Multipotent versus differentiated cell fate selection in the developing Drosophila airways
Abstract
Developmental potentials of cells are tightly controlled at multiple levels. The embryonic Drosophila airway tree is roughly subdivided into two types of cells with distinct developmental potentials: a proximally located group of multipotent adult precursor cells (P-fate) and a distally located population of more differentiated cells (D-fate). We show that the GATA-family transcription factor (TF) Grain promotes the P-fate and the POU-homeobox TF Ventral veinless (Vvl/Drifter/U-turned) stimulates the D-fate. Hedgehog and receptor tyrosine kinase (RTK) signaling cooperate with Vvl to drive the D-fate at the expense of the P-fate while negative regulators of either of these signaling pathways ensure P-fate specification. Local concentrations of Decapentaplegic/BMP, Wingless/Wnt, and Hedgehog signals differentially regulate the expression of D-factors and P-factors to transform an equipotent primordial field into a concentric pattern of radially different morphogenetic potentials, which gradually gives rise to the distal-proximal organization of distinct cell types in the mature airway.
https://doi.org/10.7554/eLife.09646.001eLife digest
Many organs are composed of tubes of different sizes, shapes and patterns that transport vital substances from one site to another. In the fruit fly species Drosophila melanogaster, oxygen is transported by a tubular network, which divides into finer tubes that allow the oxygen to reach every part of the body.
Different parts of the fruit fly’s airways develop from different groups of tracheal precursor cells. P-fate cells form the most 'proximal' tubes (which are found next to the outer layer of the fly). These cells are 'multipotent' stem cells, and have the ability to specialize into many different types of cells during metamorphosis. The more 'distal' branches that emerge from the proximal tubes develop from D-fate cells. These are cells that generally acquire a narrower range of cell identities.
By performing a genetic analysis of fruit fly embryos, Matsuda et al. have now identified several proteins and signaling molecules that control whether tracheal precursor cells become D-fate or P-fate cells. For example, several signaling pathways work with a protein called Ventral veinless to cause D-fate cells to develop instead of P-fate cells. However, molecules that prevent signaling occurring via these pathways help P-fate cells to form. Different amounts of the molecules that either promote or hinder these signaling processes are present in different parts of the fly embryo; this helps the airways of the fly to develop in the correct pattern.
This work provides a comprehensive view of how cell types with different developmental potentials are positioned in a complex tubular network. This sets a basis for future studies addressing how the respiratory organs – and indeed the entire organism – are sustained.
https://doi.org/10.7554/eLife.09646.002Introduction
Multipotent stem cells are essential for both growth and generation of cell diversities in developing organs. They also serve as reservoir to replace damaged or aged cells during physiological or pathological tissue homeostasis. The discovery of a small number of transcription factors that can induce pluripotent cells (Takahashi and Yamanaka, 2006) has fueled major research efforts to reveal the mechanisms biasing the choice between pluripotent/multipotent and more differentiated cell fates in vivo (O'Brien and Bilder, 2013). Using the Drosophila airways (tracheal system) (Manning and Krasnow, 1993; Samakovlis et al., 1996b) (Figure 1A), we studied how the initial selection of different potencies within an organ becomes first predisposed and then regionally confined.
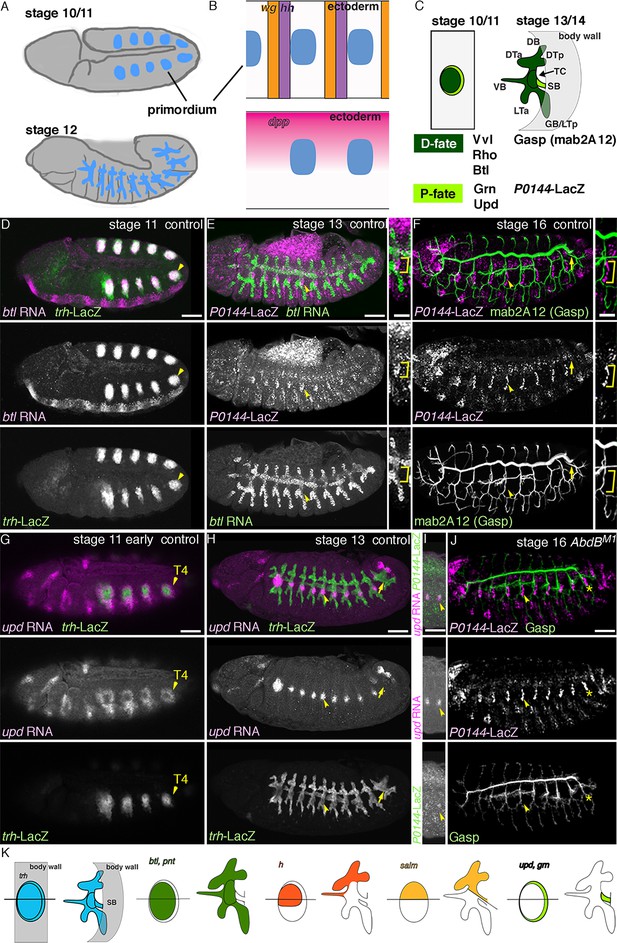
The proximo-distal cell fate organization of the Drosophila airways.
(A) A sketch of the embryonic Drosophila airways at stage 10/11 and late stage 12. (B) A representation of the ectodermal expression the secreted signaling molecules wg/WNT, hh (upper panel), and dpp (lower panel) in relation to the airway primordia at stage 10. (C–J) Expression of the P/D-fate markers at different stages of the airway development, which is summarized schematically in C, where the regional diversification of the Drosophila airway according to the proximo-distal axis is also shown in different colors. The P-fate region: spiracular branch (SB). The D-fate region: transverse connectives (TC) and six primary branches (dorsal branch/DB, dorsal trunk anterior/DTa, dorsal trunk posterior/DTp, visceral branch/VB, lateral trunk anterior/LTa, and ganglionic branch/GB/lateral trunk posterior/LTp). Expression of the D-fate markers, btl (D,E), mab2A12 (F, J) and the P-fate markers, P0144-lacZ (E, F, I, J) and upd (G-I), relative to each other or trh-lacZ (D, G, H). In this and other figures, arrowheads mark one of the 10 metameres. In E-F, enlarged picture of Tr5 is also shown, where the P-fate cells are bracketed. btl expression at stage 11 (D) is concentrated in the central parts of the primordia. At stage 13 (E), btl expression and P0144-lacZ expression (arrowheads) rarely overlap. At stage 16, mab2A12 (anti-Gasp antibody) strongly labels the lumens of the D-cells while P0144-lacZ is strongly detected in the P-cells (F, arrowheads). At stage 11 (G), upd transcript is detected at the peripheral area of each primordium (arrowheads). At stage 13 (H, I), upd is expressed in the P-cells that express P0144-lacZ (arrowheads). Note that compared to the control, where upd and P0144-lacZ are not expressed in Tr10 (arrows in F, H), the P-fate (P0144-lacZ) is established in Tr10 of AbdB mutants (asterisks in J). Scale bar is 2 μm in the enlarged panels of E-F. Scale bar in the remaining panels is 50 μm. (K) summarizes typical gene expression patterns of various marker genes in the airway primordium and in the mature airways. See text for details.
By mid-embryogenesis, Drosophila embryos are metamerically divided along the anterior-posterior (AP) axis (Ingham, 1988; Sanson, 2001). Each unit (segment or parasegment) is also subdivided along the dorso-ventral (DV) embryo axis (Anderson et al., 1985b; Wharton et al., 1993). Generation of the airway primordia is spatially restricted along these AP or DV axis, by the local concentrations of repressing and activating signals (Bier et al., 1989; Isaac and Andrew, 1996; Perrimon et al., 1991; Wilk et al., 1996). For example, Wingless (Wg) expressed in stripes along the AP axis and Decapentaplegic (Dpp) expressed in the dorsal ectoderm (Figure 1B) repress the airway primordia specification (Isaac and Andrew, 1996; Wilk et al., 1996). As a result, 10 metameric groups of cells expressing a master gene, Trachealess (Trh) are specified on each side of the lateral ectoderm (Figure 1A–B). Invagination of each primordial cluster transforms the two-dimensional (2D) shape of each cluster to 3D tubes (Figure 1C) (Campos-Ortega and Hartenstein, 1985; Turner and Mahowald, 1977). Within the invaginated primitive sacs/tubes, the proximal cells form a narrow cord, the spiracular branch/SB (P-fate) (Figure 1C, K) and stay multipotent to later proliferate and to generate many parts of the pupal/adult airways (Manning and Krasnow, 1993; Pitsouli and Perrimon, 2010; Weaver and Krasnow, 2008). Within the remaining distal cells (D-fate), the more distal parts achieve a series of morphogenetic events, extending six primary branches, fusing with branches from neighboring metameres and supplying oxygen directly to the target cells (Manning and Krasnow, 1993; Samakovlis et al., 1996a; Samakovlis et al., 1996b), while the more proximal parts (transverse connectives/TC) connect SB with the primary branches (Figure 1C, K).
The primary branches constitute D-fate cells located distally from TC, and their morphological diversification depends on the activation of various signaling pathways. For example, rhomboid (rho) is expressed in each primordium (Bier et al., 1990) and activates dEGFR (also called torpedo or faint little ball) (Price et al., 1989; Schejter and Shilo, 1989) by generating the secreted active ligand Spitz (s-Spi) (Gabay et al., 1997; Golembo et al., 1996; Schweitzer et al., 1995b; Urban et al., 2001; Wappner et al., 1997). Activated dEGFR instructs the cytoskeletal changes that coordinate invagination (Brodu and Casanova, 2006; Kondo and Hayashi, 2013; Llimargas and Casanova, 1999; Nishimura et al., 2007). Branchless (Bnl)/dFGF is expressed in patches of surrounding tissues to guide primary branching (Sutherland et al., 1996) by activating Breathless (Btl)/dFGFR on the airway cells (Klambt et al., 1992; Lee et al., 1996). Apart from receptor tyrosine kinase (RTK) signaling, Decapentaplegic (Dpp)/BMP, Wingless (Wg)/WNT, and Hedgehog (Hh) signals combinatorially establish different primary branch identities (Affolter et al., 1994; Chen et al., 1998; Chihara and Hayashi, 2000; Glazer and Shilo, 2001; Llimargas, 2000; Llimargas and Lawrence, 2001; Matsuda et al., 2015; Vincent et al., 1997). Although many studies focused on the differentiation and subsequent morphogenesis of the D-fate cells (Beitel and Krasnow, 2000; Cabernard et al., 2004; Ghabrial et al., 2011; Hosono et al., 2015), generation of the P-fate has been underexplored. Our current dissection of P/D fate establishment in the embryo, together with metamere-specific control programs of differentiation/de-differentiation in the larval airways (Djabrayan et al., 2014; Guha et al., 2008; Sato et al., 2008; Weaver and Krasnow, 2008) may broaden our understanding of the strategies for stem cell selection and maintenance in vivo.
