Breathing: CO2 in the spotlight
We breathe to get oxygen and to get rid of carbon dioxide (CO2). The precise monitoring of CO2 levels in the body is crucial because too much of it makes our blood acidic, which can have toxic effects. Under normal resting conditions, the concentration of CO2 controls our respiratory rate. For example, in most animals, including humans, high levels of CO2 lead to faster breathing. Moreover, having a weakened response to CO2 can be a life-threatening condition (Guyenet et al., 2010).
In spite of many years of research, the identity of the neurons involved in sensing CO2, and thus in controlling breathing, has been controversial (Guyenet et al., 2013). Now, in eLife, Jean-Francois Brunet of the Ecole Normale Supérieure and colleagues from France, Japan and Sweden report that neurons in the retrotrapezoid nucleus in the brainstem make up the CO2 sensor.
The retrotrapezoid nucleus is a tiny structure located in the ventral side of the brainstem—that is, it is towards the front of the brainstem in humans, but on the underside in mice and most other animals (Figure 1). These neurons rhythmically send signals to other neurons at normal body pH, and increase the rate at which they fire when the pH of the blood becomes acidic (Goridis and Brunet, 2010). The neurons in the retrotrapezoid nucleus are also directly connected to the preBötzinger complex (the structure in the brainstem that generates the breathing rhythm) and can adjust its activity. Mouse models in which the development of retrotrapezoid neurons is disrupted display slow breathing and have weakened, or blunted, responses to acidification (Sieber et al., 2007; Dubreuil et al., 2008, 2009; Goridis et al., 2010; Ramanantsoa et al., 2011). However, a recent study reported that retrotrapezoid neurons, particularly in newborn mice, might not be required for CO2 sensitivity (Huang et al., 2012). This cast doubts on the precise role of the retrotrapezoid nucleus.
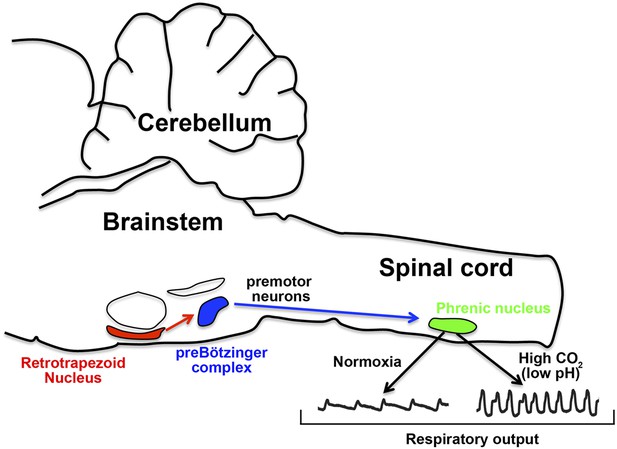
How the nervous system responds to CO2 in the blood.
In mice, the retrotrapezoid nucleus (red) contains about 600 neurons and is directly connected to the preBötzinger complex (blue). Under normal levels of oxygen (normoxia), the activity of the preBötzinger complex controls the firing rate of neurons in the phrenic nucleus (green) by sending signals via premotor neurons. Phrenic neurons control the muscles involved in breathing and therefore control respiratory output. Ruffault et al. report that when levels of CO2 are high (which lowers blood pH), the retrotrapezoid neurons increase their firing rate: this adjusts the activity of preBötzinger complex and increases the firing rate of the phrenic neurons, which in turn increases respiratory output in order to eliminate more CO2.
Retrotrapezoid neurons send signals that activate, or excite, other neurons. Brunet and colleagues—who include Pierre-Louis Ruffault as first author—combined mouse genetics and optogenetic techniques to selectively stimulate specific retrotrapezoid neurons with light (Ruffault et al., 2015). This revealed that retrotrapezoid neurons adjust the activity of the phrenic nerve—the motor nerve that controls the muscles involved in breathing—by activating phrenic motor neurons via the preBötzinger complex (Figure 1). These results provide conclusive evidence of a direct association between the activity of retrotrapezoid neurons and respiratory motor neurons.
