Paternally expressed imprinted genes establish postzygotic hybridization barriers in Arabidopsis thaliana
Abstract
Genomic imprinting is an epigenetic phenomenon causing parent-of-origin specific differential expression of maternally and paternally inherited alleles. While many imprinted genes have been identified in plants, the functional roles of most of them are unknown. In this study, we systematically examine the functional requirement of paternally expressed imprinted genes (PEGs) during seed development in Arabidopsis thaliana. While none of the 15 analyzed peg mutants has qualitative or quantitative abnormalities of seed development, we identify three PEGs that establish postzygotic hybridization barriers in the endosperm, revealing that PEGs have a major role as speciation genes in plants. Our work reveals that a subset of PEGs maintains functional roles in the inbreeding plant Arabidopsis that become evident upon deregulated expression.
https://doi.org/10.7554/eLife.10074.001eLife digest
When plants and animals reproduce sexually, their offspring inherit two copies of every gene, one from each parent, which are arranged in two sets of structures called chromosomes. In some tissues, one gene copy may be switched off—through a process called ‘genomic imprinting’—while the other copy remains active. In plants, genomic imprinting is vital for seeds to develop normally. It is particularly important in the tissue that provides nutrients for the growing embryo (the endosperm), in which one of the copies of many genes are switched off. Genes inherited from the male parent that have been imprinted are known as paternally expressed imprinted genes (PEGs).
Unlike most animals, it is common for plants to have more than two sets of chromosomes. When plants with different numbers of chromosome sets cross-fertilize each other, their offspring may have three copies of every gene instead of two. These ‘triploid’ seeds often die because their endosperm fails to develop normally. This is due to the increased activity of imprinted genes, which causes changes in the activity of many other genes in the endosperm. Although it is known that genomic imprinting in the endosperm helps to establish this reproductive barrier, it is not clear what specific roles many of the imprinted genes play.
Here, Wolff et al. switched off several different PEGs in the plant Arabidopsis to investigate how they affect seed development. The experiments show that in seeds that have the normal two copies of every gene, inactivating these imprinted genes does not affect seed development. However, in triploid seeds, inactivating three of the imprinted genes rescues seeds that would normally die. These genes encode proteins that activate pathways in the endosperm that promote the formation of cell walls, which is a crucial stage in seed development.
Wolff et al.'s findings reveal how imprinted genes in the endosperm establish a barrier to reproduction by preventing seeds produced from crosses between plants with different numbers of chromosome sets from being able to survive. Reproductive barriers are a major obstacle in plant breeding, so understanding how these barriers form may open new avenues for developing new plant varieties.
https://doi.org/10.7554/eLife.10074.002Introduction
Genomic imprinting is an epigenetic phenomenon occurring in mammals and flowering plants that leads to parent-of-origin specific differential expression of maternally and paternally inherited alleles (Gehring, 2013). Recent screening of the seed transcriptome in various plant species revealed dozens to several hundreds of novel candidate imprinted genes in maize, rice, castor bean, and Arabidopsis thaliana (Gehring et al., 2011; Hsieh et al., 2011; Luo et al., 2011; Waters et al., 2011; Wolff et al., 2011; Pignatta et al., 2014; Xu et al., 2014). While few reports demonstrate genes to be temporally imprinted in the plant embryo (Jahnke and Scholten, 2009; Raissig et al., 2013), the vast majority of imprinted genes has been observed in the endosperm, the ephemeral triploid tissue derived after fertilization of the diploid central cell with a haploid sperm cell. In most angiosperms the endosperm initially develops as a syncytium and cellularizes after a defined number of mitotic divisions (Li and Berger, 2012). The right timing of endosperm cellularization is crucial for proper seed development, its failure results in deficient nutrient supply, which causes embryo arrest and eventually seed abortion (Hehenberger et al., 2012). In Arabidopsis, endosperm cellularization is regulated by, among others, the type I MADS-box transcription factor AGL62. Loss of AGL62 leads to precocious endosperm cellularization (Kang et al., 2008), whereas increased AGL62 expression correlates with delayed or failed cellularization (Erilova et al., 2009; Tiwari et al., 2010). Similar effects on endosperm development have been observed in response to interploidy hybridizations. While maternal excess hybridizations cause precocious endosperm cellularization and reduced seed size, the reciprocal cross leads to endosperm cellularization failure and seed abortion in an accession-dependent frequency (Scott et al., 1998; Dilkes et al., 2008). This phenomenon establishes a postzygotic reproductive barrier by preventing the formation of viable triploid seeds and has been termed ‘triploid block’ (Marks, 1966). Dosage-sensitivity of the endosperm has been proposed to be a consequence of deregulated imprinted genes that are responsible for interploidy hybridization failure (Haig and Westoby, 1989; Gutierrez-Marcos et al., 2003; Kinoshita, 2007). Indeed, in response to interploidy hybridizations many imprinted genes are deregulated (Jullien and Berger, 2010; Tiwari et al., 2010; Wolff et al., 2011) and the paternally expressed imprinted gene ADMETOS (ADM) has been identified to be a causative gene responsible for abortion of triploid seeds upon paternal excess hybridizations in Arabidopsis (Kradolfer et al., 2013). While the identification of ADM provided first evidence that imprinted genes can establish reproductive barriers, the question whether this is a more general phenomenon applying to other imprinted genes as well, remained unresolved.
