Checkpoint Proteins: Closing the Mad2 cycle
Enzymes and other proteins constantly undergo changes of conformation in our cells. In most cases these changes do not involve any change in the topology of the protein: that is, the relative positions of the secondary structure elements of the protein (such as alpha helices and beta sheets) do not change. A family of proteins called HORMA domain proteins has emerged as a fascinating exception to this empirical rule. Mad2, the best-studied member of this family, changes between an open state that is inactive and a closed (active) state with a different topology (Figure 1). Crucial details of this process have remained unclear but now, in eLife, Kevin Corbett and co-workers—including Qiaozhen Ye as first author—shed new light on the role played by an enzyme called TRIP13/PCH-2 in this particular conformational change (Ye et al., 2015).
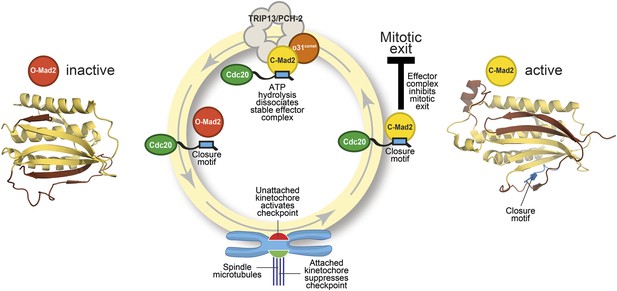
The Mad2 cycle.
The Mad2 protein exists in an ‘inactive’ open form (left) and an ‘active’ closed form (right). The structural elements highlighted in pale yellow have the same relative positions in the two states; the elements highlighted in brown have different relative positions. The open form (red) is converted to the closed form (bright yellow) by a closure motif (blue rectangle) within a Cdc20 protein. This process is strongly promoted by kinetochores that have not yet bound to the spindle (red), but not by those that are already bound (green). This results in the formation of the effector complex (containing closed Mad2 and Cdc20) that suppresses the separation of the chromosomes. A protein called p31comet acts as a bridge to allow an enzyme called TRIP13/PCH-2 to use ATP hydrolysis to dissociate this effector complex, which is very stable.
Before a cell divides, its chromosomes need to be duplicated and then separated into two groups so that each daughter cell gets a full set of chromosomes. To achieve this, protein complexes called kinetochores connect the chromosomes to a structure called the spindle, which pulls the chromosomes to opposite ends of the cell. This process is monitored by a set of proteins known as checkpoint proteins.
Mad2 is a checkpoint protein that is recruited in its open (inactive) form to kinetochores that are not yet properly attached to the spindle. It is converted to the closed (active) form by binding to a ‘closure motif’ in a protein called Cdc20. The closed Mad2 protein then joins forces with Cdc20 and other proteins to create a checkpoint effector complex that prevents the chromosomes separating until all of them are attached to the spindle (Figure 1).
When purified samples of Mad2 and Cdc20 are mixed in the laboratory, they bind together spontaneously. However, this process is very slow because a large amount of activation energy is needed to convert the open state of Mad2 into the closed state. Inside the cell, however, the kinetochores act as catalysts to accelerate the reaction through steps that are only partly understood (Lara-Gonzalez et al., 2012).
Several lines of evidence indicate that closed Mad2 can be converted back into the open form. Moreover, even when the checkpoint effector is active, some open Mad2 is always present: this allows the open form of the protein to be recruited to kinetochores that are not yet attached to the spindle. This pool of open Mad2 is not maintained through the production of new protein, so the most plausible explanation is that it comes from the continuous conversion of closed Mad2. How does this take place? Previous studies have established that the hydrolysis of ATP is required to disassemble the checkpoint effector (Miniowitz-Shemtov et al., 2010): hydrolysis of ATP releases energy, but the specific steps that require this energy had not been identified.
The key to gaining a molecular understanding was the characterization of two proteins—TRIP13/PCH2 and p31comet—that were known to be involved in disassembling the effector complex formed by closed Mad2 and Cdc20 (Eytan et al., 2014; Wang et al., 2014). By recreating the reaction in the laboratory with purified components, Ye et al.—who are based at the Ludwig Institute for Cancer Research, the Scripps Institute and the University of California, San Diego—now go further by showing that the TRIP13/PCH-2 enzyme binds to closed Mad2, which is then converted to open Mad2 in a manner that depends on ATP hydrolysis and on the activity of p31comet (Figure 1).
TRIP13/PCH-2 is a member of the AAA+ ATPase family of enzymes: these enzymes, which are found in all kingdoms of life, harness the chemical energy released by ATP hydrolysis to perform mechanical work in a variety of different reactions (Hanson and Whiteheart, 2005; Vader, 2015). For instance, an important subclass of these enzymes—known as the disaggregases—can separate proteins that have formed aggregates within the cell: this allows these proteins to refold with assistance from chaperone proteins. Another subclass assists in the breakdown of proteins by associating with protease enzymes, and a third subclass promotes the disassembly of stable protein complexes and the recycling of their subunits. TRIP13/PCH-2 appears to belong to the latter class, but Ye et al. reveal that its high-resolution three-dimensional structure is more typical of the disaggregases.
As if the pattern of events surrounding the conversion of Mad2 had not been complex enough, p31comet is also a member of the HORMA domain family and is able to selectively interact with closed Mad2 (Yang et al., 2007). Ye et al. now demonstrate that p31comet binds to Mad2 and TRIP13/PCH2 at the same time, and acts as a bridge between them (Figure 1).
