Serotonin modulates insect hemocyte phagocytosis via two different serotonin receptors
Abstract
Serotonin (5-HT) modulates both neural and immune responses in vertebrates, but its role in insect immunity remains uncertain. We report that hemocytes in the caterpillar, Pieris rapae are able to synthesize 5-HT following activation by lipopolysaccharide. The inhibition of a serotonin-generating enzyme with either pharmacological blockade or RNAi knock-down impaired hemocyte phagocytosis. Biochemical and functional experiments showed that naive hemocytes primarily express 5-HT1B and 5-HT2B receptors. The blockade of 5-HT1B significantly reduced phagocytic ability; however, the blockade of 5-HT2B increased hemocyte phagocytosis. The 5-HT1B-null Drosophila melanogaster mutants showed higher mortality than controls when infected with bacteria, due to their decreased phagocytotic ability. Flies expressing 5-HT1B or 5-HT2B RNAi in hemocytes also showed similar sensitivity to infection. Combined, these data demonstrate that 5-HT mediates hemocyte phagocytosis through 5-HT1B and 5-HT2B receptors and serotonergic signaling performs critical modulatory functions in immune systems of animals separated by 500 million years of evolution.
https://doi.org/10.7554/eLife.12241.001eLife digest
Serotonin is a small molecule found in organisms across the animal kingdom. This molecule plays various roles in the human body and affects many systems including the gut and central nervous system. Over recent decades, serotonin has been found to play a role in the immune system too, and appears to help regulate how immune cells respond to invasion by infectious bacteria or viruses. Various types of immune cells that can engulf foreign particles or microorganisms via a process called phagocytosis have receptors for serotonin on their cell surface and are activated when serotonin is present.
Signaling pathways associated with part of the immune system in mammals are often highly similar to pathways found in insects. Serotonin is also known to influence many processes in insects, such as appetite, sleep and reproduction, but its role in the insect’s immune system was not well understood. In particular, insects have phagocytic cells known as hemocytes and it was unknown if serotonin helps to activate these cells.
Qi, Huang et al. have now discovered that serotonin does indeed control the activity of insect hemocytes from the caterpillars of the small white butterfly (Pieris rapae)and the fruit fly (Drosophila melanogaster). The experiments showed that two distinct receptors on a hemocyte’s cell surface can detect serotonin. One of these receptors increases phagocytic activity in both insects, while the other has the opposite effect in the caterpillar and reduces this activity.Qi, Huang et al. also discovered that phagocytosis depends on which of these receptors is most common on the hemocyte cell surface, and demonstrated that insects exposed to bacteria start to produce more of the serotonin receptors that increase phagocytosis. Further experiments showed that fruit flies in which the gene for a serotonin receptor has been deleted are more vulnerable to bacterial infections due to their poor phagocytic ability.
Insects and mammals are separated by about 500 million years of evolution, and so these findings suggest that serotonin is an ancient signaling molecule that can control the immune system across the animal kingdom. The work also supports the idea that studies of the simpler immune systems of insects, including the model organisms such as D. melanogaster, can offer insight into the immune systems of humans and other animals.
https://doi.org/10.7554/eLife.12241.002Introduction
Serotonin (5-hydroxytryptamine, 5-HT) is one of the oldest neurotransmitters/hormones in evolution (Turlejski, 1996). It regulates or modulates a wide variety of processes in most invertebrates and vertebrates, such as metabolism (Sze et al., 2000) and locomotion (Ranganathan et al., 2000) in nematodes; reproduction (Anstey et al., 2009), learning and memory (Sitaraman et al., 2008) in insects; physiologic states and behaviors, including pain, appetite, mood, and sleep (Mössner and Lesch, 1998) in humans. 5-HT also plays an important role outside of the central nervous system (CNS) in immune signaling. Immune cells can synthesize and sequester 5-HT. For instance, human mast cells express the key peripheral 5-HT synthesizing enzyme, tryptophan hydroxylase 1 (TPH-1) (Kushnir-Sukhov et al., 2007, 2008). Mouse dendritic cells (DCs) express the serotonin transporter (SERT), taking up 5-HT from the microenvironment (O'Connell et al., 2006). 5-HT regulates immune responses and inflammatory cascades via distinct receptors and different immune cells have been shown to express a different composition of 5-HT receptor subtypes (Baganz and Blakely, 2013). 5-HT2A may contribute to chemotaxis of eosinophils (Boehme et al., 2008) and 5-HT2C receptors on alveolar macrophages can be activated by 5-HT (Mikulski et al., 2010). However, most studies assess the in vitro response of immune cells to pharmacological agents. Therefore, the function of 5-HT signaling in vivo in are still unclear.
Vertebrate blood cells (e.g. macrophages) have evolved a variety of strategies to internalize particles and solutes, including pinocytosis, receptor-mediated endocytosis, and phagocytosis, a highly conserved aspect of innate immunity. Phagocytosis, the uptake of large particles (>0.5 μm) into cells, allows for rapid engulfment of dying cells and pathogens by specialized phagocytes, such as macrophages and neutrophils in mammals (Aderem and Underhill, 1999). In insects, hemocyte phagocytosis is an important cellular defense response to pathogens and parasites (Lavine and Strand, 2002). In lepidopteran insects, the granulocytes and plasmatocytes are the major phagocytes (Kanost et al., 2004). Cross-talk between the immune and nervous system may play a role in regulating phagocytosis in insects during infection. However, the roles of serotonin in insect phagocytosis are less well characterized compared with vertebrate counterparts, although there is evidence that 5-HT can enhance phagocytosis (Baines et al., 1992; Kim et al.,2009).
Many of the intracellular signaling pathways that drive the insect immune system are very similar to those found in the mammalian innate immune system (Lemaitre and Hoffmann, 2007), and some of them were first uncovered in insects (Hoffmann, 2003). Indirect evidence suggests that similarities between insects and mammals extend to the molecular mechanisms involved in the neuroendocrine control of immune function (Adamo, 2008). Because of the relative simplicity of the insect immune system, examining the basic interactions between serotonin receptor-medicated second messenger systems and immune-related intracellular signaling pathways may be easier in insects. As an initial step toward this goal, we have made a comprehensive study in the caterpillar, Pieris rapae hemocytes to elucidate the function of 5-HT signaling in insect cellular immune responses. We found that hemocyte-derived 5-HT regulates phagocytosis in an autocrine manner through 5-HT1B and 5-HT2B receptors, each of which produces distinct effects. Mortality experiments using Drosophila mutants and hemocyte-specific RNAi-silencing further found that both 5-HT1B and 5-HT2B are necessary for effective resistance to bacterial infections.
Results
Hemocytes synthesize 5-HT in an activation-dependent manner
After activation with 100 ng/ml of lipopolysaccharide (LPS) for 2 hr, we detected 5-HT within hemocytes directly by immunolabeling and fluorescence microscopy. Figure 1A shows that granules of 5-HT labeled with 5-HT antisera (upper) are readily visible in the cytosol. As a negative control, 5-HT antisera preabsorbed with 5-HT were invisible (lower). Serotonin synthesis requires two enzymes, tryptophan hydroxylase and aromatic L-amino acid decarboxylase (AADC) (Figure 1B). Tryptophan hydroxylase is the rate-limiting enzyme in serotonin biosynthesis encoded by two genes, TPH and TRH in insects, TPH1 and TPH2 in mammals. Both gene products have tryptophan hydroxylase activity in vivo. The TPH/TPH1 gene is expressed in non-neural tissues, and the TRH/TPH2 gene is expressed in neural tissues (Côté et al., 2003; Neckameyer et al., 2007; Watanabe et al., 2011). The high-affinity SERT is a plasma membrane protein that can take up extracellular 5-HT (Rudnick, 2006; Torres et al., 2003). Thus, we performed RT-PCR to characterize TPH, TRH, and SERT expression in hemocytes. Figure 1C shows that hemocytes produced mRNA for TPH, but that transcripts of TRH and SERT were not detected. These results suggest that hemocytes do not selectively sequester 5-HT but can synthesize 5-HT using TPH. Then, we performed ELISA to quantify the amount of 5-HT released into the hemocytes’ culture media. 5-HT levels increased significantly above that found in controls in cell supernatants 1 hr after exposure of hemocytes to LPS. 5-HT levels reached maximal at 2 hr and decreased at 4 hr (Figure 1D). The real-time quantitative RT-PCR results also show that mRNA expression level of TPH was up-regulated by approximately sixfold relative to controls at 15 min and at 4 hr after LPS stimulation, suggesting a potential negative feedback regulation (Figure 1E). Therefore, it is concluded that hemocytes are able to synthesize and release 5-HT in vitro, and this activity is enhanced following their activation. We hypothesise that 5-HT may play an important role in hemocyte function.