In this study we investigated the functional role of 15 PEGs during seed development in Arabidopsis. None of the analyzed peg mutants caused qualitative or quantitative abnormalities of diploid seed development, revealing that many PEGs do either not have an important functional role in Arabidopsis seeds or act redundantly with non-imprinted genes. However, 3 out of ten tested peg mutants rescued triploid seed abortion, uncovering a major role of PEGs in establishing postzygotic interploidy hybridization barriers.
Results and discussion
Impaired PEG function does not impact on diploid seed development
Genomic imprinting has been proposed to have a major impact on seed development (Haig and Westoby, 1989). We tested this hypothesis by investigating whether loss of PEG function would negatively impact on seed development and viability. We examined 15 PEGs that were shown to be imprinted at 4 days after pollination (DAP) in reciprocal crosses between Col and Bur-0 accessions (Wolff et al., 2011). While At2g36560 (PEG5), At4g05470 (PEG8), At1g67830 (FXG1), At1g17770 (SUVH7), At1g57800 (VIM5) and AT1g48910 (YUC10) were also identified to be imprinted in Col and Ler accessions, At1g11810 (PEG1), At1g49290 (PEG2), At1g60400 (PEG3), At1g66630 (PEG4), At3g49770 (PEG6), At3g50720 (PEG7), At5g15140 (PEG9), At1g34650 (HDG10) and At4g31900 (PKR2) were not identified as being imprinted in Col and Ler accessions by other studies (Gehring et al., 2011; Hsieh et al., 2011; Pignatta et al., 2014). Due to the lack of small nucleotide polymorphisms (SNPs) for PEG1, PEG9, and PKR2 we only tested the imprinting status of the 6 remaining PEGs in reciprocal crosses of Col and Ler accessions at 4 DAP. Parent-of-origin specific expression could be detected for all the genes tested (Figure 1—figure supplement 1), revealing that all tested PEGs are consistently imprinted in different accessions.
To analyze the functional role of PEGs during seed development, we obtained T-DNA insertion mutants for all genes (Figure 1—figure supplement 2). We tested the mRNA levels in all mutants that were not yet previously investigated and found them strongly reduced compared to wild type (Figure 1—figure supplement 3). Nevertheless, none of the analyzed mutants had increased levels of unfertilized ovules or seed abortion compared to wild-type plants (Figure 1A) and neither transmission through the male gametophyte was significantly impaired (Chi–Square test, 1:1 segregation hypothesis; p > 0.3) (Figure 1—figure supplement 4). We investigated the possibility that PEGs have a positive impact on seed size by pollinating wild-type plants with pollen from heterozygous peg mutants and analyzed the size of mature seeds. This analysis revealed that there was no significant (F-test; α = 0.01) difference between wild-type and peg/+ mutant seeds (Figure 1B). We furthermore tested the possibility whether PEG function could have a more prominent effect when fitness of the maternal parent was compromised. We completely removed rosette leaves of Col mother plants 2 days prior to emasculation and repeated the crosses with peg/+ mutants. In agreement with a previous report (Akiyama and Agren, 2012), substantial loss of source tissue caused a reduction of seed number in the majority of crosses (Figure 1—figure supplement 5). Nevertheless, there was no significant (F-test; α = 0.01) difference in seed size between wild-type and peg/+ mutant seeds (Figure 1—figure supplement 6), revealing that there is no general, non-redundant role of PEGs in controlling seed development and seed size in Arabidopsis.
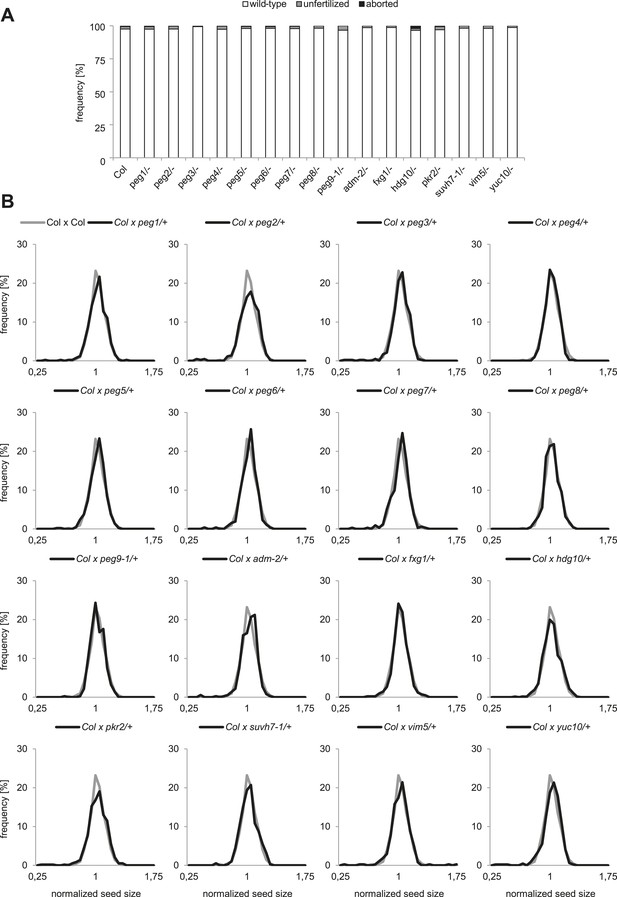
Impact of PEG function on diploid seed development.
(A) Percentage of normal, unfertilized, and aborted seeds from self-fertilized wild-type and homozygous peg mutant plants. A minimum of 300 seeds was analyzed for each genotype. (B) Size measurements of mature seeds derived from crosses of maternal Col plants pollinated with heterozygous peg pollen (black line) were normalized, plotted on a histogram and distribution was compared with wild type control crosses (grey line). A minimum of 400 seeds was analyzed for each cross.
