Mitosis: Checkpoint proteins come under scrutiny
Before a cell can divide it must make a copy of all its chromosomes. After this has happened, each pair of identical chromosomes, which are known as sister chromatids, must be pulled apart, with one sister chromatid going into each daughter cell. Defective segregation of sister chromatids in human cells can lead to miscarriages, birth defects and diseases involving the proliferation of cells, such as cancer, so the process of chromosome segregation is rigorously controlled.
To ensure that sister chromatids are separated properly, a process known as chromosome bi-orientation must take place. This involves multi-protein structures called kinetochores, which form at the centre of each chromosome, another structure called the spindle, which has poles at opposite ends of the cell, and tubular polymers called microtubules that connect the kinetochores to the poles. Each sister chromatid has its own kinetochore, and chromosome bi-orientation involves one sister chromatid being connected to one pole, and the other sister chromatid being connected to the other pole. Once this has been achieved an enzyme called the APC/C is activated. This triggers removal of the glue that hold the sister chromatids together and the process of cell division can continue.
In addition to providing the physical linkage between the chromosomes and the microtubules, the kinetochore acts as the platform for a surveillance system called the spindle assembly checkpoint (SAC), which ensures that the APC/C is not activated until all chromosomes are correctly bi-oriented. The components of the SAC—which include various proteins (Mad1, Mad2, Mad3/BubR1 and Bub3) and kinases (Bub1 and Mps1)—are recruited to the kinetochores once the SAC has been activated. In particular, the recruitment of a Mad1-Mad2 complex is thought to herald the start of a series of events that ensures that APC/C is not activated (Sironi et al., 2002).
Only when all chromatids are correctly bi-oriented is the SAC switched off or ‘silenced’. However, it is still not clear what the SAC actually monitors. Several lines of evidence suggest that full occupancy of kinetochores by microtubules is required to silence the SAC (the attachment model), whereas other evidence suggests that intra-kinetochore stretch is also critically important (the tension model; Maresca and Salmon, 2009; Uchida et al., 2009). However, there is no detailed biochemical evidence to support either of these models. One of the reasons for this is that although the components of the spindle checkpoint were identified more than 20 years ago in yeast, their binding site (or sites) at kinetochores have, until recently, remained elusive. Now, in eLife, Andreas Musacchio and colleagues—including Ivana Primorac as first author—reveal the structural basis by which the Bub1 and Bub3 checkpoint proteins interact with the kinetochore (Primorac et al., 2013).
Important initial work by Mitsuhiro Yanagida and co-workers at Kyoto University pinpointed the KNL1 kinetochore protein as a likely receptor for the Bub1 and Mad3/BubR1 checkpoint proteins (Kiyomitsu et al., 2007). In particular they and others showed that the N-terminus of human KNL1 contains two KI motifs that bind Bub1 and Mad3/BubR1 (Figure 1). Crystal structures of these interactions have been reported (Bolanos-Garcia et al., 2012; Krenn et al., 2012).