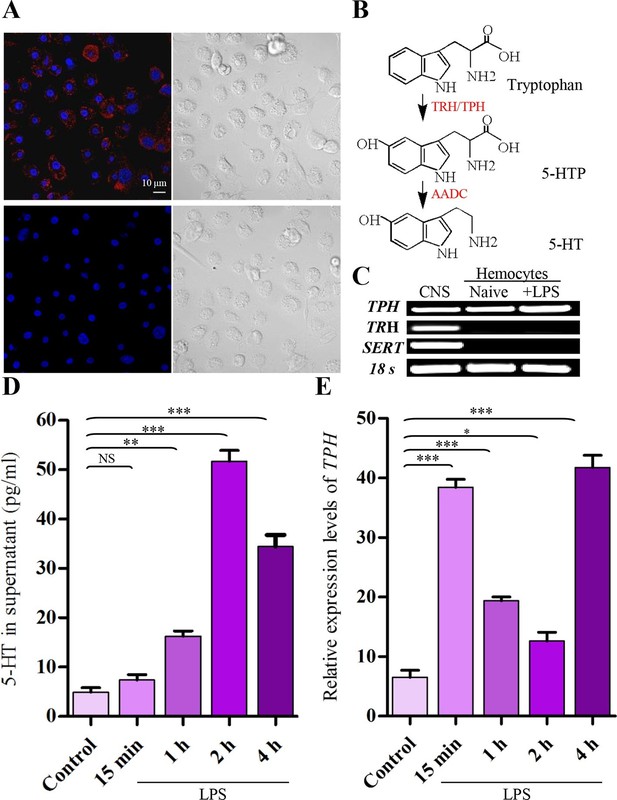
Activated hemocytes are capable of 5-HT synthesis.
(A) 5-HT was visualized by confocal microscopy in hemocytes activated with 100 ng/ml LPS by labeling with 5-HT antisera (Alexa Fluor 546; red) (upper left). 5-HT antisera was preabsorbed with 5-HT as the negative control (lower left). Nuclei were counterstained with DAPI (blue). Scale bar represents 10 μM. Data are representatives of two independent experiments. (B) Serotonin biosynthetic pathway. Tryptophan- phenylalanine hydroxylase (TRH/TPH), aromatic L-amino acid decarboxylase (AADC), 5-hydroxy tryptophan (5-HTP). (C) Expression of gene transcripts for TPH, TRH, and SERT were determined by RT-PCR from naive hemocytes and from hemocytes activated with 100 ng/ml LPS for 2 hr, central nervous system (CNS) as positive control. Data are representatives of three independent experiments. (D) 5-HT concentrations in hemocytes supernatants were determined by ELISA. Hemocytes were activated with 100 ng/ml LPS. Naive hemocytes treated with PBS are as control (n = 4). (E) Relative expression of TPH was quantified by real-time PCR. Hemocytes were activated with 100 ng/ml LPS. Naive hemocytes treated with PBS is as control (n = 3). One-way ANOVA followed by Tukey’s multiple comparison test for D and E. Error bars indicate ± s.e.m., ***p<0.001, **p<0.01, *p<0.05 and NS means no significant difference.
5-HT is involved in hemocyte phagocytosis
To examine the physiological role of 5-HT in hemocytes, we tested whether inhibition of 5-HT synthesis would affect hemocyte phagocytosis of Gram-negative E. coli bacteria labeled with pHrodo, a dye that fluoresces in the acidic environment of a mature phagosome upon fusion with lysosomes. α-methyltryptophan (AMTP) is a competitive inhibitor of 5-HT synthesis (Gal and Christiansen, 1975). As shown in Figure 2—figure supplement 1, 5-HT synthesis was significantly reduced in hemocytes after treatment with 10 μM AMTP, compared with PBS-treated controls. The results show that 10 μM AMTP also significantly impaired hemocyte phagocytic ability. Furthermore, treatment with exogenous 5-HT at 100 nM fully restored hemocyte phagocytosis (Figure 2A–D). Next, we tested siTPH-treated hemocytes (knock-down effect was approximately 80%, Figure 2E) for their phagocytosis ability. After incubation with siTPH for 48 hr, hemocyte phagocytosis rate was significantly decreased compared with the negative control. 100 nM 5-HT treatment could fully rescue the siTPH induced phenotype (Figure 2F–I). Collectively, these data indicate that hemocyte-derived 5-HT is critical for proper hemocyte phagocytosis upon immune challenge.
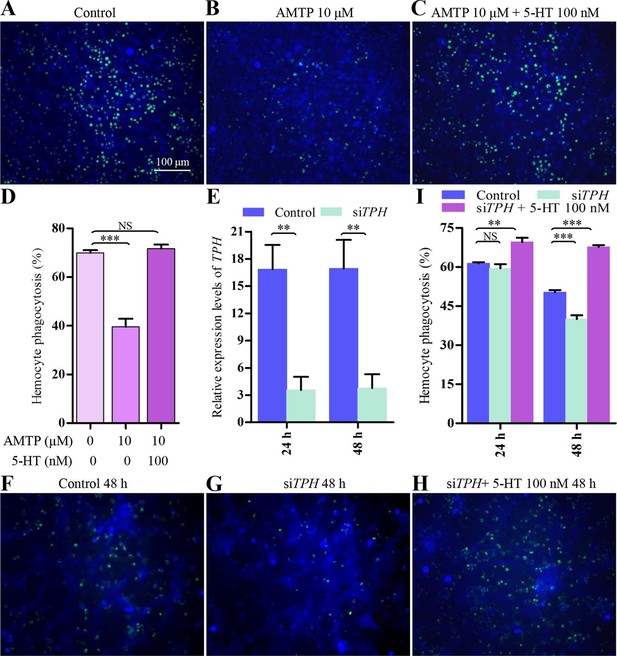
Inhibition of endogenous 5-HT synthesis impairs hemocyte phagocytosis.
(A-C) Hemocyte phagocytosis was visualized by florescence microscope. Hemocytes were stained with Cell Tracker Blue CMAC (blue), green represent the phagocytosed pHrodo E. coli. (D) Quantification of phagocytosis of E. coli by hemocytes (n = 3). (E) Confirmation of knock-down effect of TPH by real-time qPCR. The P. rapae18s rRNA gene was used as an internal reference gene (n = 4). (F-H) Effect of siTPH on hemocyte phagocytosis was visualized by florescence microscope. (I) Quantification of siTPH effect on hemocyte phagocytosis (n = 3). One-way ANOVA followed by Tukey’s multiple comparison test for D and I; two-tailed t-test for E. Error bars indicate ± s.e.m., ***p<0.001, **p<0.01 and NS means no significant difference.
5-HT regulates hemocyte phagocytosis via Pr5-HT1B and Pr5-HT2B
Since 5-HT is involved in the regulation of hemocyte function, it should exert its effects through corresponding 5-HT receptors. So far, five subtypes of 5-HT receptors including 5-HT1A, 5-HT1B, 5-HT2A, 5-HT2B and 5-HT7 are identified in insects (Blenau and Thamm, 2011; Gasque et al., 2013). 5-HT1A and 5-HT1B couple with Gi protein and decrease intracellular cAMP levels. 5-HT2A and 5-HT2B couple with Gq protein, which can induce increased intracellular Ca2+. 5-HT7 couples with Gs protein and increases cAMP levels (Blenau and Thamm, 2011; Gasque et al., 2013). We performed a comprehensive analysis of 5-HT receptor gene expression in hemocytes by RT-PCR after stimulation with 100 ng/ml LPS for 2 hr. The positive control samples were extracted from the CNS of P. rapae. As shown in Figure 3A, naive hemocytes express Pr5-HT1B, Pr5-HT2B and Pr5-HT7. We used specific antibodies to confirm the expression of Pr5-HT1B (Figure 3B) and Pr5-HT2B (Figure 3C) on the plasma membranes of hemocytes. We also performed Ca2+ imaging to further confirm functional expression of 5-HT2B in hemocytes. Both plasmatocytes and granulocytes, the two most abundant types of hemocytes, produced intracellular Ca2+ increase in response to 5-HT. 5-HT stimulated Ca2+ responses at concentrations ranging from 0.01 nM to 10 nM (maximal response), and its EC50 value was estimated at 0.15 nM (Figure 3—figure supplement 1).
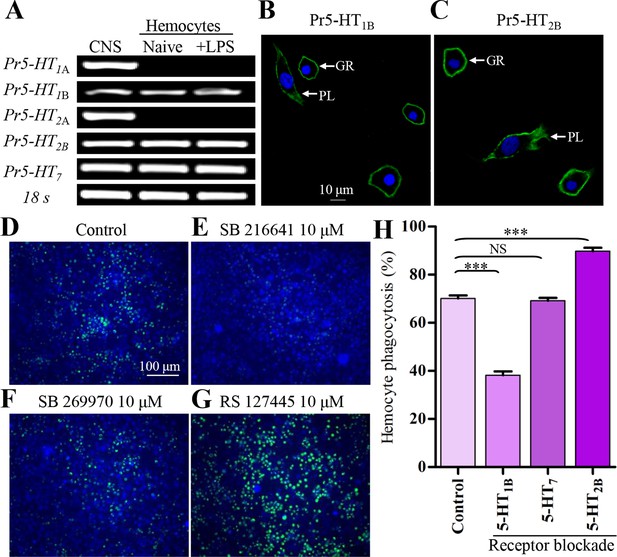
5-HT receptor subtypes expressed in naïve and LPS-activated hemocytes.