Results and discussion
The proximo-distal cell fate organization in the Drosophila airway tree
Every cell in the main airways derives from 20 primordial cell clusters expressing the master regulator TF Trh (Figure 1A–C, K) (Isaac and Andrew, 1996; Perrimon et al., 1991; Wilk et al., 1996). However, the expression of several other genes is enriched either distally or proximally in the airways. rho is expressed preferentially in the central and distal regions of the airway primordia before and during invagination (Figure 1—figure supplement 1A–E). btl/dFGFR is also expressed preferentially in the distal part (Figure 1D–E), and its expression is upregulated by Bnl/dFGF in the most distal leading cells of the primary branch tips (Figure 1E) (Ohshiro et al., 2002; Ohshiro and Saigo, 1997). mAb2A12 detecting the putative chitin-biding protein Gasp (Tiklova et al., 2013) preferentially labels the distal airways during stages 14-17 (Figure 1F) (Samakovlis et al., 1996a). On the other hand, unpaired (upd), a ligand activating the JAK/STAT signaling is expressed only in the proximal SB cells from stage 12 on (Figure 1G–I) (Harrison et al., 1998). From stage 13 onwards, the SB cells also show stronger expression of the P0144 enhancer trap marker (Figure 1E,F,I) (http://flyview.uni-muenster.de). Compared to the anterior metameres, the 10th tracheal primordium (Tr10) does not establish the P0144-postive P-fate due to the high level of a Hox protein Abdominal-B (AbdB) in this part of the embryo (Figure 1F, J), (Celniker et al., 1989; Matsuda et al., 2015). In trh mutants, both the D-fate marker (Chung et al., 2011; Jin et al., 2001; Ohshiro and Saigo, 1997; Zelzer and Shilo, 2000) and the P-fate marker expressions (Figure 1—figure supplement 1U, V) are lost.
To define the origins of the proximo-distal organization of the airway tree, we labeled cell groups during the 2D primordial stage and recorded their fates in the 3D tree. pointed (pnt) is a general transcriptional target and mediator of RTK signaling (Brunner et al., 1994; Klambt, 1993; O'Neill et al., 1994). rho induces pnt expression in the central parts of the airway primordia (Figure 1—figure supplement 1F–O) and btl/dFGFR upregulates pnt expression in the tips of the primary branches (Figure 1—figure supplement 1M–O) (Samakovlis et al., 1996a). Markers of the central parts of the airway primordia (pnt-lacZ and btl-CD8-GFP) become preferentially expressed in the distal part of the airway tree (Figure 1K, Figure 1—figure supplement 1N–O). Similarly, hairy (h) expression in the airway primordia (Carroll et al., 1988; Hooper et al., 1989; Zhan et al., 2010) is confined to the dorso-central part (Figure 1—figure supplement 1P) and its reporter (h-lacZ) becomes active in most of the DB, VB, and DT cells but not in TC, SB nor in ventral branches (Figure 1K, Figure 1—figure supplement 1Q) (Samakovlis et al., 1996a). In contrast, salm labels the dorsal part of the airway primordia (Figure 1—figure supplement 1R), (Kuhnlein and Schuh, 1996) and salm-gal4 driven UAS-GFP marks DB and DT, and parts of TC and SB in the airways (Figure 1K, Figure 1—figure supplement 1S–T). These results suggest that the dorso-peripheral sector of each primordium, which is positive for salm but lacking h, composes half of the proximal SB cells in the airway tree. The other half may originate from the ventro-peripheral sector of the primordia. Consistently, around the completion of invagination, the P-fate marker upd is enriched in a horseshoe-like peripheral ring (Figure 1G)(Harrison et al., 1998), which eventually marks the SB cells at stage 12 (Figure 1H,I,K). Collectively, the gene expression analysis suggests a fate map of the airway primordia, where the centro-peripheral axis inversely correlates both with the ordered pattern of tracheal cell invagination (Brodu and Casanova, 2006; Nishimura et al., 2007) and the PD axis of airway branching (Figure 1C,K).
RTK signaling drives the D-fate selection at the expense of the P-fate
As activation of RTKs propels several morphogenetic programs in the D-cells, we first assessed their effects on the discrimination of the P- and the D-fate. In btl/dFGFR mutants, where active branch extension does not occur, apoptotic staining in the distal cells increases from stage 12 (Figure 2—figure supplement 1A,B), suggesting that btl/dFGFR signaling directly or indirectly suppresses apoptosis of the distal airways. In dEGFR btl/dFGFR double mutants, massive cell death staining accompanies loss of most tracheal cells compared to either single mutant (Figure 2—figure supplement 1B–D). Suppression of apoptosis by removing pro-apoptotic genes using Df(H99) (Figure 2—figure supplement 1E) (White et al., 1994) or by transgenic expression of the apoptosis inhibitor, DIAP (Figure 2—figure supplement 1F) (Hay et al., 1995) partially restores the survival of tracheal cells in dEGFR btl/dFGFR double mutants (Figure 2—figure supplement 1E–J). Thus, dEGFR and Btl/dFGFR cooperate to suppress apoptosis in the airway cells. To analyze the effects of RTK signaling in the P/D fate choice independently of cell survival, we introduced Df(H99) into some of the genotypes used in the analysis described below.
In bnl/dFGF mutants, the feedback upregulation of the D-fate marker btl/dFGFR is lost in the tips of the primary branches (Figure 2A,B) (Ohshiro et al., 2002). However, the distal part of the invaginated stump retains the mab2A12 signal (Lee et al., 1996) and the basal btl/dFGFR expression (Figure 2B). Similarly, expression of the P-fate markers (upd and P0144-lacZ) in btl/dFGFR mutants remains largely comparable to the wild type (Figure 2E–F, I–J, M). These results suggest that Btl/dFGFR signaling does not play a major role in the P-D discrimination. In rho mutants, however, the refinement of upd expression to the primordia periphery is retarded (Figure 2—figure supplement 1K,L), and the expression domains of upd and P0144-lacZ become expanded (Figure 2G, K, M). Correspondingly, the domain of btl-expressing cells is decreased (Figure 2C) suggesting that the rho-mediated dEGFR signaling drives the D-fate selection at the expense of the P-fate. We investigated the possible redundant functions of the two RTKs in D-fate definition. In rho bnl/dFGF double mutants, btl expression is further reduced or even lost in some segments (Figure 2D). Correspondingly, in rho btl/dFGFR double mutants, another D-fate marker mab2A12/Gasp becomes reduced or undetectable in some segments, accompanied by a concomitant increase of the proximal P0144-lacZ expression domain (Figure 2L, N). Consistent with the phenotypes of rho btl/dFGFR mutants, P0144-lacZ expression is also increased in pnt mutants, (Figure 2M, Figure 2—figure supplement 1P). Taken together, we conclude that dEGFR signaling in cooperation with btl/dFGFR signaling promotes the D-fate selection at the expense of the P-fate partly through pnt (Figure 2O). The residual weak expression of the D-fate markers in rho btl or dEGFR btl/dFGFR double mutants (Figure 2L, Figure 2—figure supplement 1M–O) suggests additional inputs in the D fate selection, independent of RTK signaling downstream of dEGFR and btl/dFGFR (Figure 2O).
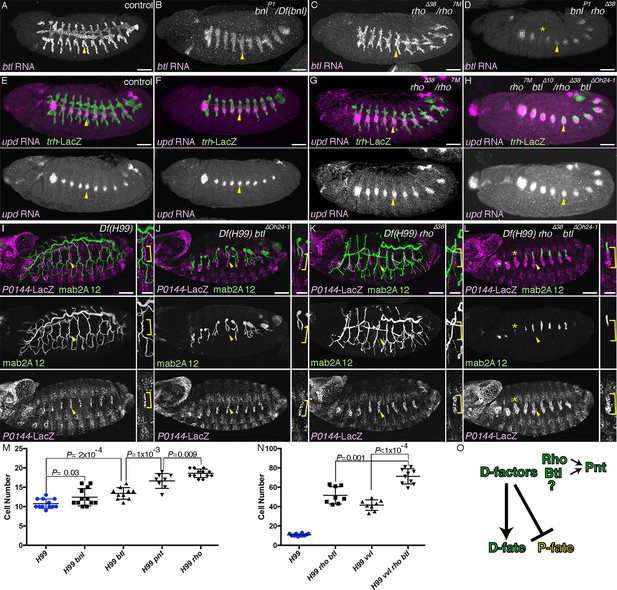
RTK activation drives the D-fate selection.