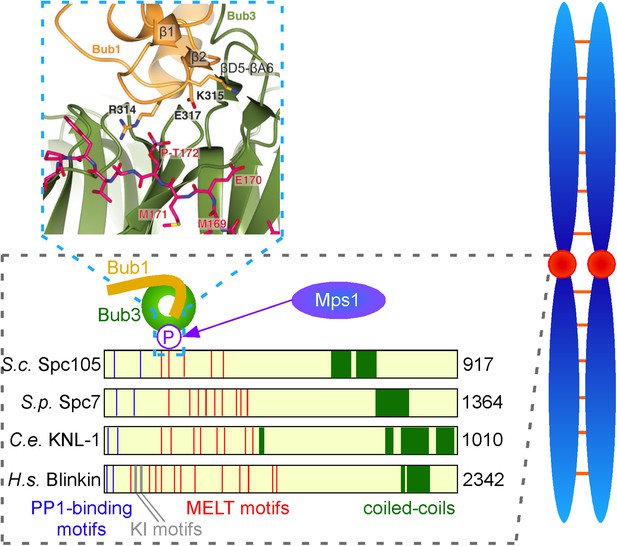
Interactions between checkpoint proteins the kinetochore. Before pairs of sister chromatids (shown in blue on the right) can be pulled apart during cell division, structures called spindle assembly checkpoints (SACs) form on the kinetochores (red circles) of each sister chromatid. The domain architecture of an important kinetochore protein called KNL1 is shown for four species, together with the name of the protein in that species and the number of amino acids it contains: S. cerevisiae (budding yeast, top); S. pombe (fission yeast); C. elegans (worm); Human (bottom). In experiments on budding yeast Primorac et al. have shown that the checkpoint protein Bub3 (green) binds to MELT motifs (red) that have been phosphorylated (P) by the enzyme Mps1, and that the checkpoint protein Bub1 (gold) then binds to Bub3 (and lies in almost the same plane as Bub3). A portion of the crystal structure displaying the interaction between Bub3, Bub1 and the phosphorylated MELT peptide (magenta) is also shown. The human version of KNL1 is the only version to have KI motifs (grey, see text); PP1-binding sites (blue) and coiled-coil kinetochore-binding domains (dark green) are also shown.
However, subsequent studies by the Musacchio lab showed these KI motifs are not essential for the association of Bub1 and Mad3/BubR1 to kinetochores (Krenn et al., 2012). Moreover, these motifs are absent from the homologues of KNL1 in yeast, so they may be less important in the recruitment of checkpoint proteins than was previous thought. More recently, several other groups, working principally with yeast, found that the KNL1 family of proteins contain a variable number of so-called MELT motifs (where M, E, L and T are all amino acids), and that these motifs, when phosphorylated by a kinase called Mps1, provide a binding site for the Bub3 and Bub1 proteins (London et al., 2012; Shepperd et al., 2012; Yamagishi et al., 2012).
Now, Musacchio and colleagues—who are based at the Max Planck Institute of Molecular Physiology in Dortmund, the IFOM laboratory in Milan and the University of Duisburg-Essen—show that a phosphorylated MELT peptide derived from budding yeast interacts with two ‘blades’ of the β-propeller in Bub3 (Figure 1). They go on to show that mutation of two basic residues in Bub3, which co-ordinate the phosphorylated threonine (T) residue of the MELT peptide, abolishes the interaction between the Bub3-Bub1 complex and the kinetochore, and therefore compromises checkpoint signalling. By demonstrating that Bub3 is the critical element that tethers Bub1 and Mad3/BubR1 to the kinetochore, Primorac et al. confirm an idea first put forward by Steven Taylor and colleagues over a decade ago (Taylor et al., 1998). Notably, however, the crystal structure suggests additional residues in the N-terminus of Bub1 contribute to this interaction (Figure 1). Although Bub3 also binds Mad3/BubR1, these additional stabilising residues are absent in the Mad3/BubR1 protein, suggesting that Mad3/BubR1 binds indirectly to KNL1 through an interaction with Bub1, rather than directly to the phosphorylated MELT motifs of KNL1.
Understanding the mechanical and biochemical basis of SAC signalling is one of the most challenging problems in cell biology. Although Primorac et al. establish the structural basis for the interaction of the Bub3-Bub1 complex with the kinetochore, many issues remain unresolved. First, it is not clear whether the MELT motifs are dephosphorylated once the spindle checkpoint is silenced, or which phosphatase catalyses this reaction, or whether dephosphorylation of these residues is important for the silencing process.
Second, structural data suggest that each MELT motif has the potential to bind one Bub3-Bub1 heterodimer. This raises the question as to why KNL1 and its homologues contain multiple MELT motifs (Figure 1)? One exciting possibility is that the arrays of MELT motifs in KNL1 act as a quantitative sensor of intra-kinetochore stretch. If this is the case, are unattached kinetochores monitored by the same, or a different, mechanism?