(A) Hemocytes were negatively purified and activated with 100 ng/ml LPS for 2 hr. The gene expression for 5-HTR subtype was examined by RT-PCR. Data are representatives of three independent experiments. (B) Gene expression for Pr5-HT1B was examined by immunofluorescence. The scale bar represents 10 μM. Data are representatives of two independent experiments. (C) Gene expression for Pr5-HT2B was examined by immunofluorescence. PL, plasmatocytes; GR, granulocytes. Data are representatives of two independent experiments. (D-G) The effect of different antagonist on hemocyte phagocytosis was visualized by florescence microscope. SB216641 is an antagonist of 5-HT1B. SB269970 is an antagonist of 5-HT7 and RS127445 is a human 5-HT2B antagonist. (H) Quantification of different antagonist on hemocyte phagocytosis. Data are from three independent experiments that each consists of cells from ten fifth-instar larvae. One-way ANOVA followed by Tukey’s multiple comparison test for H. Error bars indicate ± s.e.m., ***p<0.001, **p<0.01 and NS means no significant difference.
Since there are multiple 5-HT receptor types on the hemocyte membranes, we next investigated which one was involved in the 5-HT-mediated phagocytosis by pharmacological manipulation. Three selective 5-HT receptor antagonists (SB 216641, SB 269970, and RS 127445) were applied at a final concentration of 10 μM to block their corresponding receptors. The inhibition effects of SB 216641 and SB 269970 on Pr5-HT1B and Pr5-HT7 were also confirmed by cAMP assays in heterogeneous expression system (Figure 3—figure supplement 2). We found that only blockade of Pr5-HT1B significantly reduced the phagocytic ability of hemocytes. Blockade of Pr5-HT7 neither intensified nor reduced hemocyte phagocytosis. Surprisingly, blockade of 5-HT2B significantly increased hemocyte phagocytosis (Figure 3D–H).
We further found that the 5-HT1B blocker SB 216641 affected hemocyte phagocytosis in a dose-dependent manner (Figure 4A). To confirm the above results, we performed siRNA-mediated interference to knock-down each receptor in hemocytes. The results showed that siPr5-HT1Bdecreased Pr5-HT1B expression significantly at both mRNA and protein levels (Figures 4B–C) after 24 hr and 48 hr, respectively. As expected, the siPr5-HT1B treated hemocytes phagocytose E. coli poorly compared with control (Figure 4D–F). Significantly knock-down of Pr5-HT2B was also observed at both transcript and protein levels (Figure 4—figure supplement 1A–B) at 48 hr, which promoted hemocyte phagocytosis (Figure 4—figure supplement 1C–E). However, knock-down of Pr5-HT7have no significant effect on hemocyte phagocytosis (Figure 4—figure supplement 1F–G). The results demonstrate that 5-HT mediates hemocyte phagocytosis through 5-HT1B and 5-HT2B receptors but that these two receptors act in opposite ways.
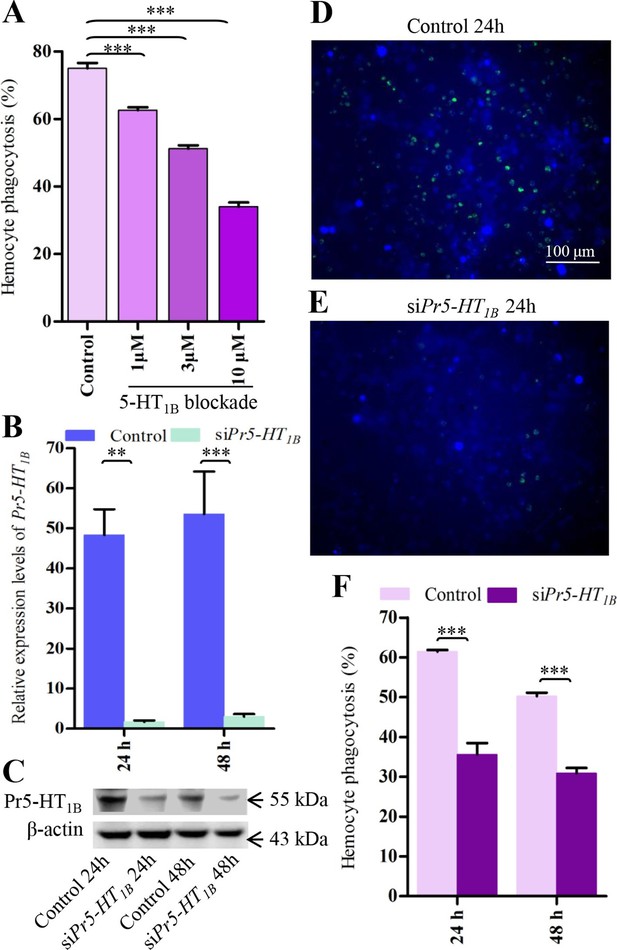
Pr5-HT1B mediates hemocyte phagocytosis.
(A) Dose-response profiles for the effects of Pr5-HT1B blocker SB 216641 on hemocyte phagocytosis. Data are from three independent experiments that each consists of cells from ten fifth-instar larvae. (B) Confirmation of knock-down effect of Pr5-HT1B by real-time PCR. The P. rapae 18s rRNA gene was used as an internal reference gene (n = 4). (C) Western blot analysis of knock-down effect of Pr5-HT1B. β-actin was used to show equal protein loading. (D-E) Effect of siPr5-HT1B on hemocyte phagocytosis was visualized by florescence microscope. (F) Quantification of siPr5-HT1B effect on hemocyte phagocytosis. Data are from three independent experiments that each consists of cells from ten fifth-instar larvae. One-way ANOVA followed by Tukey’s multiple comparison test for A; two-tailed t-test for B and F. Error bars indicate ± s.e.m., ***p<0.001, **p<0.01.
Effect of immune challenge on Pr5-HT1B and Pr5-HT2B expression
Although the above data showed that activation of Pr5-HT1B and Pr5-HT2B produced opposite effects, enhanced hemocyte phagocytosis is the overall effect by hemocytes exposed to released 5-HT. Thus, we tested whether 5-HT receptors were upregulated or downregulated in hemocytes during an immune response. qPCR results showed that the mRNA levels of Pr5-HT1B was significantly increased after LPS stimulation from 15 min to 4 hr (Figure 5A). The protein expression levels also increased at 2 hr and 4 hr (Figure 5C). Interestingly, the mRNA expression level of Pr5-HT2B was significantly decreased after LPS induction at 1 hr and 4 hr (Figure 5B), in accordance with its protein expression levels (Figure 5D).
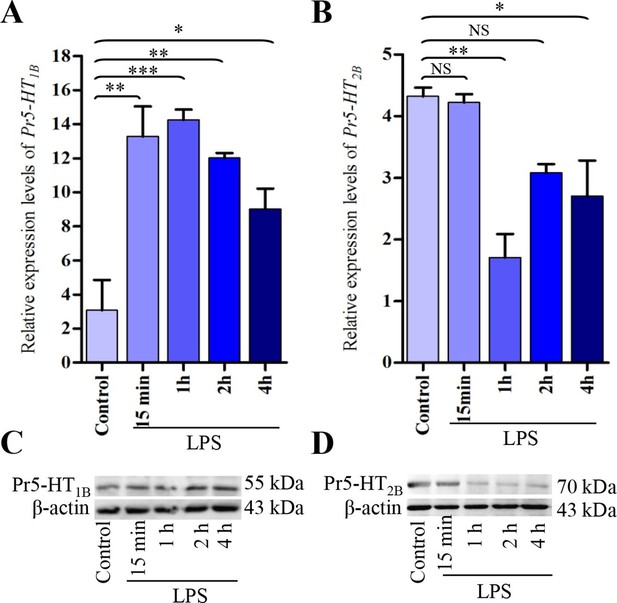
Expression analysis of Pr5-HT1B and Pr5-HT2B in naïve and LPS-induced hemocytes.
(A–B) Relative expression of Pr5-HT1B and Pr5-HT2B were quantified by q-PCR. The P. rapae 18s rRNA gene was used as an internal reference (n = 3). (C–D) Western blot analysis of Pr5-HT1B and Pr5-HT2B in naive and LPS-induced hemocytes. β-actin was used to show equal protein loading. One-way ANOVA followed by Tukey’s multiple comparison test for A and B. Error bars indicate ± s.e.m., ***p<0.001, **p<0.01, *p<0.05, and NS means no significant difference.
5-HT1B and 5-HT2B are required for proper bacteria phagocytosis and resistance in Drosophila
We hypothesized that 5-HT receptors mediate immunoregulation in other insects and that it is important in host defense in vivo. On the other hand, it is a technical challenge to perform in vivo RNAi in Lepidoptera (Terenius et al., 2011). Thus, we chose the model animal Drosophila melanogaster to address the above questions. Both 5-HT1B and 5-HT2B transcripts were detected in wild-type Drosophila hemocytes by RT-PCR. High mRNA levels of Henna, the Drosophila TPH homolog, were also expressed in hemocytes (Figure 6A). We next used a 5-HT1B null allele (5-HT1BΔIII-V) and its corresponding control allele (Gasque et al., 2013) to examine the ability to phagocytose bacteria as well as susceptibility to infections. A 1344 bp fragment is removed from genomic DNA to disrupt the III-V transmembrane domains of the 5-HT1BΔIII-V allele (Figure 6B).