Expression of the D-fate (btl in A-D and mab2A12 in I-L) and the P-fate (upd in E-H and P0144-lacZ in I-L) markers upon loss of dEGFR and/or btl/dFGFR signaling. (A–H) Stage 12/13. (I–L) Stage 16. Compared to the control (A, E, I), in bnl mutants (B), btl mutants (F) or Df(H99) btl mutants (J), expression of the D-fate markers btl (A) and mab2A12 (J) and the P-fate markers upd (F) and P0144-lacZ (J) appears similar. Note, however, that upregulation of btl in a pan-tip pattern is abolished in bnl mutants (B) (Ohshiro et al., 2002). In rho mutants (C, G) or Df(H99) rho mutants (K), the expression area of the P-fate markers upd (G) and P0144-lacZ (K, arrowheads) is increased, leading to the decreased area of the D-fate marker expression (C, K). In rho bnl double mutants (D), rho btl double mutants (H) or Df(H99) rho btl triple mutants (L), the P-fate area is further expanded with concomitant reduction of the D-fate markers. Note that in D,L expression of the D-fate markers becomes reduced very much in a few segments (asterisk). Scale bar is 2 μm in the enlarged panels of I-L where the P-fate cells are bracketed. Scale bar in the remaining panels is 50 μm. (M,N) Scatter plots of the number of P0144-lacZ positive P-fate cells in Tr5 and Tr6 of the indicated genotypes at stage 16. All p-values were calculated by Student’s t-test. Source files are supplied in Figure 2—source data 1,2. (O) A scheme of P/D-fate selection by RTK signaling components. RTK, receptor tyrosine kinase.
-
Figure 2—source data 1
Source data for Figure 2M.
The number of P0144-lacZ-positive P-fate cells in Tr5 and Tr6 of the indicated genotypes at stage 16.
- https://doi.org/10.7554/eLife.09646.006
-
Figure 2—source data 2
Source data for Figure 2N.
The number of P0144-lacZ-positive P-fate cells in Tr5 and Tr6 of the indicated genotypes at stage 16.
- https://doi.org/10.7554/eLife.09646.007
vvl drives the D-fate selection at the expense of the P-fate
The expression of two key components of RTK signaling, rho in the central part of the airway primordia and btl in the extending distal airways, partly depends on the POU-domain transcription factor vvl (Anderson et al., 1996; Llimargas and Casanova, 1997). We confirmed that in vvl mutants, expression of the D-fate markers rho and btl is reduced (Figure 3A, B, E, F). Moreover, mAb2A12 staining is almost diminished (Figure 3N, O) in the residual distal branches, which is restored by UAS-vvl driven with btl-gal4 (Figure 3—figure supplement 1T). By contrast, the expression of the P-fate marker upd is expanded to the distal branches at stage 12 (Figure 3J–K) and the P0144-lacZ domain is significantly increased at stage 15 in vvl mutants (Figure 2N, 3O). This indicates that vvl promotes the D-fate at the expense of the P-fate, and a part of this function may be achieved through vvl-dependent expression of rho and btl. In wild type, vvl expression is enriched in the distal trachea, indicating that vvl itself is a D-fate marker (Figure 3—figure supplement 1A–C).
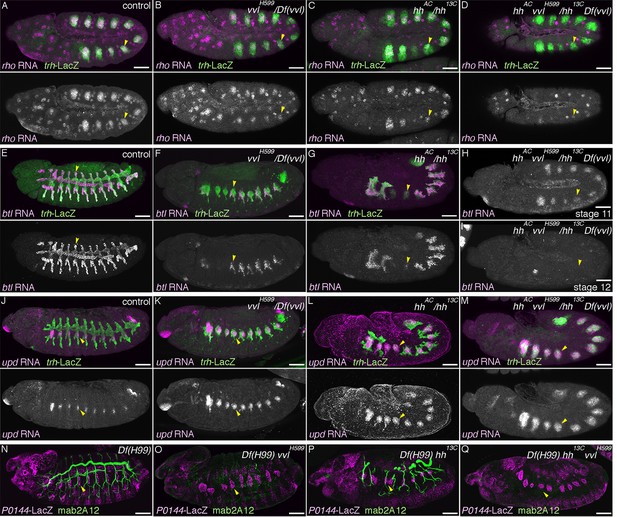
Hh and Vvl drive the D-fate selection.
Expression of the D-fate markers (rho at stage 11 in A–D, btl at stages 11-13 in E–I and mab2A12 at stages 15/16 in N–Q) and the P-fate markers (upd at stages 12/13 in J–M, P0144-lacZ in N-Q) upon loss of vvl and/or hh. Note that the genotypes in (N-Q) additionally carry the Df(H99) mutation. Compared to the control (column 1), upon loss of vvl (column 2), the expression area of the D-fate markers rho (B), btl (F), and mab2A12 (Q) is decreased while expression of the P-fate markers upd (K) and P0144-lacZ (Q) is expanded. Note that mab2A12 signals are hardly detectable in O. hh mutants (column 3) show the same trend as vvl mutants. Expression of btl (G) and mab2A12 (P) is variably lost in Tr3/4 and upd expression (P) expands to the whole distal area. In hh vvl double mutants (column 4), expression of the D-fate markers (D,I) is lost, while expression of the P-fate markers (M,Q) persist in the whole trachea. Note that weak btl expression is detectable in the primordia (H) but is lost soon after (I). Scale bar: 50 μm.
The residual expression of the RTK signaling components in vvl mutant may function as D-factors in the absence of vvl. To evaluate the relative contributions of vvl and RTKs in the P/D fate selection, we examined double or triple mutants of vvl and components of the two RTK signaling pathways. Compared to single or double mutants (Figure 3—figure supplement 1E, F), in vvl rho bnl triple mutant embryos, the expression of the D-fate marker btl is initiated at stage11 but is not maintained by stage 12 (Figure 3—figure supplement 1G,H). Consistently, the mab2A12 signal is undetectable in vvl rho btl mutants (Figure 3J–L). In contrast, expression of the P-fate markers upd and P0144-lacZ appears to be expressed in all tracheal cells (Figure 2N, Figure 3—figure supplement 1I, L). These results suggest that the two RTK pathways act both downstream of and in parallel to vvl to drive the D-fate selection at the expense of the P-fate.
Hh and Vvl drive the D-fate selection
Hh is expressed just anteriorly to the airway primordia (Glazer and Shilo, 2001) and is proposed to enhance the primordial vvl expression (Llimargas and Casanova, 1997). We confirmed that in hh mutants, vvl expression is variably decreased or lost (Figure 3—figure supplement 1D). Consistently, expression of the D-fate markers rho and btl is also variably decreased or lost in hh mutants (Figure 3C,G, in 6 out of 26 embryos for btl expression). In contrast, expression of the P-fate marker upd is expanded and sometimes occupies the whole trachea in some segments of hh mutants (Figure 3L). A similar effect is observed on the expression of a different set of the P/D fate markers, mab2A12/Gasp and P0144-lacZ (Figure 3P). These results suggest that Hh signals from the anterior border of the airway primordia to orient the P/D fate selection toward the D-fate direction. Similar to the cooperative activities of rho, btl, and vvl, hh could synergize with vvl to drive the D-fate. Indeed, in the absence of both hh and vvl, although expression of the D-fate markers rho and btl is variably detected in the primordia (Figure 3D,H), btl expression is completely absent later (Figure 3I). Correspondingly, the expression of P-fate markers expands to all tracheal cells (Figure 3M,Q). A similar trend was observed in mutants for hh and RTK signaling components (Figure 3—figure supplement 1M–S). Taken together, we conclude that Hh and Vvl cooperatively drive the D fate selection, partly through the activation of dEGFR and btl/dFGFR signaling in the central part of the primordium and in the distal branches.
Grn promotes the P-fate selection
The identification of several signaling pathways converging in the initiation and establishment of the D-fate in the airways prompted us to interrogate what promotes the P-fate. We revealed the GATA-family TF Grn (Brown and Castelli-Gair Hombria, 2000; Garces and Thor, 2006; Lin et al., 1995) as the P-fate promoting factor. grn is preferentially expressed in the peripheral parts of the airway primordia from stage 11 on (Figure 4—figure supplement 1A). At stage 13, the P-fate cells of the SB and the overlying lateral ectoderm are positive for grn (Figure 4—figure supplement 1B–C). grn expression is repressed in the D-cells by the D-fate determinants, hh, vvl, and RTKs (Figure 4—figure supplement 1D–G), while grn expression in the lateral ectoderm is largely intact in the absence of tracheal cells in upd or trh mutants (Figure 4—figure supplement 1H–I). Grn overexpression results in the upregulation of the P-fate marker P0144-lacZ in the more distal TC branches (Figure 4A–C). Conversely, in grn mutants, expression of the P-fate markers upd and P0144-lacZ is lost (Figure 4D–F, Figure 4—figure supplement 1J), resulting in mAb2A12-positive tubes directly connecting to the epidermis. Counting the Trh-positive cells in Tr5 and in TC5/SB5 reveals that around 10 cells are selectively lost from the TC/VB region (Figure 4G). As halving of the airway cell number in CycA mutants (Beitel and Krasnow, 2000) does not abolish the P-fate (Figure 4H, Figure 4—figure supplement 1K), we suggest that reduction of cell number does not account for the loss of the P-fate in grn mutants. Also, arm-gal4-mediated overexpression of Trh in grn mutants does not restore the P-fate (Figure 4H, Figure 4—figure supplement 1L). These results suggest that grn functions in two ways, namely to establish Trh expression in the P-region and to induce the P-fate marker expression (Figure 4K). Accordingly, we designate grn a master regulator of the P-fate.
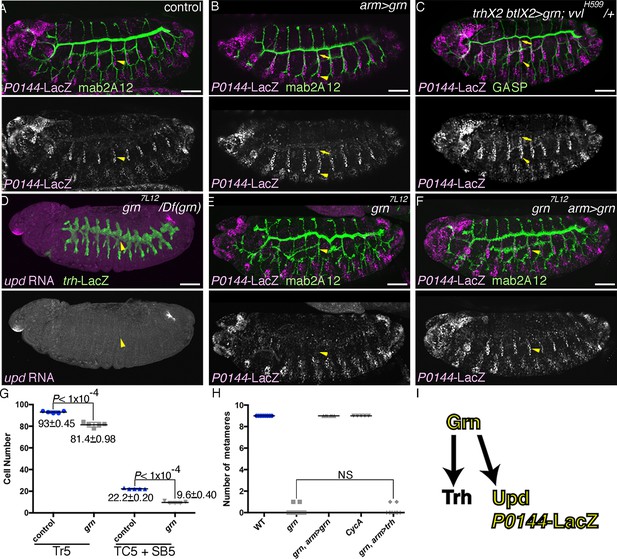
Grn promotes the P fate.
