Differences and similarities between human and chimpanzee neural progenitors during cerebral cortex development
Figures
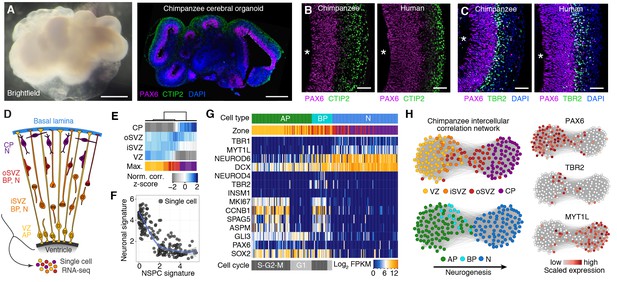
Chimpanzee cerebral organoids recapitulate cortex development.
(A) Bright-field image showing a representative chimpanzee organoid (Sandra A, left) and a cryosection from a chimpanzee organoid (PR818-5) immunostained for PAX6 (magenta) and Ctip2 (green) combined with DAPI staining (blue) (right) at day 52. Scale bars, 200 μm. (B, C) Cryosections of cortical regions from chimpanzee (Sandra A) and human (SC102A-1) organoids at day 52 immunostained for PAX6 (magenta), Ctip2 (B, green) and TBR2 (C, green), without (B) and with (C) DAPI staining (blue). Asterisks, ventricular lumen; scale bars, 50 μm. (D) Cartoon showing NSPC types (APs, BPs) and neurons enriched in zones within the neocortex at mid-neurogenesis. CP, cortical plate; N, neuron. (E) Heatmap showing normalized correlation (Z-score) of single-cell transcriptomes from chimpanzee cerebral organoid cortex with bulk RNA-seq data from laser-microdissected zones (Fietz et al., 2012) from 13 wpc human neocortex. CP, cortical plate. (F) Scatterplot showing NSPC and neuronal signature scores derived from analysis of fetal cerebral cortex single-cell transcriptomes (Figure 1—figure supplement 1) calculated for each chimpanzee cerebral organoid cortical cell. (G) Heatmap showing expression of AP, BP, and neuron (N) marker genes. Each column represents a single cell, each row a gene. Cell type and maximum correlation to bulk RNA-seq data from cortical zones are shown in the top sidebar. APs and BPs were sub-classified based on G1 (light grey) or S-G2-M (dark grey) phases of the cell cycle. (H) Lineage network based on pairwise correlations between chimpanzee cerebral organoid cortical cells reveals a structured topology where VZ-APs connect to cortical plate (CP) neurons (N) through SVZ-BPs. Cells are coloured based on cortical zone (top left) or cell type assignment (bottom left). APs, BPs, and neurons were classified based on maximum correlation with single-cell transcriptomes from the human fetal neocortex. Expression of markers PAX6, TBR2, and MYT1L are shown to the right.
-
Figure 1—source data 1
Processed single-cell RNA-seq data for chimpanzee cells.
*.txt file containing processed chimpanzee single-cell RNA-seq data (344 single cells) in log2(FPKM) with genes in columns and cells in rows. The first 7 columns contain metadata for each cell: cortex: assignment of cell to cortex (1) or to other regions within organoid(0); tSNE_1: tSNE1 loading for each cell; tSNE_2: tSNE2 loading for each cell; PC1: PC1 loading for each cell; PC2: PC2 loading for each cell; species: species of origin for each cell; cell_id: unique ID for each cell, with information about the experiment and the age of the organoid of origin for each cell.
- https://doi.org/10.7554/eLife.18683.004
-
Figure 1—source data 2
Genes describing cell populations in the chimpanzee organoids.
List of genes identified by PCA on all chimpanzee organoid single-cell transcriptomes as being most informative for defining cell populations.
- https://doi.org/10.7554/eLife.18683.005
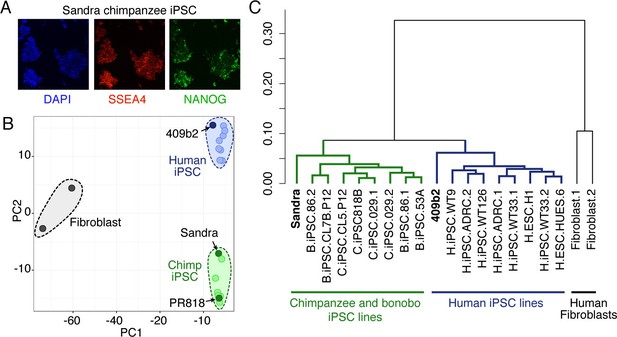
Characterization of chimpanzee iPSCs.
(A) Chimpanzee iPSC line Sandra A stained for pluripotency markers SSEA5 (red) and NANOG (Green). Nuclei are stained with DAPI. (B) PCA on bulk RNA-seq data from human iPSCs, chimpanzee and bonobo iPSCs, and human fibroblasts was used to describe the variation between cell types. RNA-seq data on chimp iPSC line Sandra A and human iPSC line 409b2 was generated in this study. Data from the other human, chimpanzee and bonobo, and fibroblast lines were previously published (Ma et al., 2014; Marchetto et al., 2013). (C) Dendrogram showing hierarchical clustering of human IPSC, chimpanzee and bonobo IPSC, and human fibroblast lines based on the Pearson correlation of the expression of 12,221 genes.
