Neuroscience: Endocytosis gets in tune with action potential bursts
The arrival of an action potential at a synapse connecting two neurons causes calcium channels in the presynaptic membrane to open, and the resulting influx of calcium triggers a sequence of events that ends with the regeneration of the action potential in the postsynaptic cell. First, vesicles filled with neurotransmitter fuse with the membrane of the presynaptic neuron and release their contents into the synapse. These neurotransmitter molecules can then bind to receptors on the postsynaptic cell, enabling the action potential to be regenerated. However, the presynaptic neuron must also recycle those vesicles that have been released to ensure that the whole process can be repeated when the next action potential arrives.
It is tempting to hypothesize that the release of the vesicles (a process known as exocytosis) and their recycling (endocytosis) are initiated or controlled by common signalling mechanisms. It is known that exocytosis is triggered by an influx of calcium ions, but the relationship between calcium ions and endocytosis is somewhat tenuous (for review, see Yamashita, 2012). Now in eLife, Tim Ryan, Pietro De Camilli, and co-workers—including Moritz Armbruster as first author—report that calcium entry during bursts of action potentials has an important role in optimizing the rate of endocytosis (Armbruster et al., 2013).
Previous studies have found that an increase in calcium influx has a variety of effects on the rate of endocytosis, ranging from inhibition (von Gersdorff and Mathews, 1994) to acceleration (Sankaranarayanan and Ryan, 2001; Wu et al., 2009). Armbruster and co-workers—who are at Cornell University and Yale University—have now reconciled these results by carefully examining how the rate of endocytosis varies as a function of the number of action potentials delivered at a given frequency, referred to as a burst. They revealed that endocytosis slows down as the number of action potentials increases from 25 to 100, and that this deceleration requires calcium. This is in contrast to previous work, which found the rate of endocytosis to be constant over this range (Balaji et al., 2008). However, Armbruster et al. note that this previous study compared the ensemble average rate between boutons, and may thus have been less sensitive than their own study, which examined the rate of endocytosis within a bouton following differing numbers of action potentials.
Perhaps the most surprising finding came when Armbruster et al. examined the effect of a single action potential on the rate of endocytosis. Given that endocytosis slows as the number of action potentials increases from 25 to 100, they expected it to occur rapidly in response to a single action potential. On the contrary, they found that endocytosis was slow after a single spike, and accelerated as the number of action potentials increased to 25. It seems, therefore, that the rate of endocytosis shows a biphasic relation to the number of action potentials, with the fastest rate corresponding to an intermediate stimulation range (Figure 1A).
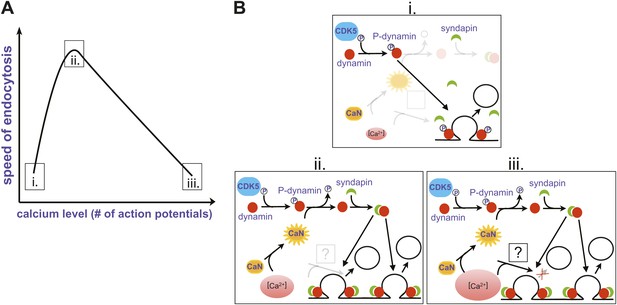
Changes in the speed of endocytosis and the underlying signalling pathways. (A) Schematic representation of the relationship between the speed of endocytosis, the level of intracellular calcium produced by increasing numbers of action potentials, and the signalling pathways activated by intracellular calcium when there is just a single action potential (i), a small burst of action potentials (ii), and a large burst of action potentials (iii). (B) Calcium levels are low when there is just one action potential (i), so calcineurin (CaN) is not activated, allowing Cdk5 to maintain high levels of phosphorylation of dynamin (P-dynamin). As the number of action potentials increases (ii), there are more calcium ions to activate the calcineurin, which results in a shift towards dephosphorylated dynamin. This can then bind to syndapin, and this interaction increases the rate of endocytosis. However, as the number of action potentials, and hence calcium levels, increase further (iii), a second calcium-dependent pathway with unknown molecular components is activated, and this reduces the rate of endocytosis.
The next question was obvious: what is the mechanism behind this modulation of endocytosis by the number of action potentials? Given that the process clearly involved calcium, Armbruster et al. turned to proteins involved in endocytosis that are also substrates for calcineurin, an enzyme activated by calcium ions. This enzyme, which removes phosphate groups, and its counterplayer Cdk5 (which adds phosphate groups) have been implicated in the dynamic regulation of synaptic strength (Kim and Ryan, 2013).
One of the targets of calcineurin/Cdk5 is dynamin, a small GTPase enzyme that helps newly formed vesicles to bud off from the presynaptic membrane during endocytosis (Ferguson and De Camilli, 2012). In an elegant set of rescue experiments, Armbruster et al. showed that the acceleration of endocytosis can only occur if phosphate groups have been removed from two previously identified sites on dynamin. Since dephosphorylated dynamin interacts with another protein called syndapin, which is also involved in endocytosis, they propose the following signalling pathway: The firing of multiple action potentials leads to a brief elevation of presynaptic calcium (Figure 1B (i)); this calcium activates calcineurin, which then dephosphorylates dynamin at two sites (ii); and a dynamin-syndapin complex then accelerates endocytosis (iii). Exactly how this last step occurs is unclear, although one possibility is that syndapin accelerates the recruitment of dynamin to sites of endocytosis (Koch et al., 2011).