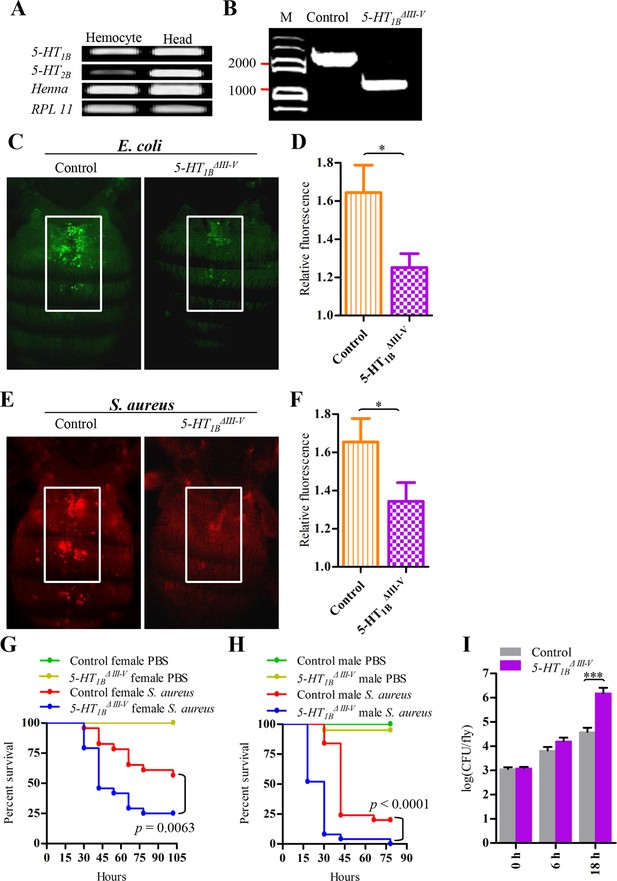
5-HT1B is required for microbial phagocytosis and plays an important role in the Drosophila defense against S. aureus infection.
(A) 5-HT1B, 5-HT2B and TPH are expressed in Drosophila naive hemocytes. RPL11 was used as an internal reference gene. Data are representatives of three independent experiments. (B) Genomic PCR of 5-HT1B control and 5-HT1BΔIII-V flies. Data are representatives of three independent experiments. (C) Representative pictures depicting phagocytosis in 5-HT1B control and 5-HT1BΔIII-V flies of fluorescein-labeled E. coli bioparticles. (D) Quantification of in vivo phagocytosis of E. coli. Approximately 10 flies per genotype were used in each experiment. Data are representatives of three independent experiments. (E) Representative pictures depicting phagocytosis in 5-HT1B control and 5-HT1BΔIII-V flies of fluorescein-labeled S. aureus bioparticles. (F) Quantification of in vivo phagocytosis of S. aureus. Approximately eight flies per genotype were used in each experiment. Data are representatives of three independent experiments. (G-H) Representative survival curves of female (G) and male (H) 5-HT1B control and 5-HT1BΔIII-V flies after injection of S. aureus (optical density [OD] 0.4). n = 20–25 flies. Experiments were performed in triplicate. (I) Comparison of the S. aureus (OD 0.4) recovered in 5-HT1B control and 5-HT1BΔIII-V flies 0, 6, and 18 hr post infection. Bacterial load was measured in eight individual female flies per genotype at each time point in each experiment. Two-tailed t-test for D, F and I. Error bars indicate ± s.e.m., ***p<0.001, **p<0.01, *p<0.05.
The phagocytic capacity of flies was measured using an in vivo adult phagocytosis assay (Kocks et al., 2005). Flies were injected with fluorescently labeled pHrodo bioparticles. The amount of fluorescence in the dorsal vessel area where sessile phagocytes accumulates were visualized and quantified. In 5-HT1BΔIII-V flies, in vivo phagocytosis of E. coli was strongly impaired when compared to controls (Figure 6C–D). A similar effect was observed with the Gram-positive bacterium S. aureus (Figure 6E–F). After infection with S. aureus, 5-HT1BΔIII-V flies die more quickly than controls, for both male and female flies (Figure 6G–H). To determine whether the increased mortality of 5-HT1BΔIII-V flies following infection was due to defective resistance or decreased tolerance (Schneider and Ayres, 2008), we also assessed bacterial clearance by comparing colony-forming units (CFU) 6 hr and 18 hr after infection. There is an increased bacterial load in 5-HT1B null flies compared to control files (Figure 6I).
5-HT1B function in the regulation of hemocyte phagocytosis was further confirmed by RNAi. We used the HmlΔ-Gal4 (Sinenko et al., 2004) driver to specifically express UAS-1B RNAi (Yuan et al. 2005) and UAS-5-HT1B RNAi25833 respectively, both of which led to decreased phagocytosis of E. coli and S. aureus (Figure 7A–D). To test whether the hemocyte-specific knockdown of 5-HT1B dampened phagocytosis in general, or whether there was a lack of inducibility after immune challenge, we injected flies with PBS or 5-HT and then latex beads. When flies were injected with PBS, all flies showed similar phagocytic capacity. However, when flies were injected with 5-HT, knocking down 5-HT1B failed to enhance latex beads phagocytosis as controls (Figure 7E). After injection with S. aureus, the flies expressing 5-HT1B RNAi in their blood cells were much weaker than the control flies (Figure 7F–I). Our RNAi data indicate that the increased susceptibility to bacteria in 5-HT1B mutants is due to 5-HT1B malfunction in hemocytes.
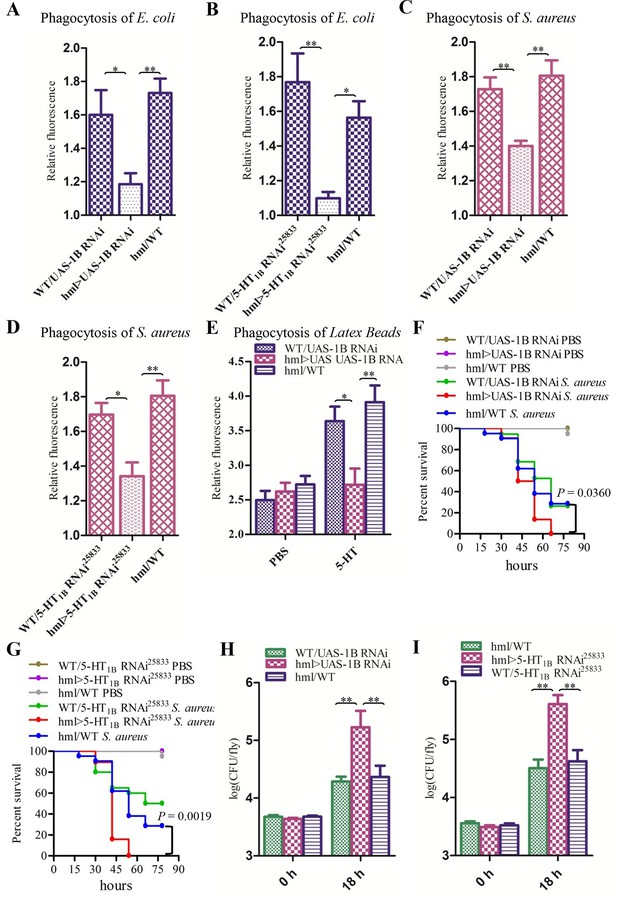
Knockdown of 5-HT1B in hemocytes affects Drosophila phagocytosis and survival.