PEGs establish postzygotic hybridization barriers
We tested the hypothesis that in addition to ADM other PEGs are involved in building the triploid block. We randomly selected 10 PEGs that were, with the exception of VIM5, strongly up-regulated in triploid seeds (Figure 2—figure supplement 1), and generated double mutants with the omission of second division1 (osd1) mutant. The osd1 mutation causes the formation of unreduced (2n) male gametes at a frequency of almost 100% (d'Erfurth et al., 2009). Therefore, using osd1 as pollen donor leads to the formation of almost 100% triploid seeds. We used pollen from plants homozygous for both mutations for crosses with wild-type Col mothers. Strikingly, 3 out of ten peg mutants were able to rescue triploid seed abortion; while crosses of Col x osd1 gave rise to 8% non-collapsed seeds, crosses of Col with suvh7 osd1, peg2 osd1 and peg9 osd1 increased levels of non-collapsed seeds to 53%, 86% and 54%, respectively (Figure 2A). The majority of non-collapsed triploid seeds germinated (Figure 2A) and developed into viable seedlings (Figure 2B). Independent mutant alleles for suvh7 and peg9 introduced into the osd1 background caused a similar rescue effect on triploid seeds (Figure 2—figure supplement 2). As for peg2 no second mutant allele could be identified, we complemented the mutant phenotype with a genomic PEG2::PEG2 construct that restored triploid seed inviability, revealing that the peg2 phenotype is indeed caused by failure of PEG2 function (Figure 2—figure supplement 2). To test whether maternal loss of PEG function would impact on triploid seed rescue, we pollinated either osd1 or peg osd1 mutant pollen onto maternal plants mutant for the corresponding peg. However, no maternal effect on triploid seed rescue could be detected (Figure 2C), revealing no impact of the maternal alleles of SUVH7, PEG2 and PEG9 on the triploid block. Consistently, SUVH7 and PEG2 remained imprinted in triploid seeds (Figure 2D). PEG9 has no polymorphism between Col and Ler accessions and could not be tested.
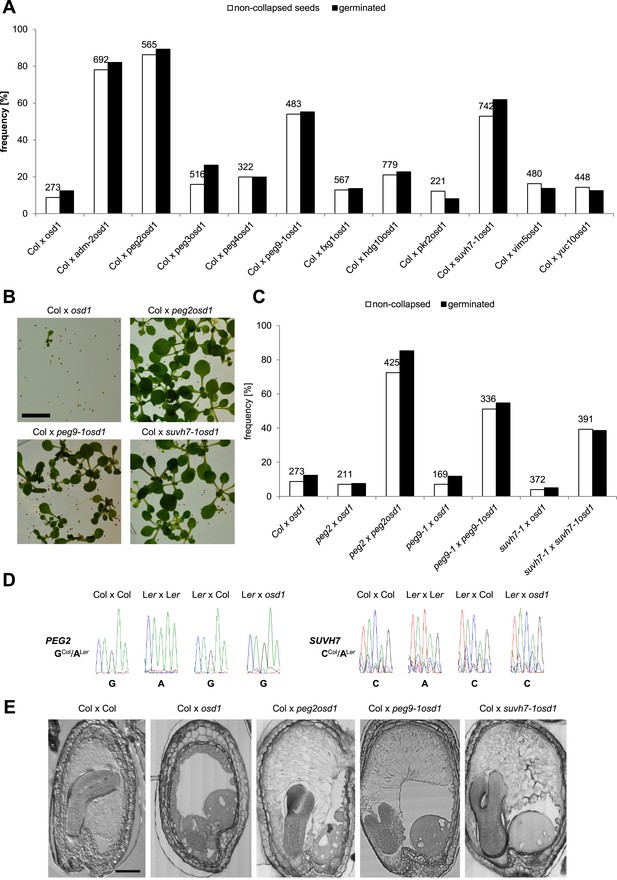
PEGs establish interploidy hybridization barriers.
(A) Percentages of non-collapsed and germinated seeds of wild-type plants pollinated with osd1 and peg osd1 pollen. Numbers on top of bars correspond to number of analyzed seeds. (B) Triploid seedlings 14 days after germination. Scale bar, 1 cm. (C) Percentages of non-collapsed and germinated seeds of peg mutant plants pollinated with osd1 and peg osd1 pollen. Numbers on top of bars correspond to number of analyzed seeds. (D) PEG2 and SUVH7 remain imprinted in diploid and triploid seeds. Siliques of crosses of Ler plants pollinated with Col or osd1 pollen were harvested at 4 DAP and imprinted expression was tested by PCR and subsequent DNA sequencing. Siliques of Col and Ler plants pollinated with Col and Ler pollen were used as controls. (E) Sections of seeds derived from crosses of Col plants pollinated with Col, osd1, peg2 osd1, peg9-1 osd1 and suvh7-1 osd1 pollen at 8 DAP. Scale bar, 0.1 mm.