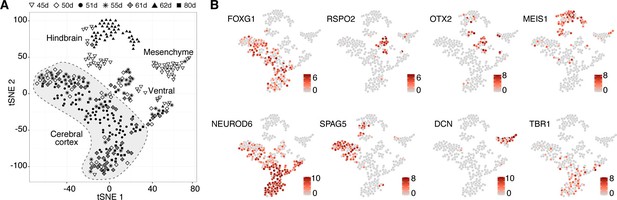
Deconstructing cell type composition in chimpanzee cerebral organoids using single-cell RNA-seq.
(A) scRNA-seq was performed on chimpanzee organoids dissociated at 45, 50, 51, 55, 62, and 80 days (d) after embryoid body (EB) culture. PCA and unbiased clustering using tSNE reveals cell populations from hindbrain, midbrain, mesenchyme, and cerebral cortex (shaded in grey) within organoids. Different symbols indicate different experiments. (B) Marker genes are shown for each cluster with cells coloured based on gene expression level. Cerebral cortex cells have high expression of FOXG1 and NEUROD6, and low expression of OTX2 and RSPO2. Progenitors express marker SPAG5. Cells are coloured based on expression level.
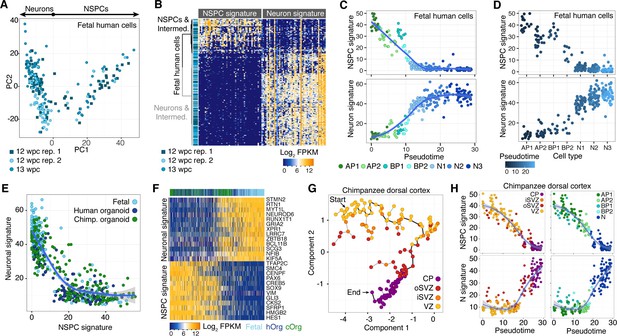
Fetal human progenitor and neuronal neocortical signatures are recapitulated in chimpanzee cerebral organoids.
(A) PCA of human fetal neocortex was used to identify genes describing cortical cell populations. Each dot represents a cell that is color-coded in shades of blue representing three different experiments. The genes correlating and anticorrelating with PC1 were used to define the NSPC and neuron signature, respectively. (B) Hierarchical clustering and heatmap visualization showing the expression of genes that have highest correlation (NSPC signature) and anti-correlation (Neuron signature) with PC1. Cells are shown in rows, genes in columns. (C,D) Fetal cortical cells were classified as APs in G2-M (AP1), APs in G1-S (AP2), BPs in G2-M (BP1), BPs in G1-S (BP2), or migrating (N1, N2) and cortical plate (N3) neurons. Each cell was scored for the NSPC (top) or neuron (bottom) signature and plotted in the order of pseudotemporal point on the neurogenic lineage (C) or plotted for each cell type (D). (E) Scatterplot showing NSPC and neuronal signature scores for each human fetal, human organoid and chimpanzee organoid cortical cell. The signatures were derived from PCA of fetal cerebral cortex single-cell transcriptomes. (F) Heatmap showing gene expression of top NSPC and neuron signature genes across human fetal, human organoid (hOrg), and chimpanzee organoid (cOrg) cells. (G) Monocle reveals a NSPC-to-neuron lineage in the chimpanzee organoid that correlates with the zones of the developing fetal primate neocortex. Cells (circles, coloured by maximum correlation with cortical zones; CP, cortical plate) are arranged in the 2-D independent component space based on genes identified using PCA. The minimal spanning tree (grey lines) connects cells, with the black line indicating the longest path. (H) Each chimpanzee cerebral organoid cortical cells scored for the NSPC (top) or neuron (N, bottom) signature and plotted in the order of pseudotemporal position on the neurogenic lineage. Cells are coloured by maximum correlation with cortical zones (left) or cell type (right).
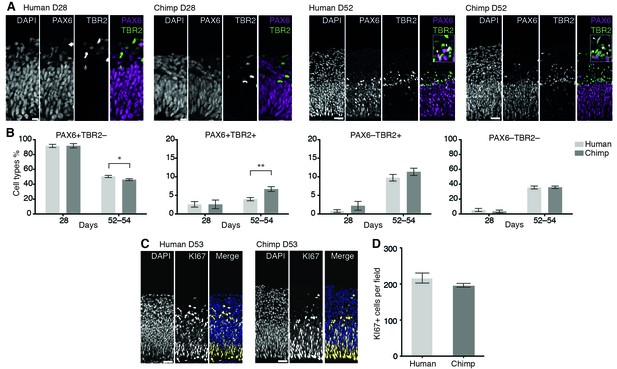
Changes in the proportion of cortical NSPC subtypes and neurons during human and chimpanzee cerebral organoid development.