Third, it is not clear what role the interaction of Bub3-Bub1 with KNL1 plays in spindle checkpoint signalling in other organisms. For instance, the Bub3 protein is required for spindle checkpoint silencing in fission yeast, but activation can happen without it (Vanoosthuyse et al., 2009). Lastly, we still know very little about the interactions between other spindle checkpoint proteins—notably Mps1, Mad1 and Mad2—and the kinetochore.
Although the process of mitosis has been studied by scientists for over 100 years, the work of Primorac et al. suggests we can, nevertheless, be confident that full enlightenment will eventually emerge through the combined application of sophisticated molecular genetics, quantitative high-resolution live-cell imaging in various model systems, structural analysis of complexes and interaction surfaces, and biophysical analysis of the reconstituted system. But we may have to be patient for a little while longer.
References
-
Bub3p facilitates spindle checkpoint silencing in fission yeastMol Biol Cell 20:5096–5105.https://doi.org/10.1091/mbc.E09-09-0762
Article and author information
Author details
Publication history
Copyright
© 2013, Mora-Santos and Millar
This article is distributed under the terms of the Creative Commons Attribution License, which permits unrestricted use and redistribution provided that the original author and source are credited.
Metrics
-
- 376
- views
-
- 41
- downloads
-
- 0
- citations
Views, downloads and citations are aggregated across all versions of this paper published by eLife.
Download links
Downloads (link to download the article as PDF)
Open citations (links to open the citations from this article in various online reference manager services)
Cite this article (links to download the citations from this article in formats compatible with various reference manager tools)
Further reading
-
- Structural Biology and Molecular Biophysics
Cyclic nucleotide-binding domain (CNBD) ion channels play crucial roles in cellular-signaling and excitability and are regulated by the direct binding of cyclic adenosine- or guanosine-monophosphate (cAMP, cGMP). However, the precise allosteric mechanism governing channel activation upon ligand binding, particularly the energetic changes within domains, remains poorly understood. The prokaryotic CNBD channel SthK offers a valuable model for investigating this allosteric mechanism. In this study, we investigated the conformational dynamics and energetics of the SthK C-terminal region using a combination of steady-state and time-resolved transition metal ion Förster resonance energy transfer (tmFRET) experiments. We engineered donor-acceptor pairs at specific sites within a SthK C-terminal fragment by incorporating a fluorescent noncanonical amino acid donor and metal ion acceptors. Measuring tmFRET with fluorescence lifetimes, we determined intramolecular distance distributions in the absence and presence of cAMP or cGMP. The probability distributions between conformational states without and with ligand were used to calculate the changes in free energy (ΔG) and differences in free energy change (ΔΔG) in the context of a simple four-state model. Our findings reveal that cAMP binding produces large structural changes, with a very favorable ΔΔG. In contrast to cAMP, cGMP behaved as a partial agonist and only weakly promoted the active state. Furthermore, we assessed the impact of protein oligomerization and ionic strength on the structure and energetics of the conformational states. This study demonstrates the effectiveness of time-resolved tmFRET in determining the conformational states and the ligand-dependent energetics of the SthK C-terminal region.
-
- Structural Biology and Molecular Biophysics
Ciliary rootlets are striated bundles of filaments that connect the base of cilia to internal cellular structures. Rootlets are critical for the sensory and motile functions of cilia. However, the mechanisms underlying these functions remain unknown, in part due to a lack of structural information of rootlet organization. In this study, we obtain 3D reconstructions of membrane-associated and purified rootlets from mouse retina using cryo-electron tomography. We show that flexible protrusions on the rootlet surface, which emanate from the cross-striations, connect to intracellular membranes. In purified rootlets, the striations were classified into amorphous (A)-bands, associated with accumulations on the rootlet surface, and discrete (D)-bands corresponding to punctate lines of density that run through the rootlet. These striations connect a flexible network of longitudinal filaments. Subtomogram averaging suggests the filaments consist of two intertwined coiled coils. The rootlet’s filamentous architecture, with frequent membrane-connecting cross-striations, lends itself well for anchoring large membranes in the cell.