Rescue of triploid seed development by peg mutants occurs by different mechanisms
SUVH7 encodes for a putative histone-lysine N-methyltransferase that however did not have histone methyltransferase activity against H3K9 in in vitro assays (Ebbs and Bender, 2006). PEG2 encodes for an unknown protein with no predicted structural domains, while PEG9 encodes for a galactose mutarotase, which catalyzes the first step of the Leloir pathway, the conversion of beta-D-galactose to alpha-D-galactose (Holden et al., 2003). Galactose is a major constituent of the Arabidopsis endosperm cell wall in the form of pectic homogalacturan (Lee et al., 2012) and altered PEG9 activity could impact on cell wall composition. We tested whether rescue of triploid seed viability by suvh7, peg2 and peg9 was associated with restored endosperm cellularization. While the endosperm of triploid seeds was completely uncellularized at 8 DAP, endosperm cellularization in triploid suvh7, peg2 and peg9 seeds was almost complete at 8 DAP; only the over-proliferated chalazal cyst remained uncellularized (Figure 2E). Failure of endosperm cellularization is responsible for embryo arrest (Hehenberger et al., 2012). Consistently, embryos of peg2, peg9, and suvh7 triploid seeds developed, albeit delayed compared to wild-type diploid embryos (Figure 2E).
Triploid seed rescue by adm is associated with strongly decreased mRNA levels of type I AGAMOUS-LIKE MADS-box genes (AGLs) as well as PEGs (Kradolfer et al., 2013). To test whether triploid rescue is generally connected with decreased expression of AGLs and PEGs, we generated whole-genome transcriptome data of seeds from triploid adm, suvh7 and peg2 mutants and analyzed expression of AGLs and PEGs that had increased mRNA levels in triploid seeds. Triploid seed rescue by adm and suvh7 was associated with a similar decrease of mRNA levels of many AGLs and PEGs (Figure 3A,B), suggesting that ADM and SUVH7 share a common mode of action. Strikingly, while peg2 had the strongest effect on triploid seed rescue (Figure 2A), the effect on gene expression was weakest among the three mutants. In particular, AGLs and PEGs that were strongly affected in adm and suvh7 triploid seeds were only weakly affected in peg2 triploid seeds (Figure 3A,B). This suggests that peg2-mediated triploid seed rescue occurs independently of normalized AGL and PEG expression and may affect a pathway downstream of either AGLs or PEGs. In support of this view, most genes down-regulated in triploid peg2 seeds were similarly down-regulated in triploid adm and suvh7 seeds (Figure 3C, Figure 3—source data 1), suggesting that all three mutants affect a common downstream pathway. Genes commonly down-regulated in all three mutants were significantly enriched for genes involved in carbohydrate metabolism and in particular genes encoding for polygalacturonases (Figure 3—figure supplement 1). Polygalacturonan is the backbone of the major primary cell wall component pectin, which is degraded by polygalacturonases (Atmodjo et al., 2013). Pectin degradation is assumed to be a key step in the deconstruction of plant cell walls (Xiao et al., 2014), therefore suppression of pectin hydrolysis in adm, suvh7, peg2, and peg9 may be the key mechanism to induce endosperm cellularization and restore triploid seed viability. In agreement with this notion, PEG9 encodes an enzyme that acts on galactose, the carbohydrate building pectin.
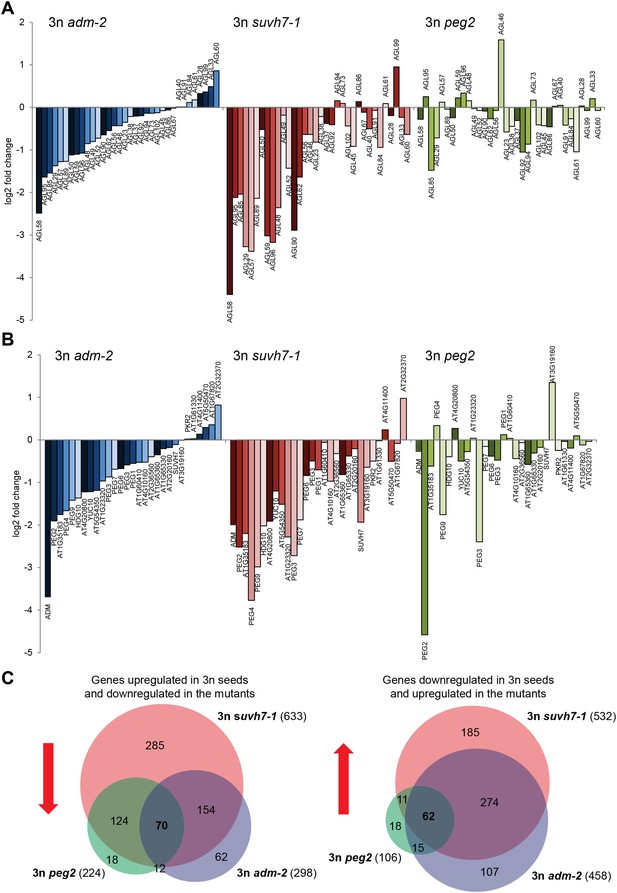
Transcriptome analysis of AGL genes and PEGs in triploid adm-2, suvh7-1 and peg2 seeds.
(A) Log2 fold change expression of AGLs in triploid adm-2, suvh7-1 and peg2 mutants compared to triploid wild-type seeds. Only AGLs were tested that were up-regulated in triploid wild-type seeds. (B) Log2 fold change expression of PEGs in triploid adm-2, suvh7-1 and peg2 mutants compared to triploid wild-type seeds. Only PEGs were tested that were up-regulated in triploid wild-type seeds. (C) Left panel: Venn diagram showing overlap of genes being up-regulated in seeds derived from wild type x osd1 crosses (signal log ratio [SLR] > 1, p < 0.05) and down-regulated in wild type x adm-2 osd1 (SLR < −1, p < 0.05), wild type x suvh7-1 osd1 (SLR < −1, p < 0.05), and wild type x peg2 osd1 (SLR < −1, p < 0.05) Hypergeometric testing was used to test for significance of overlap; p = 5.853e-09. Right panel: Venn diagram showing overlap of genes being down-regulated in seeds derived from wild type x osd1 crosses (SLR < −1, p < 0.05) and up-regulated in wild type x adm-2 osd1 (SLR >1, p < 0.05), wild type x suvh7-1 osd1 (SLR >1, p < 0.05), and wild type x peg2 osd1 (SLR >1, p < 0.05). Hypergeometric testing was used to test for significance of overlap; p = 4.622 e−16.