(A) Quantification of in vivo phagocytosis of E. coli in WT/UAS-1B RNAi, hml> UAS-1B RNAi and hml/WT flies. Approximately six flies per genotype were used in each experiment. Experiments were performed twice, (B) Quantification of in vivo phagocytosis of E. coli in WT/UAS-5-HT1B RNAi25833, hml>UAS-5-HT1B RNAi25833, and hml/WT flies. Approximately six flies per genotype were used in each experiment. Experiments were performed twice. (C) Quantification of in vivo phagocytosis of S. aureus in WT/UAS-1B RNAi, hml> UAS-1B RNAi, and hml/WT flies. Approximately six flies per genotype were used in each experiment. Experiments were performed twice, (D) Quantification of in vivo phagocytosis of S. aureus in WT/UAS-5-HT1B RNAi25833, hml>UAS-5-HT1B RNAi25833, and hml/WT flies. Approximately six flies per genotype were used in each experiment. Experiments were performed twice. (E) Quantification of in vivo phagocytosis of red fluorescently labeled latex beads in WT/UAS-1B RNAi, hml>UAS-1B RNAi, and hml/WT flies after a 30 min preinjection of either PBS or 1μg/μl 5-HT. Approximately six flies per genotype were used in each experiment. Experiments were done twice, (F) Representative survival curves of WT/UAS-1B RNAi, hml>UAS-1B RNAi, and hml/WT male flies after injection of S. aureus. n=19–22 flies. Data are representatives of three independent experiments. Each experiment was performed in triplicate. (G) Representative survival curves of WT/UAS-5-HT1B RNAi25833, hml>UAS-5-HT1B RNAi25833, and hml/WT male flies after injection of S. aureus. n=19–21 flies. Data are representatives of two independent experiments. Each experiment was performed in triplicate. (H) Comparison of the S. aureus (OD 0.4) recovered in WT/UAS-1B RNAi, hml>UAS-1B RNAi and hml/WT flies 0, and 18 hr post infection. Bacterial load was measured in eight individual male flies per genotype at each time point in each experiment. Experiments were performed in triplicate. (I) Comparison of the S. aureus (OD 0.4) recovered in WT/UAS-5-HT1B RNAi25833, hml>UAS-5-HT1B RNAi25833, and hml/WT flies 0 and 18 hr post infection. Bacterial load was measured in eight individual male flies per genotype at each time point in each experiment. Experiments were performed in triplicate. One-way ANOVA followed by Tukey’s multiple comparison test for A, B, C, D, E, H, and I. Error bars indicate ± s.e.m., ***p<0.001, **p<0.01, *p<0.05.
To investigate the role of 5-HT2B in Drosophila hemocyte phagocytosis, we also knocked down 5-HT2B expression in blood cells by RNAi using two independent UAS-5-HT2B RNAi lines. Unlike the results found in P. rapae, the reduced levels of 5-HT2B in Drosophila hemocytes led to a defect in the phagocytosis of E. coli and S. aureus (Figure 8A–D). Moreover, the flies expressing 5-HT2B RNAi in their hemocytes took up significantly fewer latex beads than did control flies after 5-HT injection (Figure 8E). 5-HT2B knockdown flies had higher bacterial loads than controls (Figure 8I) and increased susceptibility to S. aureus (Figure 8E–G). Taken together, our experiments demonstrate that both 5-HT1B and 5-HT2B play important roles in host defense against bacterial infection.
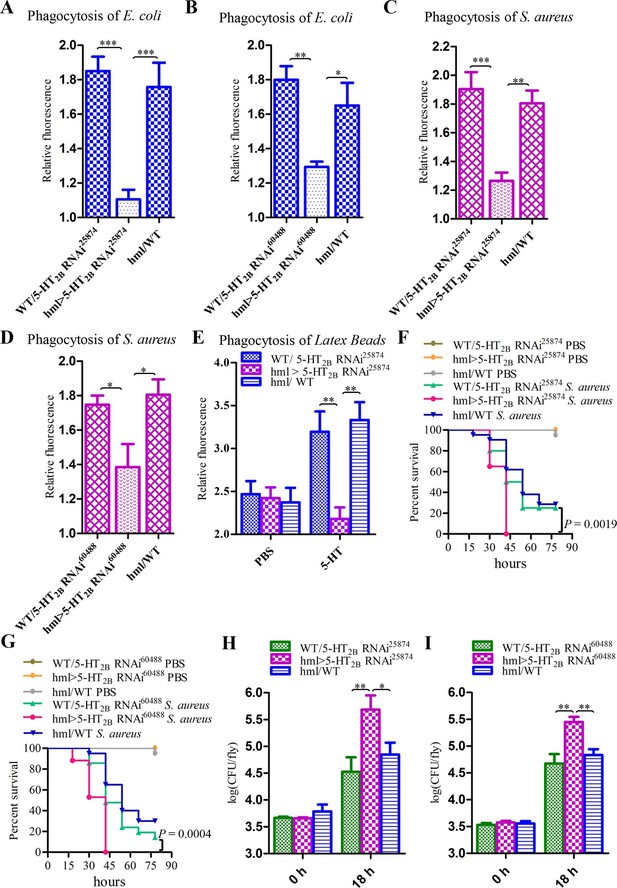
Knockdown of 5-HT2B in hemocytes affects Drosophila phagocytosis and survival.
(A) Quantification of in vivo phagocytosis of E. coli in WT/UAS-5-HT2B RNAi25874, hml>UAS-5-HT2B RNAi25874, and hml/WT flies. Approximately six flies per genotype were used in each experiment. Experiments were performed twice, (B) Quantification of in vivo phagocytosis of E. coli in WT/UAS-5-HT2B RNAi60488, hml>UAS-5-HT2B RNAi60488, and hml/WT flies. Approximately six flies per genotype were used in each experiment. Experiments were performed twice. (C) Quantification of in vivo phagocytosis of S. aureus in WT/UAS-5-HT2B RNAi25874, hml>UAS-5-HT2B RNAi25874, and hml/WT flies. Approximately six flies per genotype were used in each experiment. Experiments were performed twice, (D) Quantification of in vivo phagocytosis of S. aureus in WT/UAS-5-HT2B RNAi60488, hml> UAS-5-HT2B RNAi60488, and hml/WT flies. Approximately six flies per genotype were used in each experiment. Experiments were performed twice. (E) Quantification of in vivo phagocytosis of red fluorescently labeled latex beads in WT/UAS-5-HT2B RNAi25874, hml> UAS-5-HT2B RNAi25874, and hml/WT flies after a 30 min preinjection of either PBS or 1μg/μl 5-HT. Approximately six flies per genotype were used in each experiment. Experiments were done twice. (F) Representative survival curves of WT/UAS-5-HT2B RNAi25874, hml> UAS-5-HT2B RNAi25874, and hml/WT male flies after injection of S. aureus. n=20–21 flies. Data are representatives of two independent experiments. Each experiment was performed in triplicate. (G) Representative survival curves of WT/UAS-5-HT2B RNAi60488, hml>UAS-5-HT2B RNAi60488, and hml/WT male flies after injection of S. aureus. n=19–21 flies. Data are representatives of two independent experiments. Each experiment was performed in triplicate. (H) Comparison of the S. aureus (OD 0.4) recovered in WT/UAS-5-HT2B RNAi25874, hml>UAS-5-HT2B RNAi25874, and hml/WT flies 0, and 18 hr post infection. Bacterial load was measured in eight individual male flies per genotype at each time point in each experiment. Experiments were performed in triplicate. (I) Comparison of the S. aureus (OD 0.4) recovered in WT/UAS-5-HT2B RNAi60488, hml>UAS-5-HT2B RNAi60488, and hml/WT flies 0, and 18 hr post infection. Bacterial load was measured in eight individual male flies per genotype at each time point in each experiment. Experiments were performed in triplicate. One-way ANOVA followed by Tukey’s multiple comparison test for A, B, C, D, E, H, and I. Error bars indicate ± s.e.m., ***p<0.001, **p<0.01, *p<0.05.
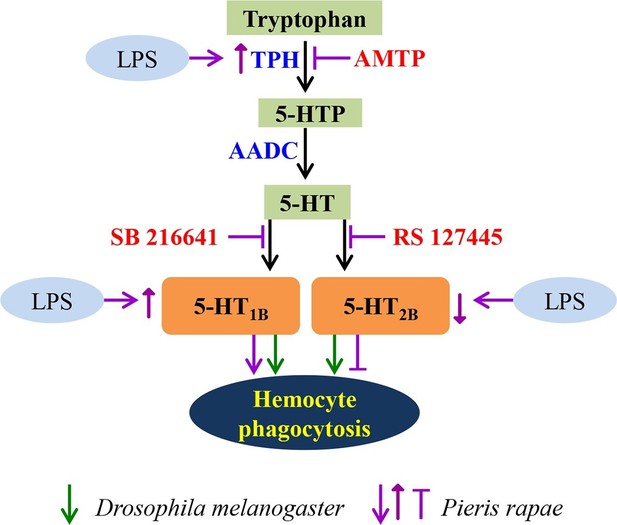
A schematic diagram of serotonin signaling on hemocyte phagocytosis.
LPS enhances the expression of TPH, which catalyzes tryptophan into 5-HT via 5-HTP. 5-HT, which secreted from hemocytes, activates the hemocyte-membrane receptor 5-HT1B and 5-HT2B. The immune responses of P. rapae are labeled in purple: activation of 5-HT1B promotes hemocyte phagocytosis and activation of 5-HT2B lead to opposite effects. LPS increases 5-HT1B expression but decreases that of 5-HT2B. The immune responses of Drosophila are labeled in green arrows: activation of 5-HT1B promotes hemocyte phagocytosis and activation of 5-HT2B lead to the same effects.
Discussion
Our study demonstrates the ubiquity of serotonergic receptor signaling pathway in immune function. This paper is the first to demonstrate that insect hemocytes express TPH and can synthesize 5-HT, like human macrophages (Nakamura et al., 2008). The exposure of hemocytes to LPS led to an induction of TPH expression and a release of 5-HT. Moreover, the secreted 5-HT appears to be an important autocrine stimulus. It promotes phagocytosis: inhibition of TPH either by a competitive inhibitor or siRNA silencing resulted in significantly decreased hemocyte phagocytosis.
