Pulmonary Hypertension: When cell teamwork turns toxic
Cells rely on cues from their environment to develop and work properly. Yet this interdependence can turn perilous when one cell goes rogue, triggering an unchecked cascade of dysfunction. For decades, understanding these toxic cellular relationships has been hindered by a fundamental challenge: the inability to simultaneously study molecular details and tissue-wide interactions. Earlier studies often prioritized one scale at the expense of the other and, because this, missed critical connections.
A striking biological example is the cellular interplay in pulmonary hypertension. This life-threatening disease, defined by an increased pressure in the blood vessels of the lungs, often ultimately leads to heart failure and death (Humbert et al., 2019). At its roots, pulmonary hypertension arises from the toxic interaction between multiple cell types compromising each other’s normal function (Frid et al., 2020). Under healthy conditions, smooth muscle cells in the arteries of the lungs (known as PASMCs) contract and dilate, regulating flow and pressure in the lung vasculature. Their counterparts, the fibroblast cells that form the vessel’s outer layer (or PAAFs), protect PASMCs and mediate communication between the two cell types (Stenmark et al., 2018).
In pulmonary hypertension, however, this partnership turns lethal. PAAFs start remodeling their external environment and secrete signals that cause PASMCs to lose their ability to contract, depriving them of their main function (Park et al., 2022). Instead, the PASMCs start stiffening, causing a cascade of irreversible vascular changes that represent a cornerstone in the development of pulmonary hypertension (Crnkovic et al., 2022).
With PAAFs and PASMCs at the forefront of this disease, many questions remain unanswered. What triggers PAAF activation? How do PASMCs lose their contractility? Is there an underlying shared mechanism linking PAAF and PASMC dysfunction? Crnkovic et al., 2022 Answering these questions could lead to new and more effective therapies for a number of deadly diseases.
Now, in eLife, Grazyna Kwapiszewska, Vinicio de Jesus Perez and colleagues at various research institutes in Austria, Germany and the United States – including Slaven Crnkovic and Helene Thekkekara Puthenparampi as joint first authors – report how PAAFs force PASMCs into a diseased state (Crnkovic et al., 2025).
Crnkovic et al. isolated PAAFs and PASMCs from both healthy donors and pulmonary hypertension patients, comparing the gene activity and protein levels between these two groups. They found that diseased PAAFs produced more collagen, which stiffens tissues, while simultaneously underproducing laminin, a protein that provides structure for collagen deposition (Figure 1). This collagen-laminin imbalance created a rigid environment, similar to a building with too much concrete and not enough scaffolding. The mechanical stress from the stiffened matrix forced PASMCs to abandon their contractile function, a hallmark of their healthy state (Crnkovic et al., 2022).
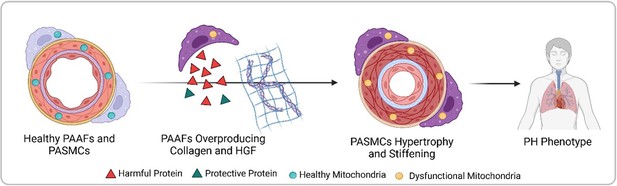
Progression from healthy pulmonary artery adventitial fibroblasts (PAAFs) and pulmonary artery smooth muscle cells (PASMCs) to pulmonary hypertension (PH) phenotype.
In healthy arteries (left), PASMCs are dynamic cells that regulate flow and pressure in the lung vasculature by contracting and dilating, while the fibroblasts on the vessel’s outer layer (PAAFs) protect PASMCs (green triangles). In pulmonary hypertension, PAAFs overproduce collagen and harmful proteins (red triangles) that cause PASMCs to become rigid. Imbalanced protein dynamics and mitochondrial dysfunction in both cell types further contribute to the diseased state (right). Created with BioRender.com.
In a parallel mechanism, diseased PAAFs exacerbated dysfunction by overproducing harmful signals while downregulating protective proteins, thus reprogramming PASMCs into a dysregulated state. As dynamic cells require constant energy, PASMCs rely heavily on mitochondria, especially in times of added cellular stress (Qin et al., 2023). Given the central role of mitochondria in generating cellular energy, regulating harmful molecules, and maintaining an adequate intracellular balance – all of which are affected in pulmonary hypertension – Crnkovic et al. investigated the mitochondria’s role in reprogramming PAAFs and PASMCs (Chan et al., 2009).
Next, mitochondrial function was evaluated by measuring membrane potential, oxygen consumption and levels of harmful reactive species, which linked the observed mitochondrial compromise to subsequent DNA damage. This analysis revealed that mitochondrial dysregulation occurred when both cell types transitioned to a diseased state. Mitochondria are crucial in protecting the cell’s DNA by neutralizing harmful molecules, especially in diseased states. However, because the mitochondria were damaged, the DNA suffered additional stress, worsening cellular injury.
In pulmonary hypertension, microenvironment stiffness and mitochondrial dysfunction are two sides of the same coin. The rigid environment forces PASMCs into a state of mechanical stress, overloading their mitochondria. In turn, dysfunctional mitochondria, while unable to protect the DNA, also leak harmful molecules, worsening cellular remodeling. This vicious cycle, where mechanics and metabolism unite, traps the cells in a diseased state leading to irreversible vascular damage. By uncovering these pathways, Crnkovic et al. revealed that targeting PAAF signaling has the potential to reverse PASMC dysfunction in pulmonary hypertension. Halting toxic crosstalk and mitochondrial damage could thus reverse vascular stiffening, a potential therapeutic breakthrough for this disease.