-
Figure 3—source data 1
List of genes deregulated in seeds derived from interploidy crosses.
- https://doi.org/10.7554/eLife.10074.014
Endosperm cellularization failure correlates with deregulated expression of genes encoding for pectin degrading enzymes
To test the hypothesis that endosperm cellularization failure in triploid seeds is a consequence of disturbed pectin degradation pathways, we analyzed expression of 173 genes acting in pectin degrading pathways (pectate lyases (GO:0030570), pectin methylesterases (GO:0030599) and polygalacturonases (GO:0004650)). Of those, 33 genes were upregulated in triploid seeds (Figure 4A). In contrast, expression of pectin biosynthesis genes (Atmodjo et al., 2013) was not negatively affected in triploid seeds (Figure 4B), suggesting that pectin degradation rather than pectin biosynthesis is disturbed in triploid seeds. Most of the 33 pectin degradation genes remained repressed until the heart stage of embryo development in wild-type seeds (Figure 4C) and became expressed at the cotyledon stage of embryo development, concomitantly with endosperm degradation (Figure 4C). In concordance with restored endosperm cellularization in triploid peg mutants, expression of 27 out of 33 pectin degradation genes that were upregulated in triploid seeds was normalized in at least one of the peg mutants (Figure 4D). Pectins are polymerized and methylesterified in the golgi and secreted into the cell wall as highly methylesterified forms. Subsequently, they can be modified by pectinases such as pectin methylesterases that catalyse the demethylesterification of homogalacturonans releasing acidic pectins, which can be visualized by ruthenium red binding (Downie et al., 1998; Micheli, 2001). Before endosperm cellularization, the ruthenium red signal was similar between diploid and triploid seeds (Figure 4E; Figure 4—figure supplement 1), suggesting that there were no major differences in pectin synthesis and degradation between diploid and triploid seeds. At 6 DAP the wild-type endosperm was largely cellularized and only a weak ruthenium red signal could be detected (Figure 4E; Figure 4—figure supplement 1), in agreement with pectin being deposited in the cell wall in a highly methylesterified form that is less intensively stained by ruthenium red (Micheli, 2001). Consistent with the expression of genes encoding for pectin degrading enzymes in 6 DAP triploid seeds (Figure 4D), the ruthenium red signal in the uncellularized endosperm at 6 DAP seeds was substantially weaker compared to the signal in 4DAP seeds (Figure 4E; Figure 4—figure supplement 1).
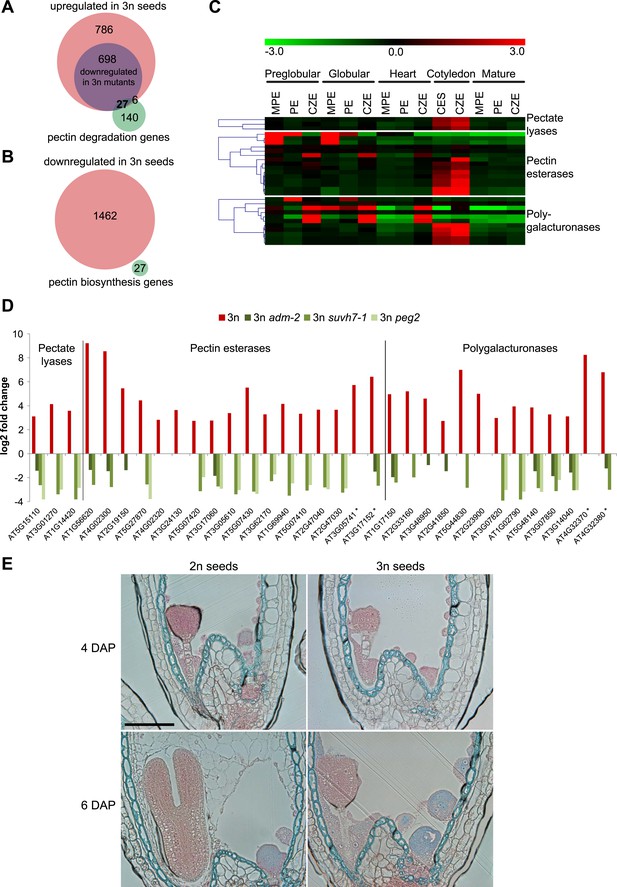
Analysis of pectin biosynthesis and degradation genes in diploid and triploid seeds.