Retrotrapezoid neurons develop from progenitor cells located in the dorsal side of the brainstem (that is, towards the back of the brainstem in humans, but on the topside in mice). At first, these progenitor cells express a transcription factor called Phox2b. Later in their development, they start to co-express a transcription factor called Lbx1 and migrate towards the ventral brainstem (Sieber et al., 2007), where they switch on the expression of a third transcription factor called Atoh1. This means that these neurons are defined by the co-expression of these three transcription factors, and mutant mice that lack any of these three fail to develop a retrotrapezoid nucleus.
Ruffault et al. observed a blunted response to CO2 at birth in mutant mice that lack Atoh1, which is similar to the weakened response seen in Phox2b mutants (Dubreuil et al., 2008, 2009; Goridis et al., 2010; Ramanantsoa et al., 2011). In Atoh1 mutants, the neurons that are intended for the retrotrapezoid nucleus are born, but they fail to migrate to the ventral brainstem. And while these incorrectly positioned ‘retrotrapezoid’ neurons remain sensitive to pH, they cannot adjust the activity of the preBötzinger complex.
There are many thousand types of neurons in the brainstem, but only two populations of neurons are known to co-express Phox2b and Atoh1 (which Ruffault et al. call ‘Phox2bon/Atoh1on neurons’). Only one of these populations is found in the retrotrapezoid nucleus, and the other population could be removed without any effect on CO2 sensitivity. Ruffault et al. then explored what happened when they silenced or activated the Phox2bon/Atoh1on neurons within the retrotrapezoid nucleus. In newborn mice, this interfered with normal breathing and the response to pH, which illustrates that these neurons are indeed necessary to sense CO2 and adjust breathing accordingly at birth.
But, this is not the full story. Ruffault et al. found that the response to CO2 partially recovers in maturing animals that lack the retrotrapezoid neurons. This suggests that, later on in life, other regions of the brain are also involved in sensing CO2. However, when CO2 levels increase, animals that lack retrotrapezoid neurons respond by only mildly increasing their breathing rate, and the full response to CO2 is largely impaired (Ruffault et al., 2015).
Congenital breathing disorders in humans are rare but represent an immense burden to the affected individuals and their families (Ramanantsoa and Gallego, 2013). These diseases are life threatening because the afflicted patients breathe slowly, especially during sleep. In spite of their relevance and impact on public health, our current knowledge about these diseases is fragmented, and the only treatment for these conditions is mechanical ventilation of the patients. Mouse genetics allows us to explore the breathing centres in greater detail, and opens avenues to test new drug treatments that are designed to improve breathing. The work of Ruffault et al. represents a stringent study on the function of the retrotrapezoid nucleus, a breathing centre that is associated with congenital respiratory disease, and provides an animal model for future therapeutic research.
References
-
A human mutation in Phox2b causes lack of CO2 chemosensitivity, fatal central apnea, and specific loss of parafacial neuronsProceedings of the National Academy of Sciences of USA 105:1067–1072.https://doi.org/10.1073/pnas.0709115105
-
Central chemoreception: lessons from mouse and human geneticsRespiratory Physiology & Neurobiology 173:312–321.https://doi.org/10.1016/j.resp.2010.03.014
-
Phox2b, congenital central hypoventilation syndrome and the control of respirationSeminars in Cell & Developmental Biology 21:814–822.https://doi.org/10.1016/j.semcdb.2010.07.006
-
Central respiratory chemoreceptionJournal of Comparative Neurology 518:3883–3906.https://doi.org/10.1002/cne.22435
-
Congenital central hypoventilation syndromeRespiratory Physiology & Neurobiology 189:272–279.https://doi.org/10.1016/j.resp.2013.05.018
-
Breathing without CO2 chemosensitivity in conditional Phox2b mutantsJournal of Neuroscience 31:12880–12888.https://doi.org/10.1523/JNEUROSCI.1721-11.2011
Article and author information
Author details
Publication history
Copyright
© 2015, Hernandez-Miranda and Birchmeier
This article is distributed under the terms of the Creative Commons Attribution License, which permits unrestricted use and redistribution provided that the original author and source are credited.