Compared to the control (A), grn overexpression with arm-gal4 (B) or two copies of btl-gal4 and trh-gal4 (C) slightly expands the area of P0144-lacZ positive cells to more distal TC areas, especially in Tr2-5 (B-C, arrows). In grn mutants, expression of the P-fate markers upd (D) or P0144-lacZ (E) disappears while arm-gal4-mediated Grn overexpression restores P0144-lacZ expression in the proximal cells (F). (G) Scatter plots of the Trh-positive cell numbers in the whole Tr5 or in the SB/TC subregion of the indicated genotypes at stage 14 (mean ± SEM, N = 5; all p-values were calculated by Student’s t-test). The cell number is decreased by around 10 cells in grn mutants in Tr5 and in the SB/TC subregion. A source file is supplied in Figure 4—source data 1. (H) Scatter plots of the number of metameres with any number of P0144-lacZ-positive cells of the indicated genotypes at stages15/16 (NS: not significant by Student’s t-test). Note that grn mutants occasionally possess a few P0144-lacZ cells in anterior metameres. A source file is supplied in Figure 4—source data 2. (I) summarizes the two functions of grn in P-fate promotion. Scale bar: 50 μm. SB, spiracular branch; SEM, standard error of the mean, TC, transverse connectives.
-
Figure 4—source data 1
Source data for Figure 4G.
The Trh-positive cell numbers in the whole Tr5 or in the SB/TC subregion of the indicated genotypes at stage 14.
- https://doi.org/10.7554/eLife.09646.012
-
Figure 4—source data 2
Source data for Figure 4H.
The number of metameres with any number of P0144-lacZ-positive cells of the indicated genotypes at stages 15/16.
- https://doi.org/10.7554/eLife.09646.013
Effects of simultaneous loss of the D- and P-factors
Having identified several regulators of the D- and P-fates, we examined their epistatic relationships. Compared to the control (Figure 5A, Figure 5—figure supplement 1E, J), in grn hh double- (Figure 5C, Figure 5—figure supplement 1G, L) or grn rho btl triple mutants (Figure 5—figure supplement 1I, N), the P-fate marker expression is not robustly restored. In grn vvl double mutants, the trh expression area appears to be very much reduced (Figure 5F), probably because the P-fate cells depend on grn for trh expression. However, in this reduced area of the distal region, we detected weak restoration of the P-fate markers upd and P0144-lacZ (Figure 5B, Figure 5—figure supplement 1F, K). These results may suggest the presence of another factor in the distal area that promotes the P-fate when grn and vvl are inactivated. Alternatively, the default airway cell fate is the proximal and multipotent cell fate (the P-fate) and an important aspect of the grn functions in P-fate selection/promotion maybe to antagonize the D-factor vvl. In the absence of both the D- and P-factors (Figure 5H, Figure 5—figure supplement 2A–B), trh expression initiates at stage 10 but becomes drastically reduced by stage 12/13 (Figure 5E–H). In these mutants (Figure 5D, Figure 5—figure supplement 1D, H, M, Figure 5—figure supplement 2A–B), the expression of both proximal and distal markers is almost completely eliminated (Figure 5A–D. Figure 5—figure supplement 1A–N). We conclude that the regulators of the D- and P-fate cooperatively promote and maintain the airway cell identity defined by trh expression. Below, we first describe roles of the embryo axis determinants on P/D-fate selection and then proceed to dissect the function of D-factors by gain-of-function approaches.
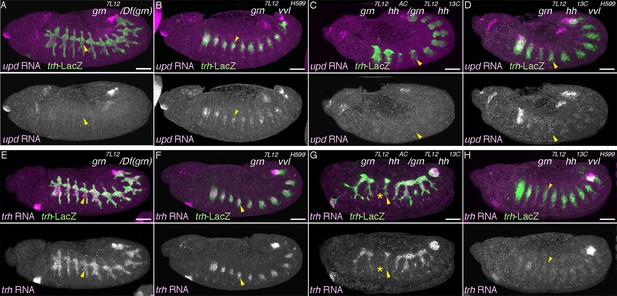
Genetic interactions of grn, hh, and vvl
Expression of the P-fate marker upd (A–D, stages 12-13) or the pan-tracheal marker trh (E–H, stages 12-13) are shown. Loss of upd expression in grn mutants (A) is partially reversed by vvl mutation (B, arrowheads) but not by hh mutation (C). In the absence of all, grn, hh, and vvl, upd expression is virtually lost (D). This accompanies severe reduction of trh expression (H, arrowheads), compared to either grn (E), grn vvl (F), or grn hh double mutants (G). Note the trh-lacZ expression in H, reflecting initial trh expression at the primordia stage. Also, expression of trh and upd in Tr1 is often detected at stage 12. Asterisks in G marks loss of trh expression in Tr3. Scale bar: 50 μm.
Wg/WNT and Dpp/BMP signaling converge on the P/D-fate selection
A key aspect of the P/D-fate selection in the Drosophila airways is the separation of the trh positive airway primordia into a D-fate circle (vvl, rho and btl positive) surrounded by a concentric P-fate ring (grn and upd positive). To investigate the origins of this rough setup, we examined the effects of Wg/WNT and Dpp/BMP (Figure 1B), two major axis determinants along the AP or DV axis, respectively (DiNardo et al., 1994; Wharton et al., 1993).
In wg/WNT mutants, expression of the D-fate markers vvl (de Celis et al., 1995), mab2A12 (Llimargas, 2000), and btl expands along the AP axis to cover most, if not all lateral parts of the embryos as a single band (Figure 6A–B, I, Figure 6—figure supplement 1B). On the other hand, expression of the P-fate markers grn, upd, and P0144-lacZ also expands along the AP axis, but this time, approximately forms two narrow stripes, dorsal and ventral, sandwiching the D-fate (Figure 6C, I, Figure 6—figure supplement 1A–B). These results suggest that Wg/WNT confines both the D- and P-fates along the AP axis (Figure 6I).
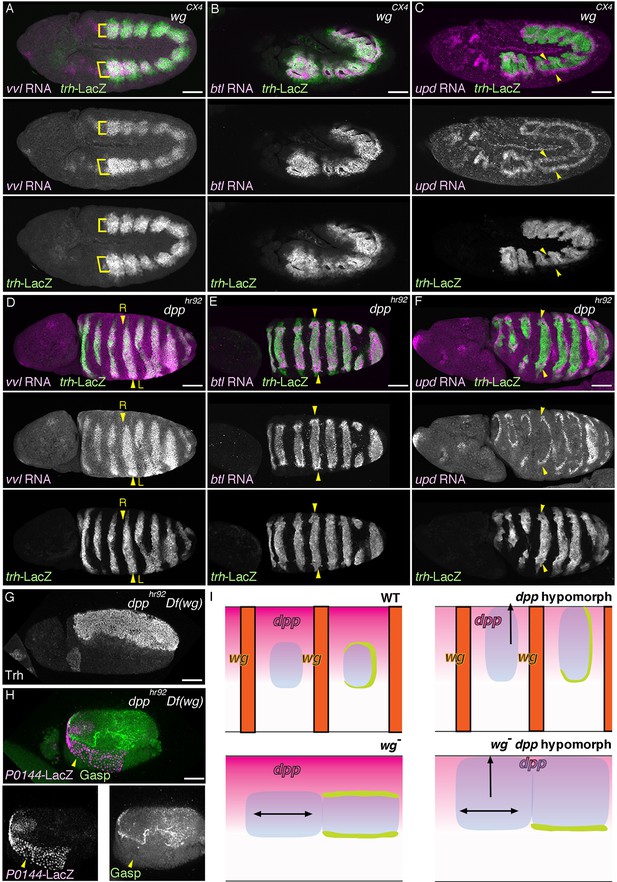
Regulation of the P/D fate selection by Wg/WNT and Dpp/BMP.
Expression regions of the D-fate markers (vvl in A, D, btl in B, E or Gasp in H) or the P-fate markers (upd in C, F or P0144-lacZ in H) are shown for wg mutants (A–C), dpp hypomorph (dpphr92) (D–F) or dpphr92 wg double mutants (H). (D-F) are dorsal views where both left (L) and right (R) sides are seen. In wg mutants, expression domains of the D-fate markers expand along the AP axis (A,B), and form a single lateral band (bracket) with occasional interruptions before the initiation of primordia invagination (A, stage 10). After invagination (B,C, stage 12), some D-fate cells remain exposed at the embryo surface to form a lateral band (B). The P-fate markers form a dorsal and a lateral band of expression at the ectoderm (C, arrowheads). In dpphr92 mutants (D-F), the airway primordia expand to the dorsal midline (D, stage 10). Concomitantly, both the D-fate (D,E) and the P-fate (F) expand to the dorsal midline. Note that the P-fate encircles the D-fate (arrowheads) and that the anterior cells often do not express the P-fate marker upd in (F, stage 11/12), probably due to the distalization by Hh that is expressed in the anterior border of each primordium. In dpphr92 Df(wg) double mutants (G,H), the whole dorsal area of the trunk/abdominal body parts become Trh positive at stage 11 (G). At stage 14/15 (H), the P-fate (arrowheads) is specified abutting the D-fate only at the ventral edge. Note that in the posterior part, the P-fate is not established as it is not established in the wild type. Scale bar: 50 μm. (I) Interpretation of data in each genotype regarding specification of the airway field (left parts) and P/D-fate selection (right parts). Expression domains of dpp and wg are colored pink and orange, respectively. In wg mutants, the airway field (light blue) expands along the AP axis (arrows) and the P-fate is established approximately as a dorsal and a ventral stripes (light green). In dpp hypomorphs (dpphr92), the airway field expands dorsally to the dorsal midline and the P-fate is established in the periphery except for the anterior and dorsal margin. In double mutants of wg and dpp hypomorph, the airway field expands both dorsally and along the AP axis, and the P-fate is only established in a ventral stripe.
