Endocytosis: Recycling at synapses
Neurons use small molecules called neurotransmitters to communicate with each other at junctions known as chemical synapses. Neurotransmitter is stored inside small sacs called synaptic vesicles, and is released into the synaptic cleft of the synapse when a vesicle fuses with the cell membrane. This process, which is known as exocytosis, can release neurotransmitter in less than a millisecond. However, it takes much longer to retrieve fused vesicle membrane to make a new vesicle (Figure 1): the fast version of this endocytosis process typically takes seconds, whereas a slow mode of endocytosis takes tens of seconds. This means that if a neuron is continuously active for a long period of time, its pool of vesicles can be depleted. Studies of vesicle recycling are complicated because the various processes involved, including membrane retrival, vesicle refilling, and transport of vesicles to the sites of exocytosis (active zones), are interdependent (Figure 1; Hosoi et al., 2009; Hua et al., 2013).
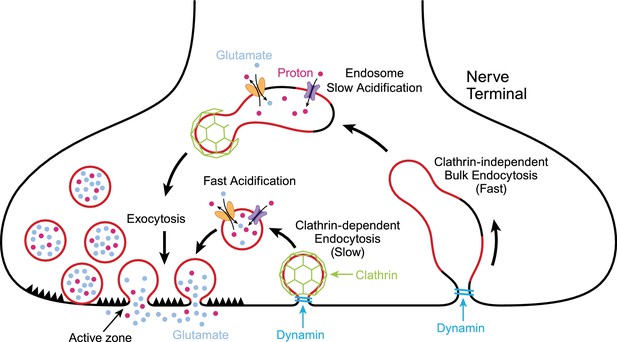
Exocytosis and endocytosis at nerve terminals.
During exocytosis, synaptic vesicles (red circles) fuse with the plasma membrane at active zones (left) to release neurotransmitter molecules (glutamate; pale blue) and protons (red dots) into the synaptic cleft. Synaptic vesicles that have fused to the plasma membrane are then recycled to make new vesicles in a process involving slow (middle) or fast (right) endocytosis. Synaptic vesicles are more acidic than the cytoplasm due to the action of pump proteins (purple) that load protons into the vesicles. Transport proteins (yellow) load glutamate into vesicles in exchange for protons. Slow endocytosis (middle) relies on a protein called clathrin (green), with membrane retrieval and acidification happening at approximately the same time. Fast endocytosis involves the production of large structures called endosomes that slowly become more acidic due to the action of proton pumps. New synaptic vesicles then bud from the endosome in a process that depends on clathrin. Both modes of endocytosis require dynamin (turquoise), a protein that pinches off the membrane.
Two techniques have been widely used to study vesicle recycling at synapses: patch clamping and fluorescent imaging. The patch clamp technique can be used to measure changes in the capacitance of the cell membrane and is a direct way to track membrane endocytosis (von Gersdorff and Matthews, 1994). Fluorescent imaging involves attaching pH-sensitive dyes to proteins in the vesicle membrane and recording how the fluorescence signal from the dye changes in response to fluctuations in pH (the inside of a vesicle is much more acidic than the cytoplasm and the environment outside the cell; Fernández-Alfonso and Ryan, 2004). When neurons are moderately stimulated, these two techniques report approximately the same time course, corresponding to the slow mode of endocytosis. However, stronger stimulation leads to conflicting results: patch clamp studies suggest that a fast mode of endocytosis becomes dominant, whereas fluorescent imaging reports a slowed time course for vesicle recycling.
Now, in eLife, Mitsuhara Midorikawa at Doshisha University and co-workers – including Yuji Okamoto as first author – report an elegant series of experiments where they used both patch clamping and fluorescent imaging at the same time to investigate vesicle recycling at a nerve terminal called the calyx of Held in rodents (Okamoto et al., 2016). Following moderate stimulation of the nerve terminal, patch-clamp experiments revealed the presence of both fast and slow modes of membrane endocytosis. However, fluorescent imaging revealed a delayed and slow time course for the pH change corresponding to the slower mode of endocytosis only. Nevertheless, both techniques reveal a significant block of endocytosis when small molecules that target the function of a critical protein called dynamin are introduced into the nerve terminal (Yamashita et al., 2005; Delvendahl et al., 2016).
When a stronger and prolonged stimulus was used, the fast form of endocytosis dominated according to membrane capacitance measurements, while the fluorescent signal reported almost no recovery of the acidic pH in vesicles for about 30 seconds after exocytosis. This crucial experiment reminds us that fluorescent imaging merely reflects the process by which the new vesicles are filled with protons (or re-acidification; see Figure 1), not the retrieval of membrane itself. Re-acidification might be much slower than membrane retrieval, particularly during fast endocytosis, which may be mediated by bulk endocytosis and the formation of transient endosomes that then bud off synaptic vesicles (Figure 1; de Lange et al., 2003; Watanabe et al., 2014). Ultimately, measuring membrane capacitance appears to be more reliable than fluorescent imaging as a tool for reporting synaptic vesicle membrane retrieval. Okamoto et al. also provide evidence that inhibiting a specific calcium-sensitive signaling pathway at active zones can prevent vesicle proteins from being taken up without affecting the retrieval of membrane. However, it is not clear whether this “decoupling” plays a biological role under physiological stimulation conditions.