(A) Cryosections of cortical regions from human and chimpanzee organoids at day 28 and day 52 immunostained for PAX6 (magenta) and TBR2 (green) combined with DAPI staining. Scale bars; D28, 10 μm; D52, 20 μm. Insets in the D52 merge images show selected areas with PAX6+TBR2+ double-positive nuclei (arrowheads) at higher magnification. (B) Quantification of the percentage of PAX6+TBR2–, PAX6+TBR2+, PAX6–TBR2+ and PAX6–TBR2– cortical cells in human (light grey) and chimpanzee (dark grey) organoids at D28 (n = 5 organoids, 50 μm wide field) and D52-D54 (n = 17 organoids, 100 μm wide field). Error bars, SEM; *p<0.05, **p<0.01. (C) Cryosections of cortical regions from human and chimpanzee organoids at D53 immunostained for KI67 (yellow) combined with DAPI staining (blue). Scale bars, 20 μm. (D) Quantification of KI67+ cells in a 100 μm wide field in human and chimpanzee organoids at D52-D53 (n = 7). Error bars, SEM.
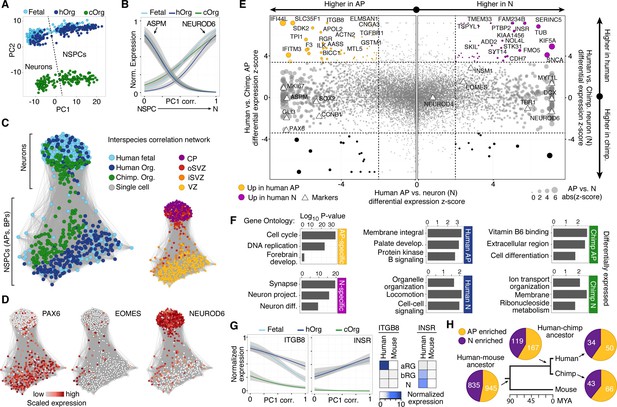
Comparing human and chimpanzee cerebral cortex gene expression.
(A) PC1 and PC2 from PCA separated NSPCs and neurons, and human and chimpanzee, respectively. PCA was performed on all single-cell transcriptomes using genes expressed in more than two cells and with a non-zero variance. (B) Quasibinomial fit line of representative marker gene expression across cells ordered by correlation with PC1. (C) Lineage network based on pairwise correlations between human fetal, human organoid, and chimpanzee organoid cells reveals a differentiation topology from VZ APs through BPs in iSVZ and oSVZ, to cortical plate (CP) neurons, with inter-species mixing in all stages. (D) Lineage network (see (C)) coloured by scaled expression level of marker genes. (E) Scatterplots showing z-scored significance estimates from single-cell differential expression (SCDE) analysis based on Bayesian probabilistic models. Reads from human and chimpanzee were mapped to a consensus genome, and human gene annotations were used for expression counting. The x-axis represents SCDE between human organoid APs vs. human organoid neurons. The y-axes on the left and right plots represent SCDE between human and chimpanzee APs and neurons (N), respectively. Genes coloured as white triangles represent marker genes from Figure 1 and are generally not differentially expressed between human and chimpanzee, but do vary between APs and neurons, validating the SCDE analysis. Yellow and purple circles represent genes upregulated specifically in human APs and neurons, respectively. Circles are sized based on differential expression between human APs and neurons. Figure 3—figure supplement 1 shows a similar plot from the chimpanzee perspective. (F) Gene ontology enrichments (-log10 P-value) for differentially expressed gene groups shown in panel E. Left, human APs (yellow) and neurons (N, purple) that are not differential between human and chimpanzee. Center, upregulated in human APs (top) or neurons (N, bottom) compared to chimpanzee. Right, upregulated in chimpanzee APs (top) or neurons (N, bottom) from Figure 3—figure supplement 1. (G) Left, expression profiles of ITGB8 and INSR are shown from human organoid, chimpanzee organoid, and human fetal cells ordered by correlation with PC1. Right, bulk RNA-seq data from sorted aRG, bRG, and neurons (N) from human and mouse developing neocortex (Florio et al., 2015) confirms enriched expression of ITGB8 and INSR in human APs and neurons, respectively. (H) The same bulk RNA-seq data was used to confirm and estimate the origin of differential gene expression in APs versus neurons from single-cell organoid data. Pie chart shows the proportion of AP-enriched (yellow) or neuron-enriched (N, purple) genes that are observed in human, chimpanzee, and mouse. Pie charts also show the proportion of genes differential between APs and neurons that are observed only in human and chimpanzee, but not mouse (human-chimp ancestor), or genes specific to human or chimpanzee.
-
Figure 3—source data 1
Processed single-cell RNA-seq data for human cells.
*.txt file containing processed human single-cell RNA-seq data (207 single cells) in log2(FPKM) with metadata in first 4 columns for each cell: cell_id: unique ID for each cell; experiment: the experiment during which each cell was isolated; species: species of origin for each cell; cortex: assignment of cell to cortex (1) or to other regions within organoid (0).
- https://doi.org/10.7554/eLife.18683.011
-
Figure 3—source data 2
Results of differential gene expression analyses.