(A) Venn diagram showing overlap of pectin degradation genes and genes being upregulated in seeds derived from wild type x osd1 crosses (signal log ratio [SLR] > 1, p < 0.05) and genes being downregulated in either wild type x adm-2 osd1 (SLR < −1, p < 0.05), wild type x suvh7-1 osd1 (SLR < −1, p < 0.05), or wild type x peg2 osd1 (SLR < −1, p < 0.05). Hypergeometric testing was used to test for significance of overlap, p = 4.048 e−10. (B) Venn diagram showing overlap of pectin biosynthesis genes and genes being down-regulated in seeds derived from wild type x osd1 crosses (SLR <1, p < 0.05). (C) Cluster analysis of pectin degradation genes that are upregulated in triploid wild-type seeds, based on their expression in endosperm during different stages of diploid seed development (Belmonte et al., 2013). Each row represents a gene, and each column represents a tissue type. Tissue types are: micropylar (MPE), peripheral (PE), chalazal (CZE) and cellularized endosperm (CES) derived from seeds containing embryos of the preglobular stage to the mature stage. Red or green indicate tissues in which a particular gene is highly expressed or repressed, respectively. (D) Log2 fold change expression of pectin degradation genes in triploid wild-type seeds (compared to diploid wild-type seeds) and triploid adm-2, suvh7-1 and peg2 mutants (compared to triploid wild-type seeds). Genes marked by an asterisk are not included in the seed transcriptome dataset (Belmonte et al., 2013) and are therefore not included in panel (C). (E) Ruthenium red staining of sections of seeds derived from crosses of Col plants pollinated with Col and osd1 at 4 DAP and 6 DAP. Red color marks the presence of demethylesterified pectin, blue color is derived from the counterstain with toluidine blue. A minimum of 100 seeds was analyzed for each cross. Scale bar, 0.1 mm.
Together, our data reveal that a subset of PEGs has a functional role in establishing interploidy hybridization barriers and that bypass of the barriers occurs by mechanisms converging on endosperm cellularization likely by suppression of pectin degradation. Thus, a subset of PEGs maintains a functional role in Arabidopsis, which is only revealed upon deregulation in triploid seeds.
Material and methods
Plant material and growth conditions
Request a detailed protocolA. thaliana mutants adm-2 (Kradolfer et al., 2013), pkr2-1 (Aichinger et al., 2009) and yuc10 (Cheng et al., 2007) have been described previously. peg1 (SAIL_659_B07), peg2 (SALK_143382), peg3 (SALK_000257), peg4 (GABI_022C09), peg5 (SALK_142234), peg6 (WiscDsLox428C06), peg7 (SALK_103601), peg8 (SALK_022399), peg9-1 (SALK_102165), peg9-2 (SALK_047888), fxg1 (SAIL_888B03), hdg10 (SALK_116071), suvh7-1 (GABI_037C06), suvh7-2 (SALK_112939), suvh7-3 (WiscDSLox297300_14H) and vim5 (SALK_033892) T-DNA insertion mutants were received from the Arabidopsis stock center (arabidopsis.info) and mutant plants were identified using primers listed in Supplementary file 1. The osd1 mutant (d'Erfurth et al., 2009) was kindly provided by Raphael Mercier. Being originally identified in the Nossen background, the mutant was introgressed into Col by repeated backcrossing over five generations. Plants were grown in a growth cabinet under long day photoperiods (16 hr light and 8 hr dark) at 22°C. After 10 days, seedlings were transferred to soil and plants were grown in a growth chamber at 60% humidity and daily cycles of 16 hr light at 22°C and 8 hr darkness at 18°C. For all crosses, designated female plants were emasculated and the pistils were hand-pollinated 2 days after emasculation.
RNA sequencing and expression analysis
Request a detailed protocolFor RNA sequencing, seeds from 20 siliques of Ler x Col, Ler x osd1, Ler x adm-2 osd1, Ler x suvh7-1 osd1, and Ler x peg2 osd1 were harvested at 6 DAP into RNA later (Sigma–Aldrich, St Louis, Missouri) in duplicates and homogenized (Silamat S5; IvoclarVivadent, Germany) using glass beads (1.25–1.55 mm; Carl Roth, Germany). RNA was extracted following a modified protocol for the RNAqueous kit (Ambion, Life Technologies, Carlsbad, California). RNA was purified by Qiagen RNeasy Plant Mini Kit (Qiagen, Germany) after residual DNA removed by 2 μL DNaseI (Thermo-Scientific, Waltham, Massachusetts). Libraries were prepared using the Truseq RNA Sample Preparation Kit (Illumina, San Diego, California) and sequenced at the SciLife Laboratory (Uppsala, Sweden) on an Illumina HiSeq2000 on two lanes in 100-bp paired-end mode. Sequencing reads have been deposited as fastq files in the Gene Expression Omnibus (Santos-González, 2015). For qPCR expression analysis three siliques of each cross were harvested, flash-frozen in liquid nitrogen and samples were disrupted using glass beads (1.25–1.55 mm; Carl Roth) and a Silamat S5 machine (IvoclarVivadent). RNA was extracted using the RNeasy Plant Mini Kit (Qiagen) according to the manufacturer's instructions and residual DNA was removed using the RNase-free DNase set (Qiagen). cDNA was synthesized using the first-strand cDNA synthesis kit (Thermo-Scientific) according to the manufacturer's instructions. Quantitative real-time PCR was performed using an iQ5 Real-Time PCR detection system (Bio-Rad, Hercules, California) in triplicates using Maxima SYBR green master mix (Thermo-Scientific). Results were analyzed as described by Simon (2003) using ACTIN11 as a reference gene and qPCR primers are listed in Supplementary file 1.