While this study advances our understanding of pulmonary hypertension, certain limitations must be acknowledged. First, by focusing only on samples from patients with an established disease, early markers of disease triggers remain unexplored (Stacher et al., 2012). Second, the study only focused on PASMCs and PAAFs, potentially excluding other significant but understudied cell types in the progression of the condition (El Kasmi et al., 2014). Third, the origin of the reported mitochondrial dysfunction, whether a cause or a consequence of pulmonary hypertension, remains unclear.
By evaluating the interplay between different cell types while pinpointing precise genetic targets, Crnkovic et al. highlight the transformative potential and inherent challenges of studying genes and protein profiles in pulmonary hypertension. Leveraging this approach in future work, researchers may be able to shed light on pulmonary hypertension’s full story and provide a therapeutic road map to restore healthy cell states for a disease with no current cure.
References
-
Immunoglobulin-driven complement activation regulates proinflammatory remodeling in pulmonary hypertensionAmerican Journal of Respiratory and Critical Care Medicine 201:224–239.https://doi.org/10.1164/rccm.201903-0591OC
-
Pathology and pathobiology of pulmonary hypertension: state of the art and research perspectivesThe European Respiratory Journal 53:1801887.https://doi.org/10.1183/13993003.01887-2018
-
Sox17 deficiency promotes pulmonary arterial hypertension via HGF/c-Met signalingCirculation Research 131:792–806.https://doi.org/10.1161/CIRCRESAHA.122.320845
-
Arterial remodeling: the role of mitochondrial metabolism in vascular smooth muscle cellsAmerican Journal of Physiology. Cell Physiology 324:C183–C192.https://doi.org/10.1152/ajpcell.00074.2022
-
Modern age pathology of pulmonary arterial hypertensionAmerican Journal of Respiratory and Critical Care Medicine 186:261–272.https://doi.org/10.1164/rccm.201201-0164OC
Article and author information
Author details
Publication history
Copyright
© 2025, Khoury and Chan
This article is distributed under the terms of the Creative Commons Attribution License, which permits unrestricted use and redistribution provided that the original author and source are credited.
Download links
Downloads (link to download the article as PDF)
Open citations (links to open the citations from this article in various online reference manager services)
Cite this article (links to download the citations from this article in formats compatible with various reference manager tools)
Further reading
-
- Cell Biology
The primary cilium is a microtubule-based organelle that cycles through assembly and disassembly. In many cell types, formation of the cilium is initiated by recruitment of preciliary vesicles to the distal appendage of the mother centriole. However, the distal appendage mechanism that directly captures preciliary vesicles is yet to be identified. In an accompanying paper, we show that the distal appendage protein, CEP89, is important for the preciliary vesicle recruitment, but not for other steps of cilium formation (Kanie et al., 2025). The lack of a membrane-binding motif in CEP89 suggests that it may indirectly recruit preciliary vesicles via another binding partner. Here, we identify Neuronal Calcium Sensor-1 (NCS1) as a stoichiometric interactor of CEP89. NCS1 localizes to the position between CEP89 and the centriole-associated vesicle marker, RAB34, at the distal appendage. This localization was completely abolished in CEP89 knockouts, suggesting that CEP89 recruits NCS1 to the distal appendage. Similar to CEP89 knockouts, preciliary vesicle recruitment as well as subsequent cilium formation was perturbed in NCS1 knockout cells. The ability of NCS1 to recruit the preciliary vesicle is dependent on its myristoylation motif and NCS1 knockout cells expressing a myristoylation defective mutant failed to rescue the vesicle recruitment defect despite localizing properly to the centriole. In sum, our analysis reveals the first known mechanism for how the distal appendage recruits the preciliary vesicles.
-
- Cell Biology
Distal appendages are ninefold symmetric blade-like structures attached to the distal end of the mother centriole. These structures are critical for the formation of the primary cilium, by regulating at least four critical steps: preciliary vesicle recruitment, recruitment and initiation of intraflagellar transport (IFT), and removal of CP110. While specific proteins that localize to the distal appendages have been identified, how exactly each protein functions to achieve the multiple roles of the distal appendages is poorly understood. Here, we comprehensively analyze known and newly discovered distal appendage proteins (CEP83, SCLT1, CEP164, TTBK2, FBF1, CEP89, KIZ, ANKRD26, PIDD1, LRRC45, NCS1, CEP15) for their precise localization, order of recruitment, and their roles in each step of cilia formation. Using CRISPR-Cas9 knockouts, we show that the order of the recruitment of the distal appendage proteins is highly interconnected and a more complex hierarchy. Our analysis highlights two protein modules, CEP83-SCLT1 and CEP164-TTBK2, as critical for structural assembly of distal appendages. Functional assays revealed that CEP89 selectively functions in the RAB34+ vesicle recruitment, while deletion of the integral components, CEP83-SCLT1-CEP164-TTBK2, severely compromised all four steps of cilium formation. Collectively, our analyses provide a more comprehensive view of the organization and the function of the distal appendage, paving the way for molecular understanding of ciliary assembly.