Excel file (*.xlsx) with multiple sheets containing results of all differential expression analyses presented in the manuscript as well as GO enrichment analysis for the differentially expressed (DE) genes: Sheet 1: Genes specific to APs, not DE between chimpanzee and human; Sheet 2: GO enrichment analysis for genes of sheet 1; Sheet 3: Genes specific to Neurons, not DE between chimpanzee and human; Sheet 4: GO enrichment analysis for genes of sheet 3; Sheet 5: Genes specific to APs and upregulated to human compared to chimpanzee; Sheet 6: GO enrichment analysis for genes of sheet 6; Sheet 7: Genes specific to Neurons and upregulated to human compared to chimpanzee; Sheet 8: GO enrichment analysis for genes of sheet 7; Sheet 9: Genes specific to APs and upregulated to chimpanzee compared to human; Sheet 10: GO enrichment analysis for genes of sheet 6; Sheet 11: Genes specific to Neurons and upregulated to chimpanzee compared to human; Sheet 12: GO enrichment analysis for genes of sheet 11; Sheet 13: GO enrichment data used to generate Figure 3F.
- https://doi.org/10.7554/eLife.18683.012
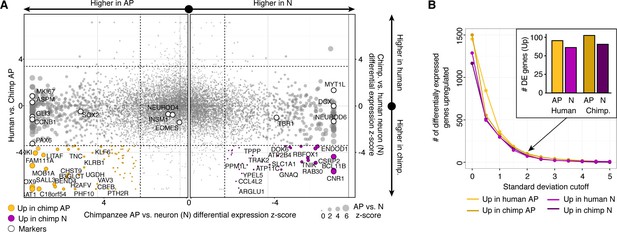
Differential expression analysis between chimpanzee and human cerebral cortex cells from the chimpanzee perspective.
(A) Scatterplots showing z-scored significance estimates from single-cell differential expression (SCDE) analysis based on Bayesian probabilistic models. Reads from human and chimpanzee were mapped to a consensus genome, and human gene annotations were used for expression counting. The x-axis represents SCDE between chimpanzee organoid APs vs. chimpanzee organoid neurons (N). The y-axes on the left and right plots represents SCDE between human and chimpanzee APs and neurons, respectively. Genes coloured as white circles represent marker genes from Figure 1 and are generally not differentially expressed between human and chimpanzee, but do vary between chimpanzee APs and neurons, validating the SCDE analysis. Yellow and purple circles represent genes upregulated specifically in chimpanzee APs and neurons, respectively. Circles are sized based on differential expression between chimpanzee APs and neurons. (B) Plot showing the number of differentially expressed genes between human and chimpanzee cells as a function of standard deviations above the mean z-score from the Bayesian differential gene expression analysis.
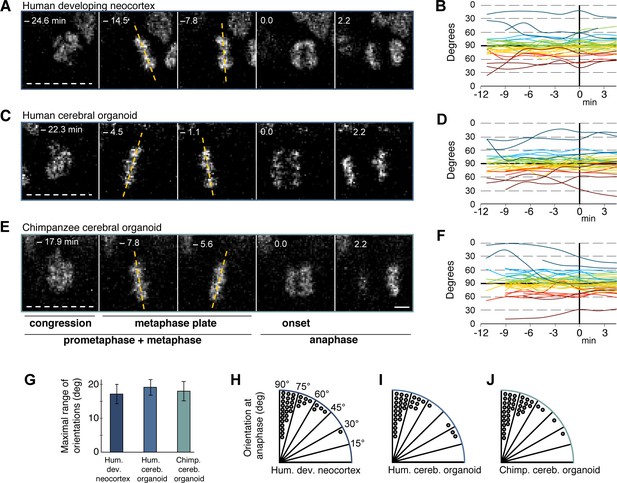
Spindle orientation variability is similar between APs of human developing neocortex, human organoids and chimpanzee organoids.
Live tissue imaging of spindle orientation, as reported by chromosome plate orientation, in organotypic slice culture of developing neocortex and cerebral organoids. Measurements were started after all chromosomes had formed a tight metaphase plate. 0 min is anaphase onset. Time-lapse is ∼1.1 min. (A,C,E) APs in a coronal slice of 13 wpc human frontal neocortex (A), in a slice of a D30 human cerebral organoid from iPSC line SC102A-1 (C), and in a slice of a D30 chimpanzee cerebral organoid from iPSC line Sandra A (E). The time indicated on each image is when that image was taken, relative to anaphase onset (0 min). White dashed lines, ventricular surface. Yellow dashed lines indicate the two metaphase plate orientations with the greatest difference to each other. Scale bar, 5 μm. (B,D,F) Quantification of all orientations of the chromosome plates from the beginning of the metaphase plate stage to anaphase, for APs in the three respective tissues described in (A,C,E). To facilitate tracing, individual tracks are colour-coded according to the initial range of the track, and the 90°−0° range is depicted twice (green and yellow, 90°−75°; cyan and red, 75°−60°; blue and dark red, 60°−0°; 90° indicates perfectly vertical chromosome plates). (G) Maximal range of chromosome plate orientations for APs, from the beginning of the metaphase plate stage to anaphase onset, as determined in the measurements shown in (B,D,F). Data are the mean ± SEM of ≥34 APs from 3 independent experiments each. (H,I,J) Orientation of chromosome plates at 2.2 min after anaphase onset, which indicates the predicted plane of cleavage, as determined in the measurements shown in (B,D,F). 90° indicates a perfectly vertical cleavage plane.