High-throughput RNA sequence analysis
Request a detailed protocolGene expression data were quality trimmed and mapped to the Arabidopsis TAIR10 reference genome using TopHat v2.0.10 (Trapnell et al., 2009). A maximum of 1 alignment to the reference was allowed for any given read, and the minimum anchor length was 10 bases. Differentially regulated genes across the two replicates were detected using the rank product method (Breitling et al., 2004) as implemented in the Bioconductor RankProd Package (Hong et al., 2011). The test was run with 100 permutations and gene selection was corrected for multiple comparison errors using a pfp (percentage of false prediction) < 0.05. Gene ontology (GO) categories were identified using AtCOECiS (Vandepoele et al., 2009).
Imprinting analysis
Request a detailed protocolTo determine the imprinting status of selected genes, RNA was extracted from crosses Col x Col, Ler x Ler, Ler x Col and Ler x osd1 at 4 DAP. Primers used for allele specific expression analysis are specified in Supplementary file 1 and PCR products were analyzed by DNA sequencing.
Microscopy
Request a detailed protocolSeeds were fixed and embedded with Technovit 7100 (Heraeus, Germany) as described (Hehenberger et al., 2012). Five-micrometer sections were prepared with an HM 355 S microtome (Microm, Germany) using glass knives. Sections were stained for 1 min with 0.1% toluidine blue and washed three times with distilled water. For pectin analysis, sections were stained for 45 min with 0.025% ruthenium red, counterstained for 1 min with 0.1% toluidine blue and washed three times with distilled water. At least ten seeds were analyzed per genotype and timepoint. Microscopy was performed using a DMI 4000B microscope with DIC optics (Leica, Germany). Images were captured using a DFC360 FX camera (Leica) and processed using Photoshop CS5 (Adobe, San Jose, California).
Seed size analysis
Request a detailed protocolSiliques were harvested when they turned brown and prior dehiscence. Mature seeds were separated from the siliques, spread on a document scanner with backlight unit (Scanmaker i800; Mikrotek, Taiwan) and analyzed as previously described (Herridge et al., 2011). Measurements were normalized by dividing by the average seed area and plotted on histograms. To determine statistical differences between sample and control crosses, Bonferroni corrected F-Tests were performed at a level of α = 0.01.
Germination and transmission analysis
Request a detailed protocolSeeds were surface sterilized in a container using chlorine gas (10 ml hydrochloric acid plus 50 ml sodium hypochlorite) and incubated for up to 3 hr. To determine germination frequency, seeds were plated on ½ MS media containing 1% sucrose, stratified at 4°C for 2 days in the dark and grown in a growth cabinet under long day photoperiods (16 hr light and 8 hr dark) at 22°C for 10 days. For transmission analysis, seedlings were harvested after 12 days and genotyped using primers specified in Supplementary file 1.
Generation of plasmids and transgenic lines
Request a detailed protocolFor the generation of a Col PEG2::PEG2 construct, At1g49290 including 1.5 kb of upstream secuence was amplified by PCR using primers specified in Supplementary file 1. The product was cloned into pENTR/D-TOPO (Invitrogen, Carlsbad, California), followed by clonase reaction with the pB7FWG2 vector (Karimi et al., 2002), from which the 35S promoter was removed. The Col PEG2::PEG2 construct was introduced into the peg2/− osd1/+ double mutant using Agrobacterium tumefaciens-mediated transformation (Clough and Bent, 1998) and transformants were selected on ½ MS media containing 30 mg/L phosphinotricin. Independent T2 lines were selected for single locus insertions and eight independent PEG2::PEG2 lines (in peg2/− osd1/− background) were used for pollinations onto Col wild-type plants.
Data availability
-
Paternally expressed imprinted genes establish postzygotic hybridization barriers in Arabidopsis thalianaPublicly available at the NCBI Gene Expression Omnibus(GSE62401).
References
-
Evolving views of pectin biosynthesisAnnual Review of Plant Biology 64:747–779.https://doi.org/10.1146/annurev-arplant-042811-105534
-
Comprehensive developmental profiles of gene activity in regions and subregions of the Arabidopsis seedProceedings of the National Academy of Sciences of USA 110:E435–E444.https://doi.org/10.1073/pnas.1222061110
-
A gel diffusion assay for quantification of pectin methylesterase activityAnalytical Biochemistry 264:149–157.https://doi.org/10.1006/abio.1998.2847
-
Genomic imprinting: insights from plantsAnnual Review of Genetics 47:187–208.https://doi.org/10.1146/annurev-genet-110711-155527
-
Imprinting in the endosperm: a possible role in preventing wide hybridizationPhilosophical Transactions of the Royal Society of London. Series B, Biological Sciences 358:1105–1111.https://doi.org/10.1098/rstb.2003.1292
-
Parent-specific gene expression and the triploid endospermThe American Naturalist 134:147–155.https://doi.org/10.1086/284971
-
Structure and function of enzymes of the leloir pathway for galactose metabolismThe Journal of Biological Chemistry 278:43885–43888.https://doi.org/10.1074/jbc.R300025200
-
Rankprod: rank product method for identifying differentially expressed genes with application in meta-analysisRankprod: rank product method for identifying differentially expressed genes with application in meta-analysis, R package version 2.32.0.