The dorsal and ventral stripes of the proximal markers surrounding the expanded airways in wg/WNT mutants suggest that there are cues driving the P/D fate selection along the DV axis. Dpp/BMP presents a top candidate for such a signal (Isaac and Andrew, 1996; Wilk et al., 1996). In the absence of dpp, trh expression expands to the dorsal midline (Figure 6—figure supplement 2A, D) (Isaac and Andrew, 1996) and the cells initiate btl expression (Figure 6—figure supplement 2B). However, neither the D-fate nor the P-fate is established (Figure 6—figure supplement 2A–E), suggesting that Dpp also functions as a positive regulator of early airway development, in addition to its repressive effect on the earliest trh expression. In dpp hypomorphs (Figure 6D–F, Figure 6—figure supplement 2F–J), trh expression is expanded to the dorsal midline and P/D specification is confined near the dorsal midline, depending on the allele severities or the residual Dpp/BMP activity in the hypomorphic conditions. In the dorsally expanded primordia of dpphr92 homozygotes, expression of the D-factors vvl and btl is detected as stripes straddling the dorsal midline (Figure 6D–E), and P-fate marker expression typically encircles the entire airway primordium except for its anterior border (Figure 6F, I, Figure 6—figure supplement 2J). The loss of the P-fate markers at the anterior border in dpphr92 homozygotes could be due to the activity of the D-fate inducer Hh, which is expressed just anterior to the airway primordia (Figure 1B) (Glazer and Shilo, 2001). Consistent with the Hh-dependent anterior bias of the D-fate selection, the expression of the dorsal-distal marker h-lacZ, is lost in hh mutant (Figure 6—figure supplement 3A–B). Similarly, expression of h-lacZ is lost in rho and pnt mutants but not in btl mutants (Figure 6—figure supplement 3A, C, D). On the other hand, overactivation of Hh signaling in wg/WNT mutants expands h expression along the AP axis (Figure 6—figure supplement 1N–Q). Collectively, this analysis suggests that the graded Dpp/BMP activity controls the P/D fate selection at the dorsal border of the airway primordia. Wg/WNT signals impinge on the P/D-fate selection along the AP axis, while Hh modulates P/D differentiation from the anterior border. Consistent with the predominant roles of Dpp/BMP and Wg/WNT in airway primordia specification, in Df(wg) dpphr92 double mutants, the whole dorsal area of the trunk/abdominal parts of the embryo takes the airway cell fate (Figure 6G, I). In this situation, the dorsal part of the airway primordia takes the D-fate and only the ventral periphery takes the P fate (Figure 6H, I), supporting our model that Dpp/BMP and Wg/WNT are key regulators of the P/D fate selection.
Several other cues including inducers of the airway fate (Brown et al., 2001) are expected to contribute to the P/D fate selection. At the ventral border for example, apart from the dorso-ventral gradient of Dpp/BMP, the ventro-dorsal gradient of dEGFR signaling (Gabay et al., 1997; Golembo et al., 1996; Raz and Shilo, 1993) and another cue originating from the ventro-lateral gradient of the TF Dorsal (Anderson et al., 1985a; Roth et al., 1991; Zhao and Skeath, 2002) may orient the P/D fate selection. The exact mechanisms by which Wg/WNT, Dpp/BMP, and these other cues impinge on P/D fate selection await future research and the development of appropriate cell-specific gene inactivation methods.
Overactivation of the D-fate determinants abrogates the P-fate selection
In order to investigate how the P- and D-fates are balanced during airway cell differentiation, we analyzed the effects of overactivation of the D-fate determinants, namely RTK signaling, hh signaling and vvl. argos (aos) is a secreted negative feedback regulator of dEGFR signaling (Schweitzer et al., 1995a) while anterior open (aop) encodes an ETS TF that antagonizes RTK signaling (Rebay and Rubin, 1995). Upon activation of RTK signaling, aos is induced, while Aop is excluded from the nucleus and becomes degraded. In the airway primordia, aos expression gradually expands from the center to the periphery (Figure 7—figure supplement 1A–E). At early stage 12, both aos and Aop are preferentially detected in the proximal airways (Figure 7—figure supplement 1E–F). In aop mutants, the number of cells expressing P-fate markers is very much reduced (Figure 7A, Figure 7—figure supplement 2A). Moreover, compared to either of the single mutants (Figure 7B, Figure 7—figure supplement 2A), in aop aos double mutants, expression of the P-fate marker P0144-lacZ is virtually abolished (Figure 7C, Figure 7—figure supplement 2A). Similar phenotypes are observed upon tracheal overexpression of RasV12 (Figure 7D) or s-spi (Figure 7E) with btl-gal4 or trh-gal4 in an aos mutant background. In double mutants of aos and Gap1, a GTPase-activating protein for Ras85D (Gaul et al., 1992), expression of the P-fate markers upd and grn is variably reduced or abolished (Figure 7G,H). The expression of another P fate marker P0144-lacZ is also lost (Figure 7F, Figure 7—figure supplement 2A–B). Concomitantly, the distal mAb2A12/Gasp marker staining appears on the embryo outer surface (Figure 7E–F). These results suggest that aos, Gap1, and aop are parts of a negative regulatory mechanism balancing the D-fate inducing activity of RTK signaling to assure P-fate selection.
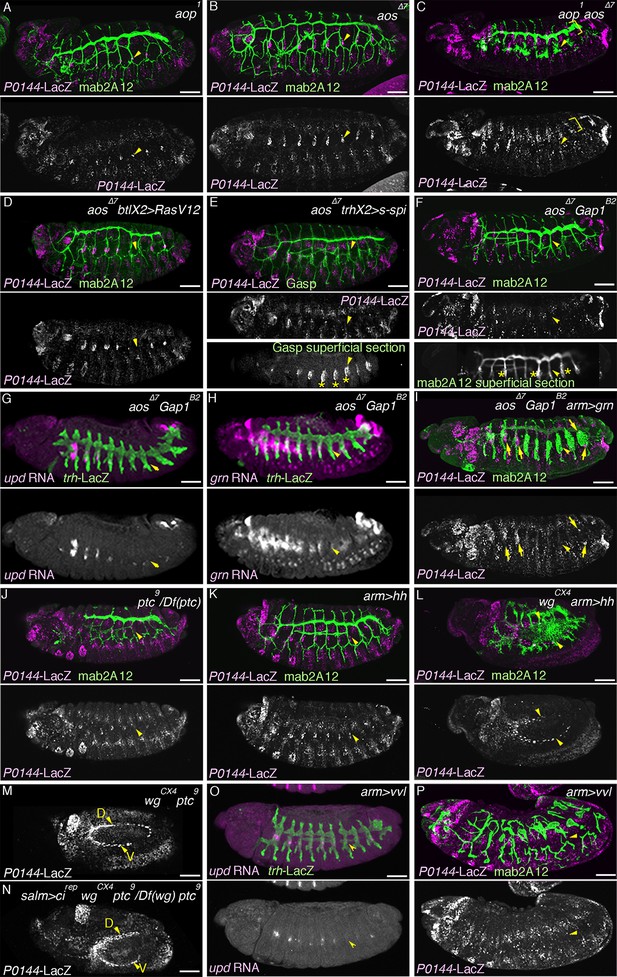
Overactivation of the D-fate determinants abrogates the P-fate specification.
(A–I) Effects of RTK signaling overactivation. Proximal areas are marked by arrowheads in Tr7. In aop mutants (A), expression of the P-fate marker P0144-lacZ is variably decreased or lost. Compared to either single mutant (A,B), in aop aos double mutants (C), P0144-lacZ expression is virtually abolished. Note that the epidermal signals in C (brackets) are from other lineages. aos mutation, combined with RasV12 overexpression by btl-gal4 (D), s-spi overexpression by trh-gal4 (E) or Gap1 mutation (F) abolish P0144-lacZ expression. Note that in E and F, after enhancement of mab2A12/Gasp signals, the epidermal surface staining is evident (asterisks). In aos Gap1 double mutants, expression of the other P-fate markers (upd in G and grn in H) is variably reduced or lost, while grn overexpression (I) partially restores P0144-lacZ expression to the proximal areas of aos Gap1 double mutants (arrows). (J–P) Effects of overactivation of hh or vvl. Overactivation of hh signaling by ptc mutation (J,M) or hh overexpression (K,L), either in the wild-type background (J,K) or wg mutant background (M,L) reduces the P0144-lacZ-positive cells. salm-gal4 mediated overexpression cirep increases the area of P0144-lacZ-positive P-fate cells in the dorsal stripe (N). The dorsal and the ventral stripes are marked with D and V in (M,N). vvl overexpression reduces or abolishes the expression region of the P-fate markers upd (O) or P0144-lacZ (P). Scale bar: 50 μm. RTK, receptor tyrosine kinase.
-
Figure 7—source data 1
Source data for Figure 7—figure supplement 2A.
The number of metameres with any number of P0144-lacZ-positive cells of the indicated genotypes at stages 15/16.
- https://doi.org/10.7554/eLife.09646.023
-
Figure 7—source data 2
Source data for Figure 7—figure supplement 2J.
The number of metameres with any number of P0144-lacZ-positive cells of the indicated genotypes at stages 15/16.