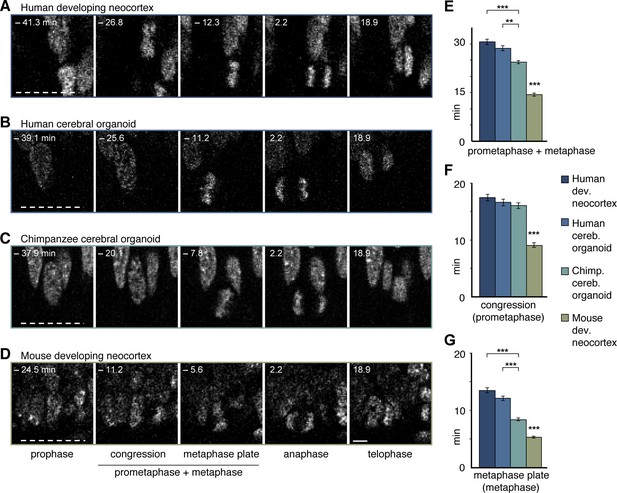
Differences in prometaphase-metaphase length between APs of human developing neocortex, human organoids, chimpanzee organoids and mouse developing neocortex.
Live tissue imaging of mitotic phases, as reported by chromosomes, in organotypic slice culture of developing neocortex and cerebral organoids. 0 min is anaphase onset. Time-lapse is ∼1.1 min. (A–D) APs in a coronal slice of 13 wpc human frontal neocortex (A), in a slice of a D30 human cerebral organoid from iPSC line SC102A-1 (B), in a slice of a D30 chimpanzee cerebral organoid from iPSC line Sandra A (C), and in a coronal slice of E14.5 mouse neocortex. The time indicated on each image is when that image was taken, relative to anaphase onset (0 min). White dashed lines, ventricular surface. Scale bar, 5 μm. (E–G) Time between the start of chromosome congression and anaphase onset (referred to as 'prometaphase + metaphase') (E), between the start of chromosome congression and the formation of a metaphase plate (referred to as 'prometaphase') (F), and between the formation of a metaphase plate and anaphase onset (referred to as 'metaphase') (G), for APs in the four tissues described in (A–D). Data include APs from 11–13 wpc human neocortex, organoids from the human iPSC lines SC102A-1 and 409b2, and chimpanzee iPSC lines Sandra A and PR818-5, and are the mean ± SEM of ≥60 APs from ≥4 independent experiments each. Bracket with **p<0.01; brackets with ***p<0.001; ***p<0.001 (mouse vs. all primate tissues).
-
Figure 5—source data 1
Durations of all mitotic phases.
Numerical values in minutes for the duration of all mitotic phases ± SEM used in the graphs in Figures 5, 6 and 7, in Figure 5—figure supplement 1, 2 and 3, and in Figure 6—figure supplement 1.
- https://doi.org/10.7554/eLife.18683.016
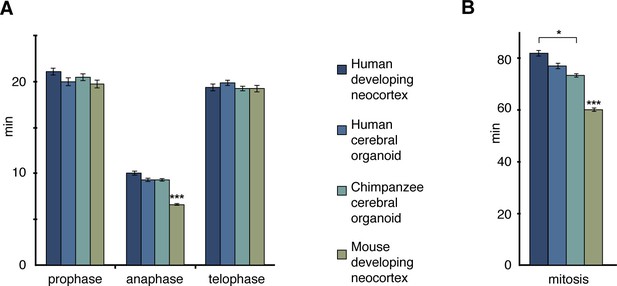
The length of the mitotic phases other than prometaphase-metaphase is similar between human and chimpanzee APs.
Length of prophase, anaphase and telophase (A), and of total mitosis (B, sum of all mitotic phases described here and in Figure 5) between APs of human developing neocortex, human and chimpanzee cerebral organoids and mouse developing neocortex, determined from the experiments described in Figure 5. Data are the mean ± SEM of ≥60 APs from ≥4 independent experiments each. *p<0.05; ***p <0.001.
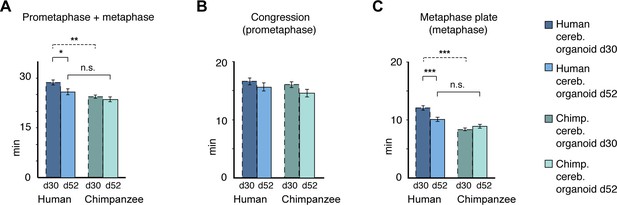
Differences in prometaphase-metaphase length between APs of D30 and D52 human and chimpanzee cerebral organoids.
Mitotic phase measurements similar to those in Figure 5E–G, but for APs in D52 organoids. Time between the start of chromosome congression and anaphase onset (referred to as 'prometaphase + metaphase') (A), between the start of chromosome congression and the formation of a metaphase plate (referred to as 'prometaphase') (B), and between the formation of a metaphase plate and anaphase onset (referred to as 'metaphase') (C). Data include APs from organoids from the human iPSC line SC102A-1 and chimpanzee iPSC line Sandra A, and are the mean ± SEM of 30 APs from 2 independent experiments each. For comparison, the relevant data for human and chimpanzee D30 cerebral organoid APs from Figure 5 are shown (dashed lines). *p<0.05; **p<0.01; ***p<0.001; n.s., not significant.
