Cryo-EM: Protein complexes in focus
The development of the first electron microscopes in the 1930s made it possible to observe cell structures that could not be seen with traditional light microscopes. However, it has only recently become possible to use electron microscopes to study the structural details of individual protein complexes and other macromolecules (Kühlbrandt, 2014). Improvements in the technique that have made this breakthrough possible – a technique known as single-particle cryo-electron microscopy (cryo-EM; Doerr, 2016) – are a very welcome addition to the other structural tools used in biochemistry and cell biology.
One of the advantages of cryo-EM over other electron microscopy techniques is that samples do not need to be exposed to chemical stains to prepare them for imaging. This means that fragile structures are less likely to undergo modifications before imaging. Unfortunately, if the image of an unstained sample is completely in focus, its features are practically invisible. Microscopists traditionally get around this problem by defocusing the image enough to see the transparent details (Adrian et al., 1984; Taylor and Glaeser, 1976). While out-of-focus images of transparent objects are, paradoxically, far better than in-focus ones, the contrast in such images (that is, the relative difference between the light and dark areas) provides only a ghostly representation of the object being studied. Now, in eLife, Radostin Danev and Wolfgang Baumeister of the Max Planck Institute of Biochemistry show that it is possible to generate highly detailed maps of large protein complexes using images that are in focus (Danev and Baumeister, 2016).
Light microscopy suffered from the same problem until Fritz Zernike discovered that an optical device called a quarter-wave phase plate could be used to produce high-contrast images of all the features in a transparent object, even for in-focus images (Figure 1; Zernike, 1955). While phase plates are easy to produce for light microscopes, making phase plates for electron microscopes has proved to be very difficult. Designs for such phase plates were proposed as early as 1947 (Boersch, 1947), but progress has been slow. 15 years ago, for example, Danev and Kuniaki Nagayama made a phase plate by using a focused-ion beam to drill a tiny hole in a thin carbon film (Danev and Nagayama, 2001; Danev and Nagayama, 2008). However, prolonged use led to a phenomenon known as “charging”, which introduced unwanted phase shifts into the electron beams.
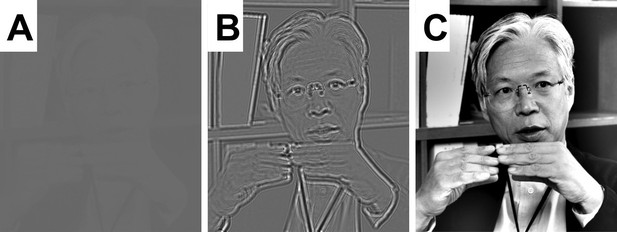
Simulations showing how a quarter-wave phase plate improves the contrast of in-focus images.
In these simulations the intensity of the beam (which can be made of light or electrons) remains the same everywhere it passes though the “transparent" object; however, the phase changes significantly where the beam passes through different parts of the object. (A) A perfectly focused, bright-field image has almost no contrast because it can only show variations in the transmitted intensity. (B) A defocused image is able to capture at least a small part of the changes in the phase, so the contrast is better and the object can be seen. (C) Zernike realized that changing the phase of the unscattered portion of the transmitted wave (that is, the part that was not deflected when the wave passed through the object) by 90 degrees greatly improved the quality of the image. The scientist in the photograph is Professor Kuniaki Nagayama, who has played a seminal role in the development of phase plates for use in the transmission electron microscope. Image credit: Dr. Radostin Danev.
A major breakthrough finally happened when Danev and colleagues discovered that charging could itself be exploited to generate the 90 degree phase shift needed in a quarter-wave phase plate (Danev et al., 2014). This new 'Volta' phase plate is already rapidly transforming the field of electron cryo-tomography (Danev et al., 2014; Mahamid et al., 2016).
Now, Danev and Baumeister go on to explore the potential uses of the Volta phase plate in single-particle cryo-EM. The first results are extremely exciting: a three-dimensional density map of a bacterial 20 S proteasome at a resolution of 3.2 Å! This protein complex, which plays a crucial role in degrading old and unwanted proteins, has been previously studied using a variety of structural biology techniques. The fact that the new results agree well with these previous findings is an important first step in testing the new phase plate.
I am personally optimistic that this technology will soon lead to equally good results for protein complexes that have proven to be difficult to study with existing techniques. Similarly, the increased signal-to-noise ratio that the phase plate provides ought to make it possible to obtain highly-detailed structures from many more proteins than is possible now, including proteins as small as, or even smaller than, hemoglobin.