Intriguingly, previous studies suggest that stimulus-induced dephosphorylation of dynamin lasts approximately 40 s (Robinson et al., 1994), which is also roughly the length of time for which endocytosis acceleration persisted after a small burst (Armbruster et al., 2013). This is the strongest piece of evidence that calcium regulates the speed of endocytosis by modulating the balance of calcineurin/Cdk5 activity. Whether dynamin is the only target of this pathway remains to be determined.
The mechanism responsible for the slowing of endocytosis with larger numbers of action potentials remains unclear (Figure 1), but it is not related to phosphorylation of dynamin at the syndapin interaction site. This suggests that the recycling of vesicles might depend on at least two separate calcium-dependent processes. One possibility is that endocytosis slows as the number of action potentials increases because the accumulation of exocytic proteins on the surface of the presynaptic cell leads to saturation of endocytic machinery, as previously described (Balaji et al., 2008). Moreover, because endocytosis still occurred in experiments where the dynamin phosphorylation state could not be altered, this pathway is likely not responsible for the calcium-dependent initiation of endocytosis (Gad et al., 1998).
Another intriguing aspect of these results is the potential for cell-type specific modulation. The acceleration of endocytosis with increasing action potential number in the small burst range could be regulated by a cell’s intrinsic calcium buffering capacity or its calcineurin/Cdk5 activity balance. Consistent with this, Armbruster et al. observed differences in the degree of regulation between cultures of neurons from different species and brain regions. Additionally, given that intrinsic firing patterns vary between cell types in vivo, it is possible that the standard burst size of a cell may define its baseline endocytosis rate. In conclusion, these findings add endocytosis to the list of dynamically regulated processes within synaptic transmission, which enable synapses to optimize information transfer between neurons in order to form networks within the brain.
References
-
Dynamin, a membrane-remodelling GTPaseNat Rev Mol Cell Biol 13:75–88.https://doi.org/10.1038/nrm3266
-
Phosphorylation of dynamin I and synaptic-vesicle recyclingTrends Neurosci 17:348–353.https://doi.org/10.1016/0166-2236(94)90179-1
Article and author information
Author details
Publication history
Copyright
© 2013, Herman and Rosenmund
This article is distributed under the terms of the Creative Commons Attribution License, which permits unrestricted use and redistribution provided that the original author and source are credited.
Metrics
-
- 512
- views
-
- 48
- downloads
-
- 1
- citations
Views, downloads and citations are aggregated across all versions of this paper published by eLife.
Download links
Downloads (link to download the article as PDF)
Open citations (links to open the citations from this article in various online reference manager services)
Cite this article (links to download the citations from this article in formats compatible with various reference manager tools)
Further reading
-
- Cell Biology
Aggregation of mutant forms of Huntingtin is the underlying feature of neurodegeneration observed in Huntington’s disorder. In addition to neurons, cellular processes in non-neuronal cell types are also shown to be affected. Cells expressing neurodegeneration–associated mutant proteins show altered uptake of ligands, suggestive of impaired endocytosis, in a manner as yet unknown. Using live cell imaging, we show that clathrin-mediated endocytosis (CME) is affected in Drosophila hemocytes and mammalian cells containing Huntingtin aggregates. This is also accompanied by alterations in the organization of the actin cytoskeleton resulting in increased cellular stiffness. Further, we find that Huntingtin aggregates sequester actin and actin-modifying proteins. Overexpression of Hip1 or Arp3 (actin-interacting proteins) could restore CME and cellular stiffness in cells containing Huntingtin aggregates. Neurodegeneration driven by pathogenic Huntingtin was also rescued upon overexpression of either Hip1 or Arp3 in Drosophila. Examination of other pathogenic aggregates revealed that TDP-43 also displayed defective CME, altered actin organization and increased stiffness, similar to pathogenic Huntingtin. Together, our results point to an intimate connection between dysfunctional CME, actin misorganization and increased cellular stiffness caused by alteration in the local intracellular environment by pathogenic aggregates.
-
- Cell Biology
- Physics of Living Systems
The endoplasmic reticulum (ER), the largest cellular compartment, harbours the machinery for the biogenesis of secretory proteins and lipids, calcium storage/mobilisation, and detoxification. It is shaped as layered membranous sheets interconnected with a network of tubules extending throughout the cell. Understanding the influence of the ER morphology dynamics on molecular transport may offer clues to rationalising neuro-pathologies caused by ER morphogen mutations. It remains unclear, however, how the ER facilitates its intra-luminal mobility and homogenises its content. It has been recently proposed that intra-luminal transport may be enabled by active contractions of ER tubules. To surmount the barriers to empirical studies of the minuscule spatial and temporal scales relevant to ER nanofluidics, here we exploit the principles of viscous fluid dynamics to generate a theoretical physical model emulating in silico the content motion in actively contracting nanoscopic tubular networks. The computational model reveals the luminal particle speeds, and their impact in facilitating active transport, of the active contractile behaviour of the different ER components along various time–space parameters. The results of the model indicate that reproducing transport with velocities similar to those reported experimentally in single-particle tracking would require unrealistically high values of tubule contraction site length and rate. Considering further nanofluidic scenarios, we show that width contractions of the ER’s flat domains (perinuclear sheets) generate local flows with only a short-range effect on luminal transport. Only contractions of peripheral sheets can reproduce experimental measurements, provided they are able to contract fast enough.