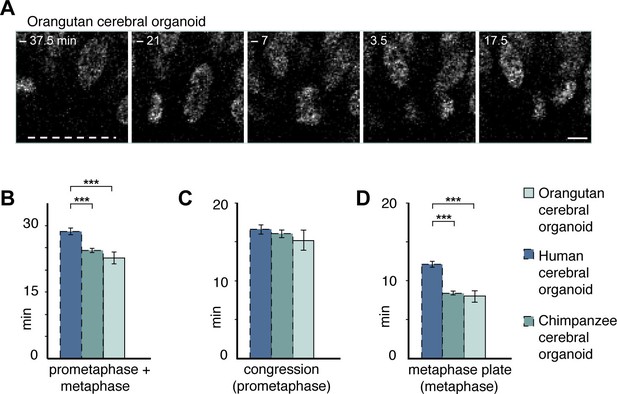
Prometaphase-metaphase in orangutan organoid APs is similar to chimpanzee organoid APs.
Live tissue imaging of mitotic phases, as reported by chromosomes, in organotypic slice culture of orangutan cerebral organoid. 0 min is anaphase onset. Time-lapse is ~3.5 min. (A) AP in a slice of orangutan D30 cerebral organoid (Toba). The time indicated on each image is when that image was taken, relative to anaphase onset (0 min). White dashed line, ventricular surface. Scale bar, 5 μm. (B–D) Time between the start of chromosome congression and anaphase onset (referred to as 'prometaphase + metaphase') (B), between the start of chromosome congression and the formation of a metaphase plate (referred to as 'prometaphase') (C), and between the formation of a metaphase plate and anaphase onset (referred to as 'metaphase') (D). For comparison, the relevant mitotic phase lengths of human and chimpanzee cerebral organoid APs from Figure 5 are shown (columns with dashed line). Data for orangutan are the mean ± SEM of 16 cells from 2 different cortex regions of an organoid. ***p<0.001.
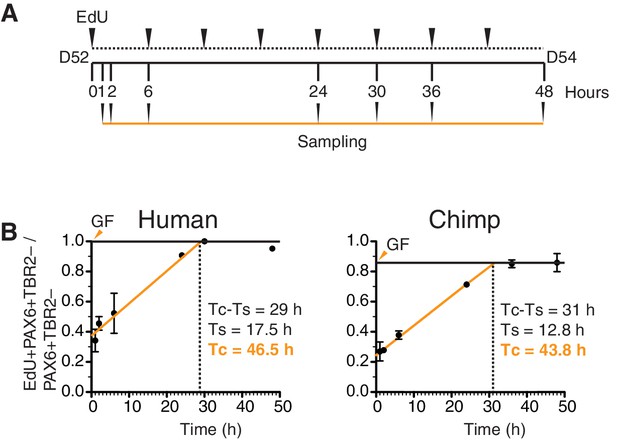
Determination of cell cycle parameters of human and chimpanzee organoid APs using cumulative EdU labeling.
(A) Schematic representation of the cumulative EdU labeling experiment. (B) Linear regression curves of human (r2 = 0.984) and chimpanzee (r2 = 0.998) PAX6+TBR2– cells after cumulative EdU labeling over 48 hr. The total cell cycle length minus the S-phase length (Tc-Ts) was obtained directly from the graph (vertical dashed lines). The S-phase length (Ts) was determined from the x-axis and y-axis intercepts of the linear regression curves, and the total cell cycle length (Tc) was calculated therefrom. The growth fraction (GF, solid horizontal line) is indicated by orange arrowheads.
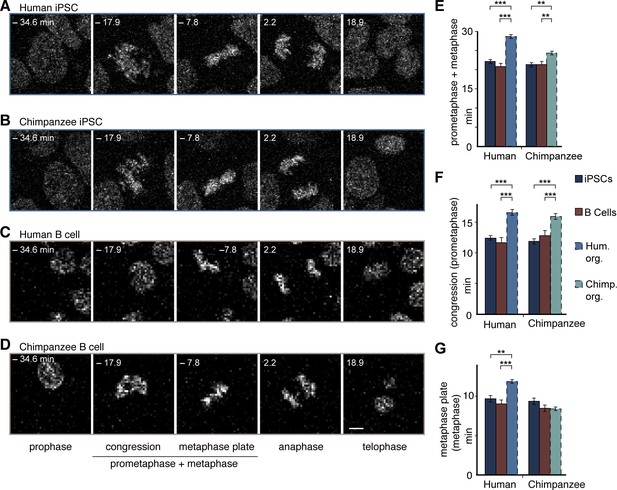
Human and chimpanzee organoid APs exhibit longer prometaphase, and human organoid APs longer metaphase, than their iPSC lines of origin or B cells.
