effect.
Over the past few decades, evidence has steadily accumulated that cancer cells also hijack surrounding cells (Cirri and Chiarugi, 2012). For example, cancer cells secrete growth factors to promote the formation of new blood vessels (Orimo et al., 2005), which are required to supply tumors with nutrients. Moreover, they co-opt surrounding connective tissue cells, including fibroblasts, which exchange signals with the cancer cells in a manner that ultimately drives tumor growth and likely helps to suppress immune responses to the tumor (Cirri and Chiarugi, 2012). However, both the mechanism of this exchange and its role in tumor growth remain poorly understood.
Fibroblasts may exchange both signaling molecules and metabolic fuels with the cancer cells, either by secreting individual molecules (e.g. lactate; Martinez-Outschoorn et al., 2014) or by releasing membrane-bound vesicles known as exosomes (Castellana et al., 2009). For example, recent work has shown that the spread of cancer in the brain is promoted by the exosomes that are released by a particular type of brain cell. These exosomes contain small RNA molecules known as microRNAs that can silence the gene that encodes the PTEN enzyme, whose loss drives an increase in glycolysis (Zhang et al., 2015).
Now, in eLife, Deepak Nagrath at Rice University and colleagues – including Hongyun Zhao as first author – show that cancer-associated fibroblasts release exosomes that both deliver nutrients to cancer cells and inhibit oxidative phosphorylation (Zhao et al., 2016; Figure 1). Zhao et al. use isotope-labelled carbon compounds to provide compelling evidence that exosomes from fibroblasts can supply an amino acid called glutamine and other nutrients to cancer cells. A shortage of glutamine can limit the growth of pancreatic and perhaps other cancers (Kamphorst et al., 2015). Importantly, although the exosomes contribute modest amounts of nutrients, they can protect cancer cells from starvation, hinting at one potential role for such metabolic exchange in tumors.
More striking and surprising is the role of the exosomes in causing the Warburg effect. Adding exosomes to prostate or pancreatic cancer cells both promotes glycolysis and blocks oxidative metabolism. It is likely that the increase in glycolysis is caused by the reduction in oxidative phosphorylation so, in this respect, the exosomes trigger glycolysis in the way initially envisioned by Warburg. These results call for a re-examination of the contributions of both processes to energy generation in cancer cells that are still associated with their neighbors.
Such re-examination is particularly important given that oxidative phosphorylation is reduced so dramatically in cancer cells, with oxygen consumption lowered by up to 80% within 24 hours of receiving exosomes from fibroblasts. Zhao et al. – who are based at Rice University, Baylor College of Medicine, the University of Texas MD Anderson Cancer Center and Stanford University – propose that the exosomes may deliver microRNAs that silence oxidative metabolism genes, but this is hard to reconcile with the timing. Since the proteins involved in oxidative phosphorylation are generally long-lived, even complete inhibition of their production seems unlikely to produce such drastic effects so quickly. Nor can the decreased oxidative phosphorylation be explained by the delivery of nutrients by exosomes, because increasing the access to such nutrients would be expected to promote, not inhibit, the use of oxygen. Thus, understanding how the exosomes inhibit oxidative phosphorylation is a key challenge going forward. Such work holds the potential to illuminate not only the Warburg effect, but also the regulation of oxidative phosphorylation in cells more generally.
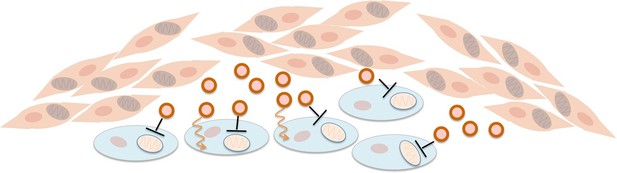
Fibroblasts supply nutrients to cancer cells and inhibit oxidative phosphorylation in cancer cells.
Fibroblasts (pink cells) associate with epithelial cancer cells (blue cells) and release exosomes (circles) that transfer nutrients to epithelial cancer cells (orange lines). In addition, they inhibit mitochondrial oxidative phosphorylation in the cancer cells (black blunt arrows), perhaps via microRNAs that silence particular genes.