Metrics
-
- 3,589
- views
-
- 167
- downloads
-
- 5
- citations
Views, downloads and citations are aggregated across all versions of this paper published by eLife.
Download links
Downloads (link to download the article as PDF)
Open citations (links to open the citations from this article in various online reference manager services)
Cite this article (links to download the citations from this article in formats compatible with various reference manager tools)
Further reading
-
- Medicine
- Neuroscience
Pain after surgery causes significant suffering. Opioid analgesics cause severe side effects and accidental death. Therefore, there is an urgent need to develop non-opioid therapies for managing post-surgical pain. Local application of Clarix Flo (FLO), a human amniotic membrane (AM) product, attenuated established post-surgical pain hypersensitivity without exhibiting known side effects of opioid use in mice. This effect was achieved through direct inhibition of nociceptive dorsal root ganglion (DRG) neurons via CD44-dependent pathways. We further purified the major matrix component, the heavy chain-hyaluronic acid/pentraxin 3 (HC-HA/PTX3) from human AM that has greater purity and water solubility than FLO. HC-HA/PTX3 replicated FLO-induced neuronal and pain inhibition. Mechanistically, HC-HA/PTX3-induced cytoskeleton rearrangements to inhibit sodium current and high-voltage activated calcium current on nociceptive DRG neurons, suggesting it is a key bioactive component mediating pain relief. Collectively, our findings highlight the potential of naturally derived biologics from human birth tissues as an effective non-opioid treatment for post-surgical pain. Moreover, we unravel the underlying neuronal mechanisms of pain inhibition induced by FLO and HC-HA/PTX3.
-
- Computational and Systems Biology
- Neuroscience
Hypothalamic kisspeptin (Kiss1) neurons are vital for pubertal development and reproduction. Arcuate nucleus Kiss1 (Kiss1ARH) neurons are responsible for the pulsatile release of gonadotropin-releasing hormone (GnRH). In females, the behavior of Kiss1ARH neurons, expressing Kiss1, neurokinin B (NKB), and dynorphin (Dyn), varies throughout the ovarian cycle. Studies indicate that 17β-estradiol (E2) reduces peptide expression but increases Slc17a6 (Vglut2) mRNA and glutamate neurotransmission in these neurons, suggesting a shift from peptidergic to glutamatergic signaling. To investigate this shift, we combined transcriptomics, electrophysiology, and mathematical modeling. Our results demonstrate that E2 treatment upregulates the mRNA expression of voltage-activated calcium channels, elevating the whole-cell calcium current that contributes to high-frequency burst firing. Additionally, E2 treatment decreased the mRNA levels of canonical transient receptor potential (TPRC) 5 and G protein-coupled K+ (GIRK) channels. When Trpc5 channels in Kiss1ARH neurons were deleted using CRISPR/SaCas9, the slow excitatory postsynaptic potential was eliminated. Our data enabled us to formulate a biophysically realistic mathematical model of Kiss1ARH neurons, suggesting that E2 modifies ionic conductances in these neurons, enabling the transition from high-frequency synchronous firing through NKB-driven activation of TRPC5 channels to a short bursting mode facilitating glutamate release. In a low E2 milieu, synchronous firing of Kiss1ARH neurons drives pulsatile release of GnRH, while the transition to burst firing with high, preovulatory levels of E2 would facilitate the GnRH surge through its glutamatergic synaptic connection to preoptic Kiss1 neurons.