- https://doi.org/10.7554/eLife.09646.024
The loss of P0144-lacZ expression in aos Gap1 double mutants is partially suppressed by overexpression of the P fate determinant Grn (Figure 7I, Figure 7—figure supplement 2A). Reversion of the aos Gap1 mutant phenotypes is also detected by introducing vvl, hh, rho, Ras85D (Simon et al., 1991) or pnt mutations but not by btl or by single-minded (sim) mutations (Figure 7—figure supplement 2A–I). sim governs the ventral midline cell fate, which is one source of dEGFR signaling before stage 10 (Golembo et al., 1996; Mayer and Nusslein-Volhard, 1988). Thus, these results illustrate the essential roles of vvl, hh, and RTK signaling components as D-fate determinants and suggest that neither the CNS midline nor btl/dFGFR are involved in the RTK overactivation observed in the aos Gap1 double mutants. Similarly, arm-gal4 mediated, ubiquitous overexpression of secreted active spitz (s-spi) results in loss of the P-fate marker P0144-lacZ (Figure 7—figure supplement 2J–K). This defect is also suppressed by mutations of vvl, pnt, or Ras85D but not by btl, rho, or hh mutations (Figure 7—figure supplement 2J–P). This suggests that vvl has an additional essential role in dEGFR signaling other than facilitating active dEGFR ligand production. In contrast to s-spi, bnl overexpression does not abolish P0144-lacZ expression (Figure 7—figure supplement 1Q). However, simultaneous overexpression of an activated form of btl/dFGFR and its downstream mediator downstream of FGFR (dof) (Imam et al., 1999; Michelson et al., 1998; Vincent et al., 1998) reduced P0144-lacZ expression (Figure 7—figure supplement 1R) suggesting that dEGFR and btl/dFGFR share the downstream signaling pathway for P/D fate selection.
Overactivation of hh signaling, either by mutation of the inhibitory receptor ptc (Chen and Struhl, 1996; Ingham et al., 1991) or by arm-gal4-mediated hh overexpression, reduces the number of cells expressing P-fate markers (Figure 7J–K). Although overactivation of Hh signaling is expected to increase the expression of Wg/WNT (DiNardo et al., 1994), a negative regulator of trh expression (Wilk et al., 1996), reduction of the P-fate cell number upon hh overactivation still occurs in wg/WNT mutant backgrounds (Figure 7M–N). Moreover, driving a repressor form of Cubitus interruptus (Cirep), a mediator TF of Hh signaling (Hepker et al., 1997; Methot and Basler, 2001) at the dorsal ectoderm with salm-gal4 in ptc wg mutants locally increases the P-fate cell number in the dorsal side (Figure 7N). These results imply that overactivation of hh signaling autonomously represses the P-fate selection independent of its effect on wg/WNT.
Overexpression of vvl with arm-gal4, like s-spi overexpression reduces or abolishes the expression of the P-fate markers, upd or P0144-lacZ (Figure 7O–P), and this occurs independently of the presence of wg/WNT (Figure 7—figure supplement 3A–C). Collectively, the analysis indicates that expansion of the D-fate-inducing activities of RTKs, Hh or Vvl is deleterious to the P-fate selection and that the negative regulators of RTK signaling (aos, aop or Gap1) and hh signaling (ptc) ensure the selection or maintenance of the P-fate.
Differential competence of the primary branches and the TC
The hitherto analysis indicates that aos sensitizes the circuit of P/D-fate selection. We noticed that crossing of a weaker driving strain of UAS-RasV12 with arm-gal4 causes loss of P0144-lacZ expression only in the aos mutant background while the stronger UAS-RasV12strain is sufficient to eliminate P0144-lacZ expression on its own (Figure 8—figure supplement 1A–D). Using the weaker UAS-RasV12 strain, we demonstrate that within the D-fate group, the cells of the TC and the remaining primary branches have differential competence (Figure 8—figure supplement 1E). In wild-type embryos, Kni and Knrl are induced in a subset of the distal primary branches (DB, LT and GB) in response to Dpp/BMP (Chen et al., 1998; Vincent et al., 1997). arm-gal4-mediated overexpression of Dpp/BMP or the active form of its co-receptor tkvQD (Nellen et al., 1996) is sufficient to induce ectopic Kni in additional branches (Figure 8A,B) (Vincent et al., 1997). However, Kni levels in the TC cells is weaker compared to the more distal cells, suggesting that TC cells are less competent in inducing the D-fate marker Kni in response to Dpp/BMP signaling. When both UAS-dpp and the weaker line of UAS-RasV12 are simultaneously driven by arm-gal4, kni expression becomes homogenously induced in both the TC cells and the more distal cells (Figure 8C). This suggests that graded activity of the D-fate inducers, like RTKs may act to generate three different cell states along the PD axis of the airways. The most distal part (the primary branches) is established in response to the highest activity, the intermediate domain (TC) requires weaker activity, whereas the level of the D-factors needs to be low in the most proximal part (Figure 8—figure supplement 1E). Slight expansion of the P-fate only in the TC region upon Grn overexpression (Figure 4A–C) may also reflect this differential competence of TC and the remaining primary branches.
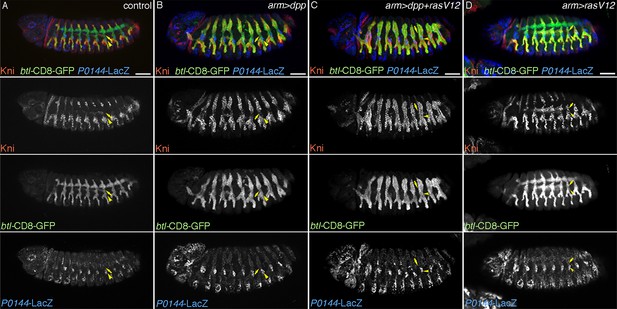
TC cells may define an intermediate fate between the more distal primary branches and the SB.
Expression of Kni is shown together with the D-fate marker btl-CD8GFP and the P-fate marker P0144-lacZ upon overexpression of dpp/BMP and/or RasV12. Arrowheads and arrows mark the P-cells and TC in Tr7, respectively. Compared to the control, dpp/BMP overexpression induces Kni expression in all the D-cells. But the Kni expression level is weaker in TC. The differential Kni expression levels in the primary branches and TC (B) become equalized by additional overexpression of RasV12 (C). Upon RasV12 overexpression alone (D), Kni expression becomes detected along the TC. Note that in all cases, P0144-lacZ-positive P-cells do not express Kni. Scale bar: 50 μm. SB, spiracular branch; TC, transverse connectives.
-
Figure 8—source data 1
Source data for Figure 8—figure supplement 1D.
The number of metameres displaying P0144-lacZ-positive cells in the indicated genotypes at stages 15/16
- https://doi.org/10.7554/eLife.09646.029
The early development of the airway primordia includes two essential aspects: first, the selection and maintenance of the airway field, and second, the selection of the P/D-fates, both of which originate from the AP and DV axis determinants of the embryo (Figure 9). More specifically, we identified a positive role of Dpp/BMP and the P/D fate factors in the establishment of the airway field. In the absence of Dpp/BMP or both P/D fate regulators, the airway field is lost. The latter phenotype could be explained in the following way in terms of P/D fate selection. Without the D-factors, the airway field is expected to uniformly take the P-fate that is promoted by grn. In this situation, grn becomes indispensable for maintenance of trh expression in the main airways. However, Wg/WNT, Hh, Dpp/BMP and other cues generate a centrally enriched expression field of the D-fate determinants vvl, rho, and btl, which then cooperate to repress the P fate, leaving the grn-trh regulation operative only in the P-region. Restoration of the P-fate in grn vvl double mutants suggests that the proximal multipotent fate is the default state for airway cells or that there is another factor that promotes the P-fate in the absence of grn and vvl.
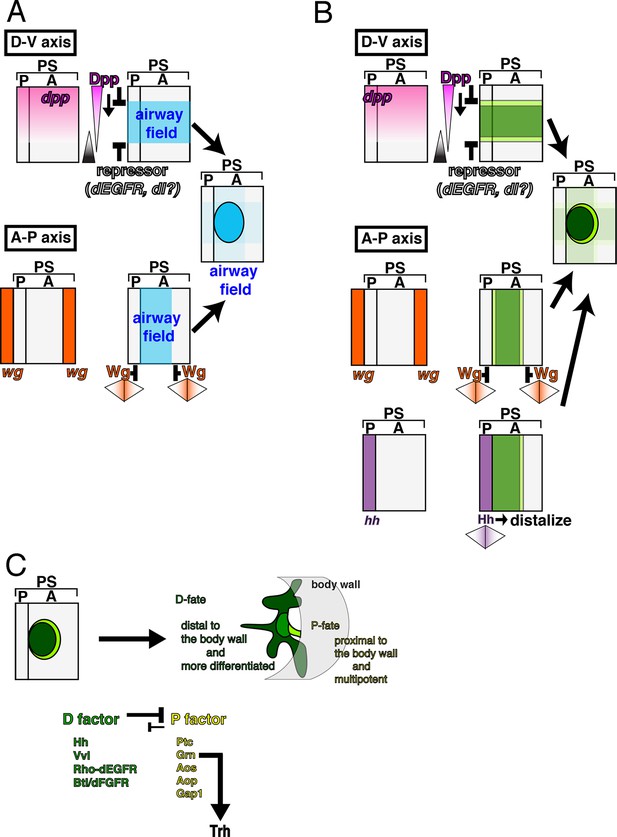
Genetic circuits explaining the early development of the Drosophila airways.
(A) Establishment of the airway field is regulated by Dpp/BMP and Wg/WNT. In each metameric unit of the Drosophila embryo (parasegment, PS) (Sanson, 2001), an airway primordium is specified just posterior to the hh expression domain (a posterior compartment of a segment, P). This is combinatorially controlled along the DV and AP axis. Dpp/BMP expressed in the dorsal region functions as both a repressor (Isaac and Andrew, 1996; Wilk et al., 1996) and an activator of the airway field (this study) while Wg/WNT functions as a repressor along the AP axis. (B) Initiation of the P/D-fate selection by Dpp/BMP, Wg/WNT, and Hh. Each airway primordium is roughly subdivided into two regions, anterior-central (D-fate, dark green) and the peripheral (P-fate, light green). The patterning cues along the AP and DV embryo axis roughly set up this radial patterning. Along the DV axis, Dpp/BMP expressed in the dorsal region functions to discriminate the P/D-fates, at least at the dorsal edge. Dpp/BMP may also discriminate the P/D-fates at the ventral edge. Alternatively, ventral cues dependent on the Dorsal TF gradient may discriminate the P/D-fates at the ventral edge. Along the AP axis, Wg/WNT expressed in transverse stripes may discriminate the P/D-fates at each edge. Hh from the anterior border stimulates the D-fate. (C) Establishing the P/D-fates by the P/D-factors. The ordered Invagination of the primordia converts the centro-peripheral patterning to the proximo-distal organization along the airway tree. By completion of invagination, D-factors establish the D-fate at the expense of the P-fate. Within the D-fate, there are two groups of cells having different responsiveness to overexpression of Dpp/BMP or Grn, e.g., TC (green) and the primary branches (dark green). See text for details. AP, anterior-posterior; DV, dorso-ventral.