Chemicals that act as neurotransmitters in the nervous system can also modulate immune function (Meredith et al., 2005). 5-HT is one of these classical neurotransmitters that is also an important immune regulatory molecule in both insects and mammals. Recent research has made progress in determining 5-HT modulated mammalian immune responses, especially regarding the machinery to produce, store, release, and respond to 5-HT in immune cells (Ahern, 2011). 5-HT was also reported to mediate immune responses, such as hemocyte phagocytosis, nodule formation and hemocyte population in insects (Baines et al., 1992; Kim et al., 2009; Kim and Kim, 2010), but the signaling pathway is unclear. Drosophila TPH homolog gene Henna was found to be one hit in a genome-wide RNAi screen for genes that affect phagocytosis of Candida albicans by hemocytes (Stroschein-Stevenson et al., 2006). Examining the basic interactions between serotonin receptor-medicated second messenger systems and immune-related intracellular signaling pathways in insects may shed light on their interactions and functions in mammals.
Second, our findings show that naive hemocytes express 5-HT1B, 5-HT2B, and 5-HT7 receptors, but only 5-HT1B and 5-HT2B appear to have functional roles. Using selective antagonists and RNAi, we found that inhibition of 5-HT1B decreases hemocyte phagocytosis. However, inhibition of 5-HT2B enhances hemocyte phagocytosis. It seems that activation of these two receptors affects hemocytes in opposite ways. Interestingly, we found that 5-HT1B is dramatically up-regulated following hemocyte activation, but 5-HT2B is significantly down-regulated. Therefore, even though the two receptors have opposite functions upon activation, the overall effects of 5-HT signaling induced by immune challenge are favorable for hemocyte phagocytosis (Figure 7). Mammalian DCs also express 5-HT1B and 5-HT2B, via which 5-HT induces chemotaxis and in vivo migration of DCs to draining lymph nodes (Müller et al., 2009). Even though insect plasmatocytes are macrophage-like cells, 5-HT activates macrophage cells via 5-HT1A (Nakamura et al., 2008) and 5-HT2C (Mikulski et al., 2010), which are different from the receptor sub-types found in insect hemocytes. The 5-HT7 receptor plays a critical role in the immune response in the gut of mice (Kim et al., 2013). Although our results indicated that 5-HT7 receptors were not involved in insect hemocyte phagocytosis, they may be critical for other immune activities.
Many neurotransmitter receptors are found on mammalian immune cells and regulate innate immune response, however, it is still unclear about their general role at the organismal level because most studies are conducted in vitro (Sternberg, 2006). However, it is relatively easy to test immunity in insects in vivo (Lemaitre and Hoffmann, 2007). We found that the 5-HT1B deficiency flies were more vulnerable to bacterial infections due to their poor phagocytic ability. Hemocyte-specific RNAi experiments showed similar results, indicating that the 5-HT1B-mediated hemocyte phagocytosis is important for insects to defend themselves against pathogens. Surprisingly, the flies expressing 5-HT2B RNAi in their hemocytes were more susceptible to bacterial infections, suggesting that activation of 5-HT2B may promote phagocytosis in Drosophila, different from the results in P. rapae.
In conclusion, we found that insect hemocytes can synthesis and release 5-HT, which regulates phagocytosis via 5-HT1B and 5-HT2B receptors on the membrane of the hemocyte. We also used the genetic model Drosophila to further confirm the roles of 5-HT1B and 5-HT2B in vivo. These findings suggest that serotonin, an ancient signaling molecule, modifies immune function in animals across phyla. Exploring the interactions between serotonin receptor-mediated pathways and immune-related pathways may be easier to initiate in insects, which have a simpler immune system.
Materials and methods
Insects
P. rapae larvae were collected primarily from cabbage fields in the experimental farmland of Zhejiang University, Hangzhou, China and the laboratory colony of P. rapae was reared at 25 ± 1°C and 70% relative humidity under a photoperiod of 14 hr light: 10 hr dark in a greenhouse as previously described by Zhang et al. (2005).
The following fly stocks were from the Bloomington Drosophila Stock Center: HmlΔ-Gal4 (30139); UAS-5-HT1B RNAi (27634, 25833); UAS-5-HT2B RNAi (25874, 60488). The 5-HT1B control and 5-HT1BΔIII-V flies were generous gifts from Leslie Vosshall (Gasque et al., 2013).
Reagents
Serotonin hydrochloride, forskolin, G418 disulfate salt and SB-269970 hydrochloride were obtained from Sigma-Aldrich (St Louis, MO). SB 216641 and RS 127445 were purchased from Tocris Bioscience (Bristol, UK).
Hemocytes isolation, culture and immune induction
Request a detailed protocolFifth-instar larvae of P. rapae were surface sterilized with 70% ethanol. The proleg was cut with a pair of scissors and the hemolymph was collected in Grace’s Insect Medium (1:10, v/v; Invitrogen, Carlsbad, CA). The diluted hemolymph was added to 12-well tissue culture plates (Nunc, Roskilde, Denmark) at a density of 2× 106 cells per well. Hemocytes were treated with 100 ng/ml LPS (Escherichia coli 0111:B4; Sigma Aldrich) for 15 min, 1 hr, 2 hr, and 4 hr (Ngkelo et al., 2012; Wu et al., 2015). The hemocyte culture was collected and centrifugated. The supernatants were collected for 5-HT detection. The hemocytes that adhered to the plate were harvested for RNA isolation.
5-HT detection
Request a detailed protocolThe hemolymph from three first day of fifth instar larvae was collected and mixed. The combined hemolymph (30 μl) was mixed with 170 μl Grace’s Insect Medium (Invitrogen) containing 50 μg/ml tetracycline and 2 μl saturated 2-phenylthiourea (PTU). The diluted hemolymph was added to each well of a 8-well chambered coverglasses (Lab-Tek, Nunc, Thermo Fisher Scientific, Rochester, USA) and hemocytes were allowed to adhere to the slide for 20 min at 27°C to form monolayers. Then, hemocytes were fixed with 4% paraformaldehyde. 5-HT was labeled with 5-HT antisera (72 hr, room temperature; S-5545; Sigma) followed by biotinylated anti- rabbit Ig (24 hr, 4°C) and SA-Alexa Fluor 546 (1 hr, room temperature; Invitrogen). For use as a negative control, 5-HT antisera was preabsorbed with 5-HT (10 mM, 24 hr, 4°C). The nuclei of hemocytes were stained with 1 μg/ml of 4′-6-diamidino-2-phenylindole (DAPI, Beyotime Biotech, Jiangsu, China) for 5 mins and hemocytes were observed by fluorescent microscope (Zeiss, Göttingen, Germany).
We performed ELISA to quantify the amount of 5-HT produced in the hemocyte culture supernatants using the 5-Hydroxytryptamine (serotonin ) assay kit (Li et al., 2014) (Jiancheng, Nanjing, China).
Cloning of 5-HT receptors, TRH and TPH
Request a detailed protocolTotal RNA was isolated from P. rapae nerve cord with Trizol reagent (Invitrogen, Carlsbad, CA, USA). Single-strand cDNA, synthesized from the RNA using a ReverTra Ace-α- kit (Toyobo, Osaka, Japan), was used as a template for PCRs. We performed transcriptome sequencing of nerve cord of P. rapae. Through transcriptome sequencing, several putative serotonin receptors were annotated using BlastX (National Center for Biotechnology Information [NCBI], Bethesda, MD). The full length was obtained using the 5’-Full rapid-amplification of cDNA ends (RACE) Kit (Takara, Dalian, China) and 3’-Full RACE Kit (Takara, Dalian, China) (Supplementary file 1A). To amplify the complete sequence, we use the forward primer located upstream of the putative start codon initiator, and the reverse primer located downstream of the putative stop codon (Supplementary file 1A).
qPCR and RT-PCR
Request a detailed protocolTotal RNA was extracted from P. rapae hemocytes with high pure RNA isolation kit (Roche) in accordance with the manufacturer’s instructions. To collect RNA from larval fly blood cells, approximately 30 larvae were carefully lacerated with tweezers on their anterior end in 100 μl of nuclease-free water. The RNA quantity and quality was measured by using a Nanodrop 2000 spectrophotometer (Thermo Scientific Inc., Bremen, Germany). Reverse transcription was performed with 1 μg of RNA by using a ReverTra Ace qPCR RT kit (Toyobo, Osaka, Japan). For conventional reverse transcription- polymerase chain reaction (RT-PCR) cDNA was amplified using KOD- Plus- (Toyobo, Osaka, Japan). Real-time quantitative PCR was performed on cDNA preparations using the SsoFast Eva Green Supermix with Low Rox (Bio-Rad, Hercules, CA) and Applied Biosystems 7500 Real-Time PCR System (Applied Biosystems by Life Technologies, Carlsbad, CA) following the manufacturer’s instructions. The quantification of transcript level of different gene was conducted according to the 2−ΔΔCT method (Livak and Schmittgen, 2001). Comparable quantities of cDNA were ensured by amplification of 18s rRNA, as a stably expressed reference gene (Wu et al., 2013) in P. rapae. The primers are listed in Supplementary file 1A.