References
-
Cancer-associated-fibroblasts and tumour cells: a diabolic liaison driving cancer progressionCancer Metastasis Reviews 31:195–208.https://doi.org/10.1007/s10555-011-9340-x
-
Otto Warburg's contributions to current concepts of cancer metabolismNature Reviews Cancer 11:325–337.https://doi.org/10.1038/nrc3038
-
Energy metabolism in tumor cellsThe FEBS Journal 274:1393–1418.https://doi.org/10.1111/j.1742-4658.2007.05686.x
-
Cancer metabolism: Facts, fantasy, and fictionBiochemical and Biophysical Research Communications 313:459–465.https://doi.org/10.1016/j.bbrc.2003.11.136
Article and author information
Author details
Publication history
Copyright
© 2016, Rabinowitz et al.
This article is distributed under the terms of the Creative Commons Attribution License, which permits unrestricted use and redistribution provided that the original author and source are credited.
Metrics
-
- 4,662
- views
-
- 826
- downloads
-
- 10
- citations
Views, downloads and citations are aggregated across all versions of this paper published by eLife.
Download links
Downloads (link to download the article as PDF)
Open citations (links to open the citations from this article in various online reference manager services)
Cite this article (links to download the citations from this article in formats compatible with various reference manager tools)
Further reading
-
- Cell Biology
The primary cilium is a microtubule-based organelle that cycles through assembly and disassembly. In many cell types, formation of the cilium is initiated by recruitment of preciliary vesicles to the distal appendage of the mother centriole. However, the distal appendage mechanism that directly captures preciliary vesicles is yet to be identified. In an accompanying paper, we show that the distal appendage protein, CEP89, is important for the preciliary vesicle recruitment, but not for other steps of cilium formation (Kanie et al., 2025). The lack of a membrane-binding motif in CEP89 suggests that it may indirectly recruit preciliary vesicles via another binding partner. Here, we identify Neuronal Calcium Sensor-1 (NCS1) as a stoichiometric interactor of CEP89. NCS1 localizes to the position between CEP89 and the centriole-associated vesicle marker, RAB34, at the distal appendage. This localization was completely abolished in CEP89 knockouts, suggesting that CEP89 recruits NCS1 to the distal appendage. Similar to CEP89 knockouts, preciliary vesicle recruitment as well as subsequent cilium formation was perturbed in NCS1 knockout cells. The ability of NCS1 to recruit the preciliary vesicle is dependent on its myristoylation motif and NCS1 knockout cells expressing a myristoylation defective mutant failed to rescue the vesicle recruitment defect despite localizing properly to the centriole. In sum, our analysis reveals the first known mechanism for how the distal appendage recruits the preciliary vesicles.
-
- Cell Biology
Distal appendages are ninefold symmetric blade-like structures attached to the distal end of the mother centriole. These structures are critical for the formation of the primary cilium, by regulating at least four critical steps: preciliary vesicle recruitment, recruitment and initiation of intraflagellar transport (IFT), and removal of CP110. While specific proteins that localize to the distal appendages have been identified, how exactly each protein functions to achieve the multiple roles of the distal appendages is poorly understood. Here, we comprehensively analyze known and newly discovered distal appendage proteins (CEP83, SCLT1, CEP164, TTBK2, FBF1, CEP89, KIZ, ANKRD26, PIDD1, LRRC45, NCS1, CEP15) for their precise localization, order of recruitment, and their roles in each step of cilia formation. Using CRISPR-Cas9 knockouts, we show that the order of the recruitment of the distal appendage proteins is highly interconnected and a more complex hierarchy. Our analysis highlights two protein modules, CEP83-SCLT1 and CEP164-TTBK2, as critical for structural assembly of distal appendages. Functional assays revealed that CEP89 selectively functions in the RAB34+ vesicle recruitment, while deletion of the integral components, CEP83-SCLT1-CEP164-TTBK2, severely compromised all four steps of cilium formation. Collectively, our analyses provide a more comprehensive view of the organization and the function of the distal appendage, paving the way for molecular understanding of ciliary assembly.