Intriguingly, the Drosophila legs and the trachea are supposed to have evolved form common ancestor appendages (Franch-Marro et al., 2006). The proximo-distal patterning mechanisms of these two organs are similar in that RTK activation distalizes the field (Campbell, 2002; Galindo et al., 2002) while initiation of the distal identity is conferred by the same set of signaling molecules (Dpp/BMP, Wg/WNT, and Hh) from the outside (for the airways) or the inside (for the legs) (Estella et al., 2012). The proximal location of multipotent cells in the Drosophila airway tree resembles the conspicuous proximal localization of multipotent tracheal basal cells in the mouse lung (Rock et al., 2009; Rock et al., 2010) Given the prominent roles of FGFR signaling in branching morphogenesis in both flies (Sutherland et al., 1996) and mice (Min et al., 1998; Sekine et al., 1999), the identification of a genetic mechanism for P- and D-fate selection in flies may aid future studies aiming to identify the mechanisms that spatially confine multipotent cell selection in the embryonic vertebrate lung.
Materials and methods
Fly genetics
Request a detailed protocolFlies kept over balancer chromosomes (Lindsley et al., 1992) were grown in standard medium. We obtained the appropriate genotypes by standard genetic crosses. For overexpression of genes, we used the Gal4/UAS system (Brand and Perrimon, 1993). Germline clones of Ras85Dc40b mutants were made by FLP-DFS technique (Chou and Perrimon, 1996; Hou et al., 1995). Mutant embryos were identified by the expression of twi-lacZ, ftz-lacZ, hb-lacZ, Ubx-lacZ, or GMR-dfd-GFP constructs inserted on balancer chromosomes. We identified mutants harboring dpp mutations by selecting embryos with previously reported phenotypes in the embryo DV patterning. For collection of large numbers of virgins, we used a Y chromosome harboring hs-hid construct developed by R. Lehmann and M. Van Doren (Starz-Gaiano et al., 2001). See Flybase (St Pierre et al., 2014) for details of strains described below.
Mutant strains; AbdBM1 (a gift from I. Lohmann) (Lohmann et al., 2002), btl∆oh10 and btl∆oh24-1(Ohshiro and Saigo, 1997), Df(os)1A (a gift from D. Harrison) (Harrison et al., 1998), Gap1B2 (a gift from N. Perrimon) (Hou et al., 1995), grn7L12 (a gift from J. Hombria) (Brown and Castelli-Gair Hombria, 2000), hh13C (Hosono et al., 2003), Ras85D∆c40b (a gift from N. Perrimon and C. A. Berg) (Hou et al., 1995; Schnorr and Berg, 1996), rho∆38 (a gift from D. Andrew) (Bradley and Andrew, 2001), rho7M (a gift from J. Skeath) (Skeath, 1998), topf24 (a gift from K. Moses) (Kumar et al., 1998) utH599 = vvlH599 (a gift from A. Salzberg) (Inbal et al., 2003). arm4 was obtained from National Institute of Genetics (NIG), Mishima, Japan. aop1, aos∆7, bnlP1, Df(3R)Dl-BX12 as Df(bnl), dpphr92, dppH46, pnt∆88, Df(H99), hhAC, pnt2, ptc9, Df(2R)Exel7098 as Df(ptc), sim2, topf2, trh2, Df(3L)Exel6109 as Df(vvl), wgCX4, Df(2L)Exel6017 as Df(wg) were obtained from Bloomington stock center (BDSC), Indiana.
Enhancer trap strains; 1-eve-1 as trh-lacZ (a gift from N. Perrimon) (Perrimon et al., 1991) and P0144-lacZ (a gift from W. Janning, Flyview), pnt-lacZ (a gift from M. Krasnow) (Samakovlis et al., 1996a). h-lacZ was obtained from BDSC.
Enhancer reporter strains; btl-CD8-GFP (a gift from M. Sato) (Sato and Kornberg, 2002), kni-(dpp)-lacZ (Chen et al., 1998), and salm-TSE-lacZ (Kuhnlein and Schuh, 1996) (gifts from R. Schuh).
Gal4 and UAS strains; btl-gal4 and trh66-gal4 on sencond and third chromosomes (gifts from S. Hayashi) (Kondo and Hayashi, 2013; Shiga et al., 1996), salm-gal4 (a gift from M. Llimargas) (Llimargas, 2000), UAS-bnl (a gift from M. Krasnow) (Sutherland et al., 1996), UAS-btl/dFGFR (R. Matsuda and K. Saigo), UAS-ci75 (a gift from S. Ishii) (Dai et al., 2003), UAS-cirep (a gift from A. Moore) (Karim and Moore, 2011), UAS-DIAP (a gift from E. Kuranaga and M. Miura) (Kuranaga et al., 2002), UAS-dof (a gift from M. Affolter) (Vincent et al., 1998), UAS-dpp (a gift from K. Basler) (Ruberte et al., 1995), UAS-grn (a gift from J. Hombria) (Brown and Castelli-Gair Hombria, 2000), UAS-hh (Hosono et al., 2003), UAS-torso4021-btl (a gift from E. Hafen) (Feldmann et al., 1999), UAS-RasV12 (gifts from G. M. Rubin) (Karim and Rubin, 1998), UAS-sspi (a gift from B. Shilo) (Schweitzer et al., 1995b), UAS-vvl and UAS-vvl vvlH599 (gifts from A. Salzberg) (Inbal et al., 2003). arm-gal4, UAS-dEGFR and UAS-nGFP were obtained from BDSC.
In situ hybridization and immunostaining
Request a detailed protocolEggs were collected on apple/grape juice plates at 25°C. Embryos were bleached and fixed as previously described (Patel, 1994) for 15–30 min with a 1:1 mixture of heptane and a fix solution (3.7% formaldehyde, 0.1 M Hepes pH6.9, 2 mM MgSO4). Embryos were dechorionated with methanol and incubated in 0.1% PBT supplemented with 0.5% BSA. Staging of embryos was done as previously described (Campos-Ortega and Hartenstein, 1997).
For immunostaining, the following primary antibodies were used. Guinea-pig anti-Gasp (1:1000) (Tiklova et al., 2013), Guinea-pig anti-Kni (1:300), (developed by J. Reinitz and distributed by Y. Hiromi, East Asian Segmentation Antibody Center, Mishima, Japan) (Kosman et al., 1998), rabbit anti-Trh (1:50). Mouse anti-Abd-B (1:10, donated by S. Celniker) (Celniker et al., 1989), mouse anti-Aop (1:10, donated by I. Rebay and G. M. Rubin) (Rebay and Rubin, 1995), mouse Dcad (1:10, donated by T. Uemura) (Oda et al., 1994), mouse mab2A12 (anti-Gasp) (1:5, donated by M. Krasnow, N. Patel and C. Goodman) (Samakovlis et al., 1996b; Tiklova et al., 2013) were obtained from Developmental Studies Hybridoma Bank (DSHB), Iowa. Commercially available antibodies were anti-LacZ (E. coli. β-Galactosidase) antibodies made in goat (1:500, Biogenesis, UK) or rabbit (1:1000, Cappel, Netherlands) and anti-GFP antibodies made in rabbit (1:500, JL-8 Clontech, Mountain View, CA) or mouse (1:1000, GFP20 Sigma, St. Louis, MO).
Donkey or goat biotin- or fluorescently labeled secondary antibodies made against the host species of primary antibodies were purchased from Jackson Laboratories, Sacramento, CA. Streptavidin coupled with AMCA, FITC, or Cy5 were used when necessary. For mab2A12 detection TSA amplification (PerkinElmer, Waltham, MA) was used. For detection of apoptosis, a TUNEL kit from Roche (Switzerland) was used.
Double fluorescent labeling with RNA probes and antibodies was carried out as described (Goto and Hayashi, 1997). The following cDNA clones were used to make hybridization probes; btl (Ohshiro and Saigo, 1997), h (a gift from D. Ish-Horowicz) (Hooper et al., 1989), grn (a gift from J. Hombria) (Brown and Castelli-Gair Hombria, 2000), pnt (a gift from C. Klambt) (Klambt, 1993), salm (a gift from R. Schuh) (Kuhnlein and Schuh, 1996), upd (a gift from D. Harrison) (Harrison et al., 1998), vvl (a gift from J. Casanova) (Llimargas and Casanova, 1997). DNA clones of aos, rho and trh were obtained from Drosophila Genomics Resource Center (DGRC), Indiana, USA.
Confocal images were taken by Bio-Rad (Hercules, CA) MRC1024, Olympus (Japan) Fluoview 1000 or Zeiss (Germany) LSM780. Images of controls and mutants taken by the same confocal microscopes were used for comparison. Images were processed by ImageJ and figures were prepared with Adobe Photoshop and Illustrator.
References
-
Multiple requirements for the receptor serine/threonine kinase thick veins reveal novel functions of TGF beta homologs during drosophila embryogenesisDevelopment 120:3105–3117.
-
Function of the drosophila POU domain transcription factor drifter as an upstream regulator of breathless receptor tyrosine kinase expression in developing tracheaDevelopment 122:4169–4178.
-
Genetic control of epithelial tube size in the drosophila tracheal systemDevelopment 127:3271–3282.
-
Searching for pattern and mutation in the drosophila genome with a p-lacZ vectorGenes & Development 3:1273–1287.https://doi.org/10.1101/gad.3.9.1273
-
Ribbon encodes a novel BTB/POZ protein required for directed cell migration in drosophila melanogasterDevelopment 128:3001–3015.
-
Targeted gene expression as a means of altering cell fates and generating dominant phenotypesDevelopment 118:401–415.