Phagocytosis assay
Hemocyte phagocytosis assays in vitro
Request a detailed protocolThe assay for phagocytosis was performed according to the method described by Cuttell et al., (2008), with minor modifications. After isolation of hemocytes, they were prepared in a 96-well tissue culture plate (CoStar, Washington). Cells were incubated for 1 hr counterstained with 20 μM Cell Tracker Blue CMAC (Molecular Probes) (Life Technologies, Carlsbad, CA) and then washed in PBS. 5 μl of the drug solution were mixed with 45 μl of Grace’s medium were added into each well for 30 min. Bacterial phagocytosis assays using pHrodo E. coli (Invitrogen, Carlsbad, CA) were performed according to the manufacturer’s instructions. The proportion of cells that had phagocytosed labeled E. coli was determined under a florescence microscope (Nikon Eclipse TS100, Nikon, Japan) at 200 × in five different fields.
Fly phagocytosis assays in vivo
Request a detailed protocolTo assay E. coli and S. aureus phagocytosis in adults, approximately eight to ten flies with an equal distribution of females and males per genotype were injected with ~0.2 μl of 1 mg/mL pHrodo green E. coli or pHrodo red S. aureus (Invitrogen, Carlsbad, CA) using a FemtoJet microinjection system (Eppendorf). They were then incubated for 1 hr at room temperature. Fluorescently labeled particles were visualized through the cuticle using a florescence microscope (Nikon AZ100M, Nikon, Japan). We use Image J software to quantify the results. Relative fluorescence calculated as: [fluorescence]dorsal vein area/[fluorescence]adjacent area.
To assay phagocytosis of beads, flies were first injected with approximately 36.8 nl PBS or 1 μg/μl 5-HT dissolved in PBS using a Drummond Scientific Nanoject II. Flies were incubated at room temperature for 30 min and then injected with approximately 27 nl of 1.0 μm Red Fluorescent Carboxylate Modified FluoSpheres diluted 1: 2 (Invitrogen), incubated at room temperature for 10 min, injected with 36.8 nl 0.4% Trypan blue (Invitrogen), and then mounted and visualized as described above.
Hemocytes RNAi
Request a detailed protocolSmall interfering RNA (siRNA) molecules were designed from the nucleotide sequence obtained from P. rapae using Invitrogen siRNA design software (http://rnaidesigner.thermofisher.com/rnaiexpress/) (Supplementary file 1B) and were synthesized by Invitrogen. siRNA for the negative control was used in transfection experiments as a control. Hemocytes were isolated as described above and were attached to the surface of 96-well tissue culture plates and incubated in Grace’s medium. The monolayers of hemocytes were treated with 2.4 ng siRNA and 0.7 μl siRNA transfection reagent INTERFERin (Polyplus-transfection SA, France) according to the instructions from the manufacturer, and incubated at 27°C.
Polyclonal antibody preparation
Request a detailed protocolWe use the forward primer Pr5-HT1B- BamHI 5′- CGGGATCCCAAACAGCTAGGAAAAGAAT -3′ and the reverse primer Pr5-HT1B- XholI 5′- CCCTCGAGTTATGTCTTTGCCGCTTTCC -3′ to amplify the third intracellular loop cDNA fragment of Pr5-HT1B. Purified PCR products was cloned into vector pET-28a (+) and confirmed by DNA sequencing. The expression product was 17 kDa which carried a His-tag. The construct was used to transform Escherichia coli BL21 (DE3) and the cells inoculated into 2 l of Luria–Bertani (LB) medium containing kanamycin (50 mg/ml) at 37°C. Until A600 reached 0.8, the cultures were added with 0.5 mM isopropyl-β-d-thiogalactopyranoside (IPTG) and incubated overnight at 28°C. The cells were collected by centrifugation and disrupted by sonication. The insoluble recombinant protein was purified through Ni-chelating affinity column (TransGen Biotech, Beijing, China) under denaturing conditions. To confirm the identity of the recombinant protein, proteins were separated by SDS-PAGE, transferred to PVDF (Bio-Rad) and detected with an anti-His polyclonal antibody HRP (HuaAn Biotechnology, Hangzhou, China) conjugate by ECL (Thermo Scientific). Then the purified protein was submitted to Hangzhou HuaAn Biotechnology Company as an antigen for immunization in male New Zealand rabbits. The antibody to Pr5-HT1B was purified from antiserum which had been done by the company.
Polyclonal antibody against Pr5-HT2B was generated by Hangzhou HuaAn Biotechnology Company using peptide antigen for antibody development. Epitopes are predicted by GenScript Optimum Antigen design tool. Peptide sequence synthesized for Pr5-HT2B antibody development is SAAAKTSKGTNISEC. The amino acid cysteine (C), which locates at the end of the peptide, is used for carrier protein conjugation.
Western blot and immunofluorescence
Request a detailed protocolHemocytes were lysed in ice-cold buffer (1% Triton X-100, 150 mM NaCl, 10 mM Tris-HCl pH 7.5, 5 mM EDTA, 1 mM sodiumo-vanadate) containing protease inhibitors phenylmethyl sulfonylfluoride (PMSF, Sangon Biotech, P0754, Shanghai, China). For membrane proteins, debris was sedimented by centrifugation (15,500 g, 10 min, 4°C), supernatants were collected and their protein concentration was determined by Bradford reagent (Sangon Biotech, Shanghai, China). Samples were diluted in 4 × Protein SDS PAGE Loading Buffer (Takara Biotechnology, Japan), then boiled for 10 min. Samples were separated in a denaturing polyacrylamide gel and transferred to a PVDF membrane. After blocking (5% Tris-buffered saline; pH 7.0; containing 0.1% Tween 20) and washing, membranes were then incubated overnight with primary antibodies against Pr5-HT1B and Pr5-HT2B (1: 500). Membranes were then incubated with secondary antibody horseradish peroxidase–conjugated goat anti-rabbit IgG diluted 1:5,000 in Tris-buffered saline with Tween-20. Membranes were rinsed five times with wash buffer and then incubated with the ECL western blotting substrate (Promega, Wisconsin). Membranes were stripped and reprobed with anti-β- actin (Cell Signaling Technology, Beverly, MA) for 2 hr at room temperature, followed by incubation with secondary antibody.
Immunofluorescence was performed using the same methods as used for 5-HT detection except that the primary antibody was different.
Construction of expression plasmids
Request a detailed protocolAn expression plasmid containing the Kozak consensus sequence (Kozak, 1987) was constructed by PCR with specific primers (Supplementary file 1A). The PCR product was double digested. The digested DNA fragments were purified by PCR Clean-Up Kit (Axygen, Union City, CA) and then subcloned into pcDNA3.0 vector (Invitrogen). The correct insertion was confirmed by DNA sequencing.
HEK 293 cell culture, transfection, and creation of stable cell lines
Request a detailed protocolHuman Embryonic Kidney 293 (HEK 293) cells were obtained from the Cell Bank of Type Culture Collection of Chinese Academy of Sciences (Shanghai, China). The cell line identity has been authenticated by short tandem repeat (STR) profiling, and the cells were free from the mycoplasma contamination. HEK-293 cells were grown in Dulbecco’s modified Eagle’s medium (D-MEM) (Gibco BRL, Gaithersburg, MD) supplemented with 10% fetal bovine serum (FBS) (Gibco BRL) and antibiotics at 37°C and 5% CO2. After transfection of plasmid into the cells using Lipofectamine 2000 (Invitrogen), the antibiotic G418 (0.8 mg/mL) was added to the medium to select for cells that stably expressed the receptors. After 2 weeks of G418 selection, G418- resistant colonies were trypsinized in cloning cylinders and transferred to 12-well plastic plates for expansion. These individual cell lines were analyzed for integration of the receptor DNA by RT-PCR and localization of the protein by immunofluorescence (data not shown). The clonal cell line that most efficiently expressing 5-HT receptor was chosen for this study.
cAMP assay
Request a detailed protocolIntracellular cAMP concentration ([cAMP]i) was determined as previously described (Huang et al., 2007). Cells were plated into 12-well tissue culture plates (Nunc, Roskilde, Denmark) at a density of 1× 106 cells per well and incubated at 37°C with 10% CO2 in a humidified incubator. The cells were pre-incubated in Dulbecco’s phosphate-buffered saline (DPBS; Gibco-Invitrogen) containing 100 uM phosphodiesterase inhibitor IBMX for 20 min at room temperature. After the preincubation, a 50-μl aliquot of D-PBS containing various concentrations of reagents was added. The culture was then incubated for 20 min at room temperature. The reaction was stopped by aspirating the solution and then adding 250 μl ice-cold cell lysis buffer immediately to lysis the cells. The cell lysate was scraped into 1.5 ml Eppendorf tubes for collections and stored at –70°C until use. The solution was centrifugated and [cAMP]i in the supernatant was determined using a cAMP ELISA kit (R&D Systems, Minneapolis, MN).