However, Danev and Baumeister identify a challenge that will need to be addressed. To reconstruct the structure of a protein complex, many cryo-EM images need to be merged together using image processing software. Correcting for each image being out-of-focus by a (slightly) different amount is currently a standard part of image processing in cryo-EM, but this is much trickier to do when images are taken as close as possible to being in focus. Although Danev and Baumeister took steps to collect each image as carefully as possible, I worry that some remaining uncertainty might limit the level of detail achieved when the images are processed.
One way to determine the focus more accurately would be to prepare samples on a thin, amorphous-carbon support film. One could then estimate the focus from looking at the support film in the images, although the support film would also increase the amount of noise in the images. This is a step that many researchers who use cryo-EM are unwilling to take. I thus imagine a door of opportunity will open to anyone who comes up with a better alternative.
References
-
Uber die Kontraste von Atomen im ElectronenmikroskopZeitschrift Fur Naturforschung A 2:615–633.
-
Transmission electron microscopy with Zernike phase plateUltramicroscopy 88:243–252.https://doi.org/10.1016/S0304-3991(01)00088-2
-
Single particle analysis based on Zernike phase contrast transmission electron microscopyJournal of Structural Biology 161:211–218.https://doi.org/10.1016/j.jsb.2007.10.015
-
Volta potential phase plate for in-focus phase contrast transmission electron microscopyProceedings of the National Academy of Sciences of the United States of America 111:15635–15640.https://doi.org/10.1073/pnas.1418377111
-
Methods for imaging weak-phase objects in electron microscopyReview of Scientific Instruments 84:111101.https://doi.org/10.1063/1.4830355
-
Electron microscopy of frozen hydrated biological specimensJournal of Ultrastructure Research 55:448–456.https://doi.org/10.1016/S0022-5320(76)80099-8
Article and author information
Author details
Publication history
Copyright
© 2016, Glaeser
This article is distributed under the terms of the Creative Commons Attribution License, which permits unrestricted use and redistribution provided that the original author and source are credited.
Metrics
-
- 3,802
- views
-
- 301
- downloads
-
- 3
- citations
Views, downloads and citations are aggregated across all versions of this paper published by eLife.
Download links
Downloads (link to download the article as PDF)
Open citations (links to open the citations from this article in various online reference manager services)
Cite this article (links to download the citations from this article in formats compatible with various reference manager tools)
Further reading
-
- Biochemistry and Chemical Biology
- Structural Biology and Molecular Biophysics
Liquid-liquid phase separation (LLPS) involving intrinsically disordered protein regions (IDRs) is a major physical mechanism for biological membraneless compartmentalization. The multifaceted electrostatic effects in these biomolecular condensates are exemplified here by experimental and theoretical investigations of the different salt- and ATP-dependent LLPSs of an IDR of messenger RNA-regulating protein Caprin1 and its phosphorylated variant pY-Caprin1, exhibiting, for example, reentrant behaviors in some instances but not others. Experimental data are rationalized by physical modeling using analytical theory, molecular dynamics, and polymer field-theoretic simulations, indicating that interchain ion bridges enhance LLPS of polyelectrolytes such as Caprin1 and the high valency of ATP-magnesium is a significant factor for its colocalization with the condensed phases, as similar trends are observed for other IDRs. The electrostatic nature of these features complements ATP’s involvement in π-related interactions and as an amphiphilic hydrotrope, underscoring a general role of biomolecular condensates in modulating ion concentrations and its functional ramifications.
-
- Biochemistry and Chemical Biology
- Structural Biology and Molecular Biophysics
Both immunoglobulin light-chain (LC) amyloidosis (AL) and multiple myeloma (MM) share the overproduction of a clonal LC. However, while LCs in MM remain soluble in circulation, AL LCs misfold into toxic-soluble species and amyloid fibrils that accumulate in organs, leading to distinct clinical manifestations. The significant sequence variability of LCs has hindered the understanding of the mechanisms driving LC aggregation. Nevertheless, emerging biochemical properties, including dimer stability, conformational dynamics, and proteolysis susceptibility, distinguish AL LCs from those in MM under native conditions. This study aimed to identify a2 conformational fingerprint distinguishing AL from MM LCs. Using small-angle X-ray scattering (SAXS) under native conditions, we analyzed four AL and two MM LCs. We observed that AL LCs exhibited a slightly larger radius of gyration and greater deviations from X-ray crystallography-determined or predicted structures, reflecting enhanced conformational dynamics. SAXS data, integrated with molecular dynamics simulations, revealed a conformational ensemble where LCs adopt multiple states, with variable and constant domains either bent or straight. AL LCs displayed a distinct, low-populated, straight conformation (termed H state), which maximized solvent accessibility at the interface between constant and variable domains. Hydrogen-deuterium exchange mass spectrometry experimentally validated this H state. These findings reconcile diverse experimental observations and provide a precise structural target for future drug design efforts.