-
Drosophila grain encodes a GATA transcription factor required for cell rearrangement during morphogenesisDevelopment 127:4867–4876.
-
Cellular and molecular mechanisms involved in branching morphogenesis of the drosophila tracheal systemJournal of Applied Physiology 97:2347–2353.https://doi.org/10.1152/japplphysiol.00435.2004
-
BookThe Embryonic Development of Drosophila MelanogasterBerlin, Heidelberg: Springer Berlin Heidelberg.https://doi.org/10.1007/978-3-662-02454-6
-
BookThe Embryonic Development of Drosophila Melanogaster (2nd edn)Berlin, Heidelberg: Springer Berlin Heidelberg.https://doi.org/10.1007/978-3-662-22489-2
-
The transcription factors KNIRPS and KNIRPS RELATED control cell migration and branch morphogenesis during drosophila tracheal developmentDevelopment 125:4959–4968.
-
The autosomal FLP-DFS technique for generating germline mosaics in drosophila melanogasterGenetics 144:1673–1679.
-
Trachealess (trh) regulates all tracheal genes during drosophila embryogenesisDevelopmental Biology 360:160–172.https://doi.org/10.1016/j.ydbio.2011.09.014
-
Ventral veinless, the gene encoding the Cf1a transcription factor, links positional information and cell differentiation during embryonic and imaginal development in drosophila melanogasterDevelopment 121:3405–3416.
-
The making of a maggot: patterning the drosophila embryonic epidermisCurrent Opinion in Genetics & Development 4:529–534.https://doi.org/10.1016/0959-437X(94)90068-E
-
A dynamic network of morphogens and transcription factors patterns the fly legCurrent Topics in Developmental Biology 98:173–198.https://doi.org/10.1016/B978-0-12-386499-4.00007-0
-
Control of growth and differentiation by drosophila RasGAP, a homolog of p120 ras–GTPase-activating proteinMolecular and Cellular Biology 19:1928–1937.https://doi.org/10.1128/MCB.19.3.1928
-
MAP kinase in situ activation atlas during drosophila embryogenesisDevelopment 124:3535–3541.
-
The drosophila embryonic midline is the site of spitz processing, and induces activation of the EGF receptor in the ventral ectodermDevelopment 122:3363–3370.
-
Specification of the embryonic limb primordium by graded activity of decapentaplegicDevelopment 124:125–132.
-
Organ renewal and cell divisions by differentiated cells in drosophilaProceedings of the National Academy of Sciences of the United States of America 105:10832–10836.https://doi.org/10.1073/pnas.0805111105
-
Drosophila unpaired encodes a secreted protein that activates the JAK signaling pathwayGenes & Development 12:3252–3263.https://doi.org/10.1101/gad.12.20.3252
-
Drosophila cubitus interruptus forms a negative feedback loop with patched and regulates expression of hedgehog target genesDevelopment 124:549–558.
-
Spatial control of hairy protein expression during embryogenesisDevelopment 107:489–504.
-
Functional subdivision of trunk visceral mesoderm parasegments in drosophila is required for gut and trachea developmentDevelopment 130:439–449.
-
Stumps, a drosophila gene required for fibroblast growth factor (fGF)-directed migrations of tracheal and mesodermal cellsGenetics 152:307–318.
-
Tubulogenesis in drosophila: a requirement for the trachealess gene productGenes & Development 10:103–117.https://doi.org/10.1101/gad.10.1.103
-
Ectopic expression of activated Ras1 induces hyperplastic growth and increased cell death in drosophila imaginal tissuesDevelopment 125:1–9.
-
Convergent local identity and topographic projection of sensory neuronsThe Journal of Neuroscience 31:17017–17027.https://doi.org/10.1523/JNEUROSCI.2815-11.2011
-
The drosophila gene pointed encodes two ETS-like proteins which are involved in the development of the midline glial cellsDevelopment 117:163–176.
-
Rapid preparation of a panel of polyclonal antibodies to drosophila segmentation proteinsDevelopment Genes and Evolution 208:290–294.https://doi.org/10.1007/s004270050184
-
Dual function of the region-specific homeotic gene spalt during drosophila tracheal system developmentDevelopment 122:2215–2223.
-
Dissecting the roles of the drosophila EGF receptor in eye development and MAP kinase activationDevelopment 125:3875–3885.
-
Ventral veinless, a POU domain transcription factor, regulates different transduction pathways required for tracheal branching in drosophilaDevelopment 124:3273–3281.
-
EGF signalling regulates cell invagination as well as cell migration during formation of tracheal system in drosophilaDevelopment Genes and Evolution 209:174–179.https://doi.org/10.1007/s004270050241
-
Wingless and its signalling pathway have common and separable functions during tracheal developmentDevelopment 127:4407–4417.
-
Seven wnt homologues in drosophila: a case study of the developing tracheaeProceedings of the National Academy of Sciences of the United States of America 98:14487–14492.https://doi.org/10.1073/pnas.251304398
-
BookThe Development of Drosophila MelanogasterPlainview, NY: Cold Spring Harbor Laboratory Press.
-
An absolute requirement for cubitus interruptus in hedgehog signalingDevelopment 128:733–742.
-
Heartbroken is a specific downstream mediator of FGF receptor signalling in drosophilaDevelopment 125:4379–4389.
-
Beyond the niche: tissue-level coordination of stem cell dynamicsAnnual Review of Cell and Developmental Biology 29:107–136.https://doi.org/10.1146/annurev-cellbio-101512-122319
-
Transcriptional regulation of breathless FGF receptor gene by binding of TRACHEALESS/dARNT heterodimers to three central midline elements in drosophila developing tracheaDevelopment 124:3975–3986.
-
Ligand-dependent activation of breathless FGF receptor gene in drosophila developing tracheaMechanisms of Development 114:3–11.https://doi.org/10.1016/S0925-4773(02)00042-4
-
Generating lineage-specific markers to study drosophila developmentDevelopmental Genetics 12:238–252.https://doi.org/10.1002/dvg.1020120309
-
Basal cells as stem cells of the mouse trachea and human airway epitheliumProceedings of the National Academy of Sciences of the United States of America 106:12771–12775.https://doi.org/10.1073/pnas.0906850106
-
Airway basal stem cells: a perspective on their roles in epithelial homeostasis and remodelingDisease Models & Mechanisms 3:545–556.https://doi.org/10.1242/dmm.006031
-
Cactus, a maternal gene required for proper formation of the dorsoventral morphogen gradient in drosophila embryosDevelopment 112:371–388.
-
Development of the drosophila tracheal system occurs by a series of morphologically distinct but genetically coupled branching eventsDevelopment 122:1395–1407.
-
Genetic control of epithelial tube fusion during drosophila tracheal developmentDevelopment 122:3531–3536.
-
Differential activity of Ras1 during patterning of the drosophila dorsoventral axisGenetics 144:1545–1557.
-
Fgf10 is essential for limb and lung formationNature Genetics 21:138–141.https://doi.org/10.1038/5096
-
A nuclear GFP/beta-galactosidase fusion protein as a marker for morphogenesis in living drosophilaDevelopment, Growth and Differentiation 38:99–106.https://doi.org/10.1046/j.1440-169X.1996.00012.x
-
The drosophila EGF receptor controls the formation and specification of neuroblasts along the dorsal-ventral axis of the drosophila embryoDevelopment 125:3301–3312.
-
FlyBase 102--advanced approaches to interrogating FlyBaseNucleic Acids Research 42:D780–D788.https://doi.org/10.1093/nar/gkt1092
-
Spatially restricted activity of a drosophila lipid phosphatase guides migrating germ cellsDevelopment 128:983–991.
-
Scanning electron microscopy of drosophila melanogaster embryogenesisDevelopmental Biology 57:403–416.https://doi.org/10.1016/0012-1606(77)90225-1
-
DPP controls tracheal cell migration along the dorsoventral body axis of the drosophila embryoDevelopment 124:2741–2750.
-
Interactions between the EGF receptor and DPP pathways establish distinct cell fates in the tracheal placodesDevelopment 124:4707–4716.
-
An activity gradient of decapentaplegic is necessary for the specification of dorsal pattern elements in the drosophila embryoDevelopment 117:807–822.
-
The sox-domain containing gene dichaete/fish-hook acts in concert with vnd and ind to regulate cell fate in the drosophila neuroectodermDevelopment 129:1165–1174.
Article and author information
Author details
Funding
Ministry of Education, Culture, Sports, Science, and Technology
- Kaoru Saigo
Vetenskapsrådet
- Christos Samakovlis
Cancerfonden
- Christos Samakovlis
The funders had no role in study design, data collection and interpretation, or the decision to submit the work for publication.
Acknowledgements
We thank the members of the fly community who isolated, characterized or distributed fly strains, antibodies or DNA clones. Especially, we thank M Affolter, D Andrew, K Basler, CA Berg, J Casanova, E Hafen, D Harrison, S Hayashi, Y Hiromi, J Hombria, D Ish-Horowicz, S Ishii, W Janning, C Klambt, M Krasnow, E Kuranaga, M Llimargas, M Miura, A Moore, K Moses, N Perrimon, GM Rubin, A Salzberg, M Sato, R Schuh, B Shilo, J Skeath, R Ueda, BDSC, DGRC and NIG for directly sharing fly strains and DNA clones. We thank Flybase for the Drosophila genomic resources. We thank the Stockholm University Imaging Facility and members of the Mannervik, Saigo, Samakovlis and Åström laboratories for support during the project, especially M Björk for fly service and V Tsarouhas for continuous support. Special thanks to Y Emori and F Ui-Tei for help in maintaining fly strains after the retirement of KS and to J Muhr for critical comments on the manuscript.
Copyright
© 2015, Matsuda et al.
This article is distributed under the terms of the Creative Commons Attribution License, which permits unrestricted use and redistribution provided that the original author and source are credited.
Metrics
-
- 4
- citations
Views, downloads and citations are aggregated across all versions of this paper published by eLife.
Citations by DOI
-
- 4
- citations for umbrella DOI https://doi.org/10.7554/eLife.09646