Ca2+ imaging
Request a detailed protocolIntracellular calcium concentration ([Ca2+]i) was estimated and analyzed with a calcium imaging system. Fresh hemocytes from fifth-instar larvae were seeded on the coverslip with Grace’s medium and incubated for 30 min at 27°C. Then, the cells were loaded with the fluorescent probe Fura 2-AM (Dojindo Laboratories, Kumamoto, Japan) using 0.2% Cremophor EL (Sigma–Aldrich) for 30 min at 27°C. The cells were subsequently washed twice with a bathing solution (152 mM NaCl, 5.4 mM KCl, 5.5 mM glucose, 1.8 mM CaCl2, 0.8 mM MgCl2, and 10 mM HEPES, pH 7.4). The coverslips were transferred to a microscopic chamber that was constantly perfused with the bathing solution at ≈ 2 ml/min (Huang et al., 2012). The fluorescence at 510 nm by excitation at 340 or 380 nm with a xenon lamp was measured with individual cells using an Easy Ratio Pro calcium imaging system (Photon Technology International, Birmingham, NJ). Each experiment was repeated three times or more. EC50 values were estimated by fitting to a dose-dependent curve in Origin Pro (Origin Lab, Northampton, MA).
Survival following infection
Request a detailed protocolThe tetracycline-resistant S. aureus was grown overnight in a shaking incubator at 37ºC shaking at 225 rpm. Cultures were spun down. The resuspended cells were diluted in sterile PBS to achieve an optical density (OD) of 0.4. Five to seven days post-eclosion, flies were injected with 32.2 nl of the bacterial resuspension using a Drummond Scientific Nanoject II. Flies were kept at 25°C. Flies that died within 6 hr were considered dead and were removed from the count. Flies were transferred every day to new food and death was recorded every 12 hr.
Bacterial load
Request a detailed protocolThe tetracycline-resistant S. aureus was cultured in LB broth overnight at 37ºC shaking at 225 rpm, and subcultured to an OD of 0.4. Approximately 24 female flies per genotype were injected with 23 nl of the bacterial suspension. Eight flies from each group were then immediately homogenized in individual tubes with 100 μl sterile PBS. The material was serially diluted to 1:10, twice, in sterile PBS, and then plated on LB plates containing tetracycline. After 6 and 18 hr of infection at 25°C, eight additional flies from each genotype per time point were assayed as above with the exception that each sample was serially diluted 1:10 five times. The plates were incubated at 37°C for 8 to 10 hr. Bacterial colonies were counted when the colonies remain small and discrete.
Statistical analysis
Request a detailed protocolData had a normal distribution and are expressed as means ± standard error (s.e.m.). Data were analyzed using analysis of variance (ANOVA) with Tukey-Kramer post-hoc test and Student t tests. Log rank tests were used to determine whether survival curves of male flies were significantly different from one another. Gehan-Breslow-Wilcoxon test were used to determine whether survival curves of female flies were significantly different from one another. All curve fitting and statistical calculations were performed with Origin 8.0 (Origin Lab, Northampton, MA) and GraphPad Prism 5.0 (San Diego, CA).
References
-
Norepinephrine and octopamine: linking stress and immune function across phylaInvert Surv J 5:12–19.
-
Mechanisms of phagocytosis in macrophagesAnnual Review of Immunology 17:593–623.https://doi.org/10.1146/annurev.immunol.17.1.593
-
5-HT and the immune systemCurrent Opinion in Pharmacology 11:29–33.https://doi.org/10.1016/j.coph.2011.02.004
-
A Dialogue between the Immune System and Brain, Spoken in the Language of SerotoninACS Chemical Neuroscience 4:48–63.https://doi.org/10.1021/cn300186b
-
Cutting edge: serotonin is a chemotactic factor for eosinophils and functions additively with eotaxinJournal of Immunology 173:3599–3603.
-
Disruption of the nonneuronal tph1 gene demonstrates the importance of peripheral serotonin in cardiac functionProceedings of the National Academy of Sciences of the United States of America 100:13525–13530.https://doi.org/10.1073/pnas.2233056100
-
Alpha-methyltryptophan: effects on cerebral monooxygenases in vitro and in vivoJournal of Neurochemistry 24:89–95.
-
Innate immune responses of a lepidopteran insect, Manduca sextaImmunological Reviews 198:97–105.https://doi.org/10.1111/j.0105-2896.2004.0121.x
-
Octopamine and 5-hydroxytryptamine mediate hemocytic phagocytosis and nodule formation via eicosanoids in the beet armyworm, Spodoptera exiguaArchives of Insect Biochemistry and Physiology 70:162–176.https://doi.org/10.1002/arch.20286
-
At least six nucleotides preceding the AUG initiator codon enhance translation in mammalian cellsJournal of Molecular Biology 196:947–950.https://doi.org/10.1016/0022-2836(87)90418-9
-
Clinical correlates of blood serotonin levels in patients with mastocytosisEuropean Journal of Clinical Investigation 38:953–958.https://doi.org/10.1111/j.1365-2362.2008.02047.x
-
Human mast cells are capable of serotonin synthesis and releaseJournal of Allergy and Clinical Immunology 119:498–499.https://doi.org/10.1016/j.jaci.2006.09.003
-
Insect hemocytes and their role in immunityInsect Biochemistry and Molecular Biology 32:1295–1309.https://doi.org/10.1016/S0965-1748(02)00092-9
-
The Host Defense of Drosophila melanogasterAnnual Review of Immunology 25:697–743.https://doi.org/10.1146/annurev.immunol.25.022106.141615
-
Additive antidepressant-like effects of fasting with imipramine via modulation of 5-HT2 receptors in the miceProgress in Neuro-Psychopharmacology and Biological Psychiatry 48:199–206.https://doi.org/10.1016/j.pnpbp.2013.08.015
-
Serotonin activates murine alveolar macrophages through 5-HT2C receptorsAJP: Lung Cellular and Molecular Physiology 299:L272–L280.https://doi.org/10.1152/ajplung.00032.2010
-
Role of Serotonin in the Immune System and in Neuroimmune InteractionsBrain, Behavior, and Immunity 12:249–271.https://doi.org/10.1006/brbi.1998.0532
-
Role of a Serotonin Precursor in Development of Gut MicrovilliThe American Journal of Pathology 172:333–344.https://doi.org/10.2353/ajpath.2008.070358
-
Compartmentalization of neuronal and peripheral serotonin synthesis in Drosophila melanogasterGenes, Brain and Behavior 6:756–769.https://doi.org/10.1111/j.1601-183X.2007.00307.x
-
Serotonin Transporters – Structure and FunctionJournal of Membrane Biology 213:101–110.https://doi.org/10.1007/s00232-006-0878-4
-
Two ways to survive infection: what resistance and tolerance can teach us about treating infectious diseasesNature Reviews Immunology 8:889–895.https://doi.org/10.1038/nri2432
-
Serotonin is necessary for place memory in DrosophilaProceedings of the National Academy of Sciences of the United Staes of America 105:5579–5584.https://doi.org/10.1073/pnas.0710168105
-
Neural regulation of innate immunity: a coordinated nonspecific host response to pathogensNature Reviews Immunology 6:318–328.https://doi.org/10.1038/nri1810
-
Plasma membrane monoamine transporters: structure, regulation and functionNature Reviews Neuroscience 4:13–25.https://doi.org/10.1038/nrn1008
-
Evolutionary ancient roles of serotonin: long-lasting regulation of activity and developmentActa Neurobiol Exp 56:619–636.
-
Molecular and cellular analyses of a ryanodine receptor from hemocytes of Pieris rapaeDevelopmental & Comparative Immunology 41:1–10.https://doi.org/10.1016/j.dci.2013.04.006
Article and author information
Author details
Funding
Ministry of Science and Technology of the People's Republic of China (2013CB127600)
- Jia Huang
National Natural Science Foundation of China (31572039)
- Jia Huang
National Natural Science Foundation of China (31321063)
- Gong-yin Ye
The funders had no role in study design, data collection and interpretation, or the decision to submit the work for publication.
Acknowledgements
This work was supported by National Program on Key Basic Research Projects (2013CB127600), National Natural Science Foundation of China (31572039) and China National Science Fund for Innovative Research Groups of Biological Control (31321063). We thank Leslie Vosshall (The Rockefeller University) for sharing 5-HT1B control and 5-HT1BΔIII-V flies and Yu Yun-song (College of Medicine, Zhejiang University) for providing the bacterial strain Staphylococcus aureus. We thank Shelley Adamo (Dalhousie University) for her helpful comments on the manuscript.
Copyright
© 2016, Qi et al.
This article is distributed under the terms of the Creative Commons Attribution License, which permits unrestricted use and redistribution provided that the original author and source are credited.
Metrics
-
- 3,480
- views
-
- 891
- downloads
-
- 55
- citations
Views, downloads and citations are aggregated across all versions of this paper published by eLife.
Citations by DOI
-
- 55
- citations for umbrella DOI https://doi.org/10.7554/eLife.12241