TRIP13/PCH-2 can also regulate a group of HORMA domain proteins that are involved at multiple stages of a type of cell division called meiosis (Vader, 2015). The significance of this regulation was not clear at first, but recent studies have revealed that two of these HORMA domain proteins interact with the closure motifs in their binding partners, indicating that their safety belt is, like that of Mad2, a variable element of their topology (Chen et al., 2014; Kim et al., 2014).
Collectively, these recent studies show that TRIP13/PCH-2 is a general regulator of a topological conversion that occurs when HORMA domains bind to their partner proteins. The techniques described by Corbett, Ye and co-workers are without doubt a promising way forward for the further investigation of this fascinating problem.
References
-
Pch2 is a hexameric ring ATPase that remodels the chromosome axis protein Hop1Proceedings of the National Academy of Sciences of USA 111:E44–E53.https://doi.org/10.1073/pnas.1310755111
-
Disassembly of mitotic checkpoint complexes by the joint action of the AAA-ATPase TRIP13 and p31(comet)Proceedings of the National Academy of Sciences of USA 111:12019–12024.https://doi.org/10.1073/pnas.1412901111
-
AAA+ proteins: have engine, will workNature Reviews Molecular Cell Biology 6:519–529.https://doi.org/10.1038/nrm1684
-
The spindle assembly checkpointCurrent Biology 22:R966–R980.https://doi.org/10.1016/j.cub.2012.10.006
-
ATP is required for the release of the anaphase-promoting complex/cyclosome from inhibition by the mitotic checkpointProceedings of the National Academy of Sciences of USA 107:5351–5356.https://doi.org/10.1073/pnas.1001875107
-
Thyroid hormone receptor interacting protein 13 (TRIP13) AAA-ATPase is a novel mitotic checkpoint-silencing proteinJournal of Biological Chemistry 289:23928–23937.https://doi.org/10.1074/jbc.M114.585315
Article and author information
Author details
Publication history
Copyright
© 2015, Musacchio
This article is distributed under the terms of the Creative Commons Attribution License, which permits unrestricted use and redistribution provided that the original author and source are credited.
Metrics
-
- 1,478
- views
-
- 279
- downloads
-
- 5
- citations
Views, downloads and citations are aggregated across all versions of this paper published by eLife.
Download links
Downloads (link to download the article as PDF)
Open citations (links to open the citations from this article in various online reference manager services)
Cite this article (links to download the citations from this article in formats compatible with various reference manager tools)
Further reading
-
- Biochemistry and Chemical Biology
- Microbiology and Infectious Disease
Malaria parasites have evolved unusual metabolic adaptations that specialize them for growth within heme-rich human erythrocytes. During blood-stage infection, Plasmodium falciparum parasites internalize and digest abundant host hemoglobin within the digestive vacuole. This massive catabolic process generates copious free heme, most of which is biomineralized into inert hemozoin. Parasites also express a divergent heme oxygenase (HO)-like protein (PfHO) that lacks key active-site residues and has lost canonical HO activity. The cellular role of this unusual protein that underpins its retention by parasites has been unknown. To unravel PfHO function, we first determined a 2.8 Å-resolution X-ray structure that revealed a highly α-helical fold indicative of distant HO homology. Localization studies unveiled PfHO targeting to the apicoplast organelle, where it is imported and undergoes N-terminal processing but retains most of the electropositive transit peptide. We observed that conditional knockdown of PfHO was lethal to parasites, which died from defective apicoplast biogenesis and impaired isoprenoid-precursor synthesis. Complementation and molecular-interaction studies revealed an essential role for the electropositive N-terminus of PfHO, which selectively associates with the apicoplast genome and enzymes involved in nucleic acid metabolism and gene expression. PfHO knockdown resulted in a specific deficiency in levels of apicoplast-encoded RNA but not DNA. These studies reveal an essential function for PfHO in apicoplast maintenance and suggest that Plasmodium repurposed the conserved HO scaffold from its canonical heme-degrading function in the ancestral chloroplast to fulfill a critical adaptive role in organelle gene expression.
-
- Biochemistry and Chemical Biology
- Cell Biology
Activation of the Wnt/β-catenin pathway crucially depends on the polymerization of dishevelled 2 (DVL2) into biomolecular condensates. However, given the low affinity of known DVL2 self-interaction sites and its low cellular concentration, it is unclear how polymers can form. Here, we detect oligomeric DVL2 complexes at endogenous protein levels in human cell lines, using a biochemical ultracentrifugation assay. We identify a low-complexity region (LCR4) in the C-terminus whose deletion and fusion decreased and increased the complexes, respectively. Notably, LCR4-induced complexes correlated with the formation of microscopically visible multimeric condensates. Adjacent to LCR4, we mapped a conserved domain (CD2) promoting condensates only. Molecularly, LCR4 and CD2 mediated DVL2 self-interaction via aggregating residues and phenylalanine stickers, respectively. Point mutations inactivating these interaction sites impaired Wnt pathway activation by DVL2. Our study discovers DVL2 complexes with functional importance for Wnt/β-catenin signaling. Moreover, we provide evidence that DVL2 condensates form in two steps by pre-oligomerization via high-affinity interaction sites, such as LCR4, and subsequent condensation via low-affinity interaction sites, such as CD2.