Live imaging of mitotic phases, as reported by chromosomes, in human and chimpanzee iPSCs and B cells. 0 min is anaphase onset. Time-lapse is ∼1.1 min. (A–D) Human iPSC (SC102A-1) (A), chimpanzee iPSC (Sandra A) (B), human B cell (A158) (C), and chimpanzee B cell (Dorien) (D). The time indicated on each image is when that image was taken, relative to anaphase onset (0 min). Scale bar, 5 μm. (E–G) Time between the start of chromosome congression and anaphase onset (referred to as 'prometaphase + metaphase') (E), between the start of chromosome congression and the formation of a metaphase plate (referred to as 'prometaphase') (F), and between the formation of a metaphase plate and anaphase onset (referred to as 'metaphase') (G). Data include cells from each of the following iPSC lines: human, SC102A-1 and 409b2; chimpanzee, Sandra A and PR818-5; and from the following B cell lines: human, A144, A156 and A158; chimpanzee, Jahaga, Ulla and Dorien. For comparison, the relevant mitotic phase lengths of human and chimpanzee cerebral organoid APs from Figure 5 are shown (columns with dashed line). Data are the mean ± SEM of ≥30 cells from ≥3 independent experiments each. **p<0.01; ***p<0.001.
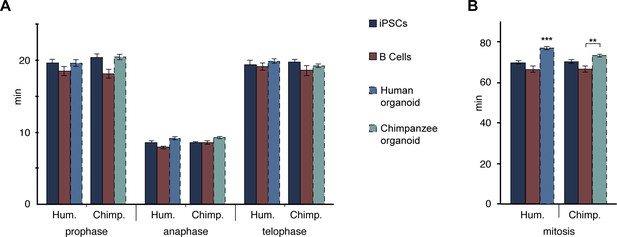
The length of prophase, anaphase and telophase is similar in human and chimpanzee iPSCs, B cells and organoid APs.
Length of prophase, anaphase and telophase (A), and of total mitosis (B, sum of all mitotic phases described here and in Figure 6) in human and chimpanzee iPSCs and B cells, determined from the experiments described in Figure 6. For comparison, the relevant mitotic phase lengths of human and chimpanzee cerebral organoid APs from Figure 5–figure supplement 1 are shown (columns with dashed line). Data are the mean ± SEM of ≥30 cells from ≥3 independent experiments each.
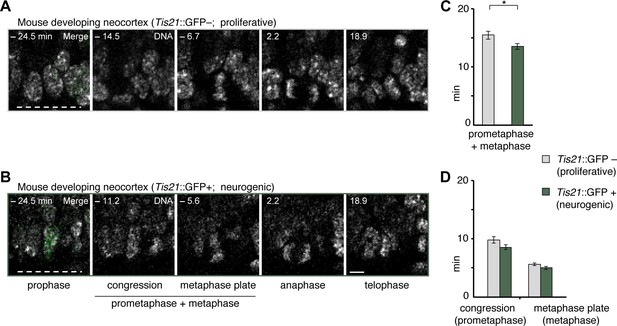
Prometaphase-metaphase is longer in proliferative than neurogenic mouse APs.
Live tissue imaging of mitotic phases, as reported by chromosomes, in organotypic slice culture of E14.5 mouse neocortex. 0 min is anaphase onset. Time-lapse is ~1.1 min. Data is from the same mouse dataset shown in Figure 5, but distinguishes between Tis21::GFP– (proliferative) and Tis21::GFP (neurogenic) APs. (A,B) APs in a coronal slice of mouse E14.5 dorsolateral telencephalon, either negative (A) or positive (B) for expression of Tis21::GFP. The time indicated on each image is when that image was taken, relative to anaphase onset (0 min). White dashed lines, ventricular surface. Scale bar, 5 μm. Image panels in (B) are the same as in Figure 5D, but the Tis21::GFP fluorescence (green) is included in the prophase image (merge). The GFP channel is also merged in the prophase image of (A), and the other panels are DNA staining only. (C,D) Length of prometaphase and/or metaphase in proliferative vs. neurogenic APs. Data are the mean ± SEM of 41 Tis21::GFP– and 37 Tis21::GFP APs from 4 independent experiments. *p<0.05. (C) Time between the start of chromosome congression and anaphase onset (referred to as 'prometaphase + metaphase'). (D) Time between the start of chromosome congression and the formation of a metaphase plate (referred to as 'prometaphase', left), and time between the formation of a metaphase plate and anaphase onset (referred to as 'metaphase', right).
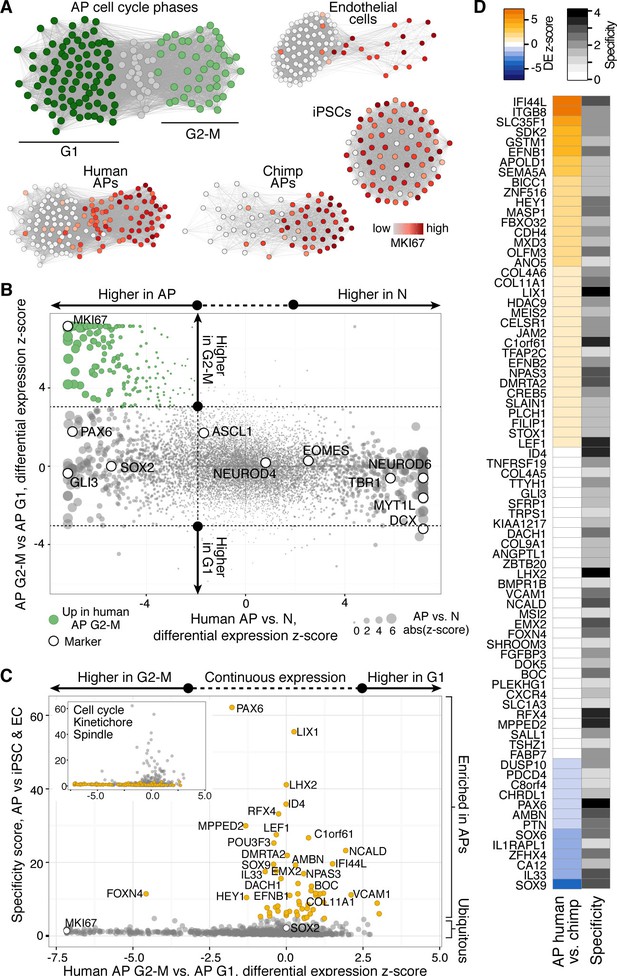
Differential gene expression during AP mitotic phases.