-
Inaugural article: regulation of imprinted gene expression in Arabidopsis endospermProceedings of the National Academy of Sciences of USA 108:1755–1762.https://doi.org/10.1073/pnas.1019273108
-
Epigenetic resetting of a gene imprinted in plant embryosCurrent Biology 19:1677–1681.https://doi.org/10.1016/j.cub.2009.08.053
-
Gateway vectors for agrobacterium-mediated plant transformationTrends in Plant Science 7:193–195.https://doi.org/10.1016/S1360-1385(02)02251-3
-
Reproductive barrier and genomic imprinting in the endosperm of flowering plantsGenes & Genetic Systems 82:177–186.https://doi.org/10.1266/ggs.82.177
-
Endosperm: food for humankind and fodder for scientific discoveriesThe New Phytologist 195:290–305.https://doi.org/10.1111/j.1469-8137.2012.04182.x
-
Pectin methylesterases: cell wall enzymes with important roles in plant physiologyTrends in Plant Science 6:414–419.https://doi.org/10.1016/S1360-1385(01)02045-3
-
Paternally expressed imprinted genes establish postzygotic hybridization barriers in Arabidopsis thalianaPaternally expressed imprinted genes establish postzygotic hybridization barriers in Arabidopsis thaliana, GSE62401, NCBI Gene Expression Omnibus, http://www.ncbi.nlm.nih.gov/geo/query/acc.cgi?acc=GSE62401.
-
Parent-of-origin effects on seed development in Arabidopsis ThalianaDevelopment 125:3329–3341.
-
Q-gene: processing quantitative real-time Rt-Pcr dataBioinformatics 19:1439–1440.https://doi.org/10.1093/bioinformatics/btg157
-
Tophat: discovering splice junctions with Rna-SeqBioinformatics 25:1105–1111.https://doi.org/10.1093/bioinformatics/btp120
-
Genomic imprinting, methylation and parent-of-origin effects in reciprocal hybrid endosperm of castor beanNucleic Acids Research 42:6987–6998.https://doi.org/10.1093/nar/gku375
Article and author information
Author details
Funding
European Research Council (ERC) (ERC-2011-StG - 280496)
- Claudia Köhler
Vetenskapsrådet (Swedish Research Council) (2014-3820)
- Claudia Köhler
Knut och Alice Wallenbergs Stiftelse (Knut and Alice Wallenberg Foundation)
- Claudia Köhler
Stiftelsen Olle Engkvist Byggmästare
- Claudia Köhler
The funders had no role in study design, data collection and interpretation, or the decision to submit the work for publication.
Acknowledgements
We thank Lars Hennig for critical comments on the manuscript. Sequencing was performed by the SNP&SEQ Technology Platform, Science for Life Laboratory at Uppsala University, a national infrastructure supported by the Swedish Research Council (VRRFI) and the Knut and Alice Wallenberg Foundation. This research was supported by a European Research Council Starting Independent Researcher grant (to CK), a grant from the Swedish Science Foundation (to CK) and a grant from the Knut and Alice Wallenberg Foundation (to CK).
Copyright
© 2015, Wolff et al.
This article is distributed under the terms of the Creative Commons Attribution License, which permits unrestricted use and redistribution provided that the original author and source are credited.
Metrics
-
- 4,724
- views
-
- 937
- downloads
-
- 98
- citations
Views, downloads and citations are aggregated across all versions of this paper published by eLife.
Download links
Downloads (link to download the article as PDF)
Open citations (links to open the citations from this article in various online reference manager services)
Cite this article (links to download the citations from this article in formats compatible with various reference manager tools)
Further reading
-
- Developmental Biology
The morphogen FGF8 establishes graded positional cues imparting regional cellular responses via modulation of early target genes. The roles of FGF signaling and its effector genes remain poorly characterized in human experimental models mimicking early fetal telencephalic development. We used hiPSC-derived cerebral organoids as an in vitro platform to investigate the effect of FGF8 signaling on neural identity and differentiation. We found that FGF8 treatment increases cellular heterogeneity, leading to distinct telencephalic and mesencephalic-like domains that co-develop in multi-regional organoids. Within telencephalic regions, FGF8 affects the anteroposterior and dorsoventral identity of neural progenitors and the balance between GABAergic and glutamatergic neurons, thus impacting spontaneous neuronal network activity. Moreover, FGF8 efficiently modulates key regulators responsible for several human neurodevelopmental disorders. Overall, our results show that FGF8 signaling is directly involved in both regional patterning and cellular diversity in human cerebral organoids and in modulating genes associated with normal and pathological neural development.
-
- Developmental Biology
Wnt signaling plays crucial roles in embryonic patterning including the regulation of convergent extension (CE) during gastrulation, the establishment of the dorsal axis, and later, craniofacial morphogenesis. Further, Wnt signaling is a crucial regulator of craniofacial morphogenesis. The adapter proteins Dact1 and Dact2 modulate the Wnt signaling pathway through binding to Disheveled. However, the distinct relative functions of Dact1 and Dact2 during embryogenesis remain unclear. We found that dact1 and dact2 genes have dynamic spatiotemporal expression domains that are reciprocal to one another suggesting distinct functions during zebrafish embryogenesis. Both dact1 and dact2 contribute to axis extension, with compound mutants exhibiting a similar CE defect and craniofacial phenotype to the wnt11f2 mutant. Utilizing single-cell RNAseq and an established noncanonical Wnt pathway mutant with a shortened axis (gpc4), we identified dact1/2-specific roles during early development. Comparative whole transcriptome analysis between wildtype and gpc4 and wildtype and dact1/2 compound mutants revealed a novel role for dact1/2 in regulating the mRNA expression of the classical calpain capn8. Overexpression of capn8 phenocopies dact1/2 craniofacial dysmorphology. These results identify a previously unappreciated role of capn8 and calcium-dependent proteolysis during embryogenesis. Taken together, our findings highlight the distinct and overlapping roles of dact1 and dact2 in embryonic craniofacial development, providing new insights into the multifaceted regulation of Wnt signaling.