Previous studies have shown that calcium ions both inhibit and promote endocytosis under various conditions (Hosoi et al., 2009; Leitz and Kavalali, 2011). The results of Okamoto et al. will be useful for designing experiments to clarify the distinct roles of calcium ions in regulating the different modes of endocytosis. Their approach could also be extended to use conditions that more closely match the normal activation patterns of neurons in the brain, where vesicle recycling happens very quickly at physiological temperatures (Delvendahl et al., 2016).
The slow mode of endocytosis depends on a protein called clathrin to make vesicles from the cell membrane or from endosomes (López-Murcia et al., 2014). Recently researchers in the UK observed a new role for clathrin in coordinating vesicle recycling in a ribbon-type chemical synapse on a faster time scale than seen previously (Pelassa et al., 2014). Further investigation is required to determine if this role for clathrin is specific to ribbon-type synapses, or whether it also applies to other types of synapses. Moreover, Pelassa et al. also found that the timing of the changes in the fluorescent signal and the membrane capacitance corresponded well with each other for a single brief stimulus condition. However, Okamoto et al. have demonstrated that there is much insight to be gained from studying strongly stimulated neurons where this correspondence breaks down.
References
-
Two modes of vesicle recycling in the rat calyx of HeldJournal of Neuroscience 23:10164–10173.
-
Ca2+ influx slows single synaptic vesicle endocytosisJournal of Neuroscience 31:16318–16326.https://doi.org/10.1523/JNEUROSCI.3358-11.2011
-
Presynaptic clathrin levels are a limiting factor for synaptic transmissionJournal of Neuroscience 34:8618–8629.https://doi.org/10.1523/JNEUROSCI.5081-13.2014
-
Synaptic vesicles are "primed" for fast clathrin-mediated endocytosis at the ribbon synapseFrontiers in Molecular Neuroscience 7:91.https://doi.org/10.3389/fnmol.2014.00091
Article and author information
Author details
Publication history
Copyright
© 2016, Gross et al.
This article is distributed under the terms of the Creative Commons Attribution License, which permits unrestricted use and redistribution provided that the original author and source are credited.
Metrics
-
- 13,603
- views
-
- 613
- downloads
-
- 13
- citations
Views, downloads and citations are aggregated across all versions of this paper published by eLife.
Download links
Downloads (link to download the article as PDF)
Open citations (links to open the citations from this article in various online reference manager services)
Cite this article (links to download the citations from this article in formats compatible with various reference manager tools)
Further reading
-
- Cell Biology
- Neuroscience
The assembly and maintenance of neural circuits is crucial for proper brain function. Although the assembly of brain circuits has been extensively studied, much less is understood about the mechanisms controlling their maintenance as animals mature. In the olfactory system, the axons of olfactory sensory neurons (OSNs) expressing the same odor receptor converge into discrete synaptic structures of the olfactory bulb (OB) called glomeruli, forming a stereotypic odor map. The OB projection neurons, called mitral and tufted cells (M/Ts), have a single dendrite that branches into a single glomerulus, where they make synapses with OSNs. We used a genetic method to progressively eliminate the vast majority of M/T cells in early postnatal mice, and observed that the assembly of the OB bulb circuits proceeded normally. However, as the animals became adults the apical dendrite of remaining M/Ts grew multiple branches that innervated several glomeruli, and OSNs expressing single odor receptors projected their axons into multiple glomeruli, disrupting the olfactory sensory map. Moreover, ablating the M/Ts in adult animals also resulted in similar structural changes in the projections of remaining M/Ts and axons from OSNs. Interestingly, the ability of these mice to detect odors was relatively preserved despite only having 1–5% of projection neurons transmitting odorant information to the brain, and having highly disrupted circuits in the OB. These results indicate that a reduced number of projection neurons does not affect the normal assembly of the olfactory circuit, but induces structural instability of the olfactory circuitry of adult animals.
-
- Neuroscience
Specialized chemosensory signals elicit innate social behaviors in individuals of several vertebrate species, a process that is mediated via the accessory olfactory system (AOS). The AOS comprising the peripheral sensory vomeronasal organ has evolved elaborate molecular and cellular mechanisms to detect chemo signals. To gain insight into the cell types, developmental gene expression patterns, and functional differences amongst neurons, we performed single-cell transcriptomics of the mouse vomeronasal sensory epithelium. Our analysis reveals diverse cell types with gene expression patterns specific to each, which we made available as a searchable web resource accessed from https://www.scvnoexplorer.com. Pseudo-time developmental analysis indicates that neurons originating from common progenitors diverge in their gene expression during maturation with transient and persistent transcription factor expression at critical branch points. Comparative analysis across two of the major neuronal subtypes that express divergent GPCR families and the G-protein subunits Gnai2 or Gnao1, reveals significantly higher expression of endoplasmic reticulum (ER) associated genes within Gnao1 neurons. In addition, differences in ER content and prevalence of cubic membrane ER ultrastructure revealed by electron microscopy, indicate fundamental differences in ER function.