(A) G1 (dark green) and G2-M (light green) cell cycle phases were assigned to cells by performing PCA using genes involved in cell cycle regulation. PC1 and PC2 described cell cycle phases, and the top 50 correlating and anticorrelating genes were used to infer an intercellular correlation network for human and chimp APs, human iPSCs, and a human endothelial cell line. Networks are coloured based on the expression level of MKI67. (B) Scatterplot shows z-scored significance estimates from single-cell differential expression (SCDE) analysis between human organoid APs vs. neurons (N, x-axis) and APs in G2-M vs. APs in G1 (y-axis). Genes coloured as white circles represent marker genes and green circles represent genes upregulated specifically in APs in G2-M. Circles are sized based on differential expression between human APs and neurons. (C) iPSC and endothelial cell (EC) expression was used to assign a specificity score for genes enriched in human organoid APs compared to neurons (higher in AP genes from panel B). The specificity score is plotted against the differential expression between APs in G2-M and APs in G1. Cells with high AP specificity scores are in yellow in the main scatter plot. This shows that nearly all genes enriched in G2-M phase of the AP cell cycle are not specific to APs, but also enriched in G2-M of mitotic iPSCs and endothelial cells. (D) Heatmap shows the differential expression score between human and chimpanzee APs (z-score) and AP specificity score (Log2 normalized) of the same genes that are specific to APs relative to endothelial cells and iPSCs.
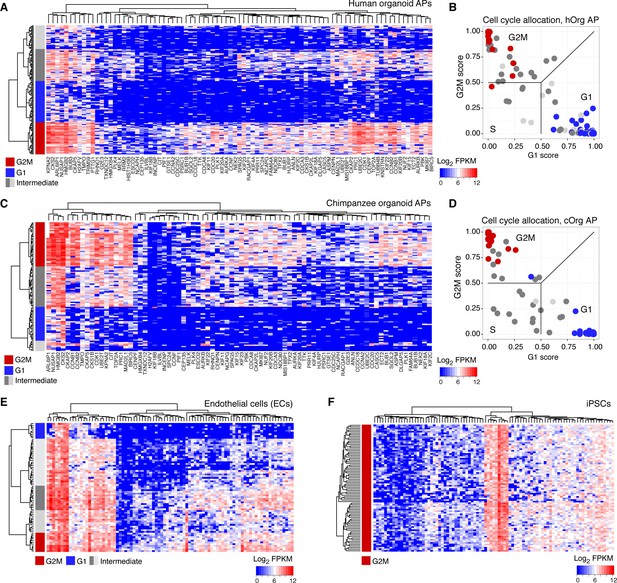
Cell cycle assignment for differential gene expression analysis.
(A) Hierarchical clustering was used to identify human organoid APs that most strongly expressed genes enriched in G2M phase of the cell cycle (red). The genes were identified from PCA on fetal cortex progenitor cells (top 100 correlating genes) (Camp et al., 2015). The cluster with weakest expression of these G2M associated genes was assigned as G1 phase (blue). Intermediate cells (grey) were discarded from differential gene expression analysis. (B) A previously published method was used to computationally assign cell-cycle stage based on a machine-learning approach (Scialdone et al., 2015). This method was generally consistent with our assignment based on the hierarchical clustering presented in panel A. (C–F) The same approach was used to identify the chimpanzee organoid APs, endothelial cells (ECs), and iPSCs that most highly express G2M markers. Note that all iPSCs analyzed highly expressed most of the G2M marker genes.
Videos
Differences in prometaphase-metaphase length between APs of human and chimpanzee cerebral organoids.
Related to Figure 5B and C Live tissue imaging of mitotic phases, as reported by chromosomes, in organotypic slice culture of cerebral organoids. Time-lapse is ∼1.1 min. Datasets are the same as in Figure 5B and C. Left side: APs in a slice of a D30 human cerebral organoid from iPSC line SC102A-1. Right side: APs in a slice of a D30 chimpanzee cerebral organoid from iPSC line Sandra A. Growing colour bars at the bottom indicate time progression of the respective dividing AP and are synchronized to the beginning of prometaphase (in green). Metaphase plate time is in yellow and anaphase time is in red. Note the slower progression of the dividing human AP on the left.