Neural Wiring: The circuitry of sex
Reproduction is widely considered to be the raison d’etre of life on Earth, and sex in the animal kingdom can take many forms. Some organisms – including worms and snails – are hermaphroditic and can self-fertilise to produce offspring (Leonard, 2006; Thomas et al., 2012). In other, perhaps more familiar, species, males and females mate to produce the next generation.
Given the importance of reproduction, it is not surprising that animals can devote much of their adult lives to, and employ all of their senses in, reproductive behaviours – whether that is seeking out mates and courting, or assessing the environment to see if it is a good time to reproduce. Moreover, cognitive processes such as memory, learning and decision-making are also harnessed to optimize reproductive success (Reif et al., 2002).
The vinegar fly Drosophila melanogaster is a model organism that shares its history and habitat with people (Keller, 2007). The mating habits of the fly have been closely observed in both the wild and the laboratory (Markow, 2015; Villella and Hall, 2008). What we know is: a male vinegar fly will court a female and, if she so chooses, they will mate. The male first uses his genital claspers to couple his genitals to those of the female. Next, the penis and associated branches emerge from his genital region and are inserted into her vaginal area as they copulate (Kamimura, 2010). Sex between vinegar flies typically lasts about 20 minutes, with the transfer of sperm and ejaculate being completed after about 9 minutes (Gilchrist and Partridge, 2000). When mating is finished, the male withdraws, and the claspers release.
The male’s penis is controlled by the coordinated interaction of about ten muscles in his genital region (Kamimura, 2010). However, until recently, relatively little was known about the way these muscles were controlled by the nervous system. Now, in eLife, Stephen Goodwin, Hania Pavlou and co-workers, at the University of Oxford, the National Institute of Mental Health and McGill University, report that they have identified the neural circuits that coordinate copulation in male vinegar flies (Pavlou et al., 2016).
Based on previous findings, Pavlou et al. knew that the neurons controlling copulation in male flies would be located in the fly’s nerve cord, which is equivalent to the spinal cord in humans (Hall, 1979; Ferveur and Greenspan, 1998). They looked for a population of neurons that expressed both a gene called doublesex and a neurotransmitter called glutamate. They did this because doublesex is an important gene that generates differences in the anatomy and behaviour of males and females, and because glutamate is associated with the motor neurons that instruct muscles to move (Rideout et al., 2010; Daniels et al., 2008). Also, doublesex-positive neurons were already known to control, amongst other things, the courtship songs of male vinegar flies (Rideout et al., 2007; Rideout et al., 2010; Shirangi et al., 2016).
About 80 such neurons were found in the male flies. Further experiments showed that when these neurons were triggered prior to copulation, the males would court but not copulate. On the other hand, when these neurons were triggered during copulation, the males would not terminate the act and separate. Based on these findings, Pavlou et al. suggested that these neurons control the muscles that contract or relax to move the penis during copulation. They also identified a set of interneurons that suppress these 80 or so motor neurons. These interneurons expressed both doublesex and the neurotransmitter called GABA.
The motor neurons and interneurons form the wires of a simple circuit that regulates the muscles (Figure 1). But one more type of neuron was required to complete the circuit: an input. Pavlou et al. found these missing connections in the form of sensory neurons linked to hair-like bristles positioned near the penis. These neurons express doublesex and the neurotransmitter called acetylcholine, and they project to the interneurons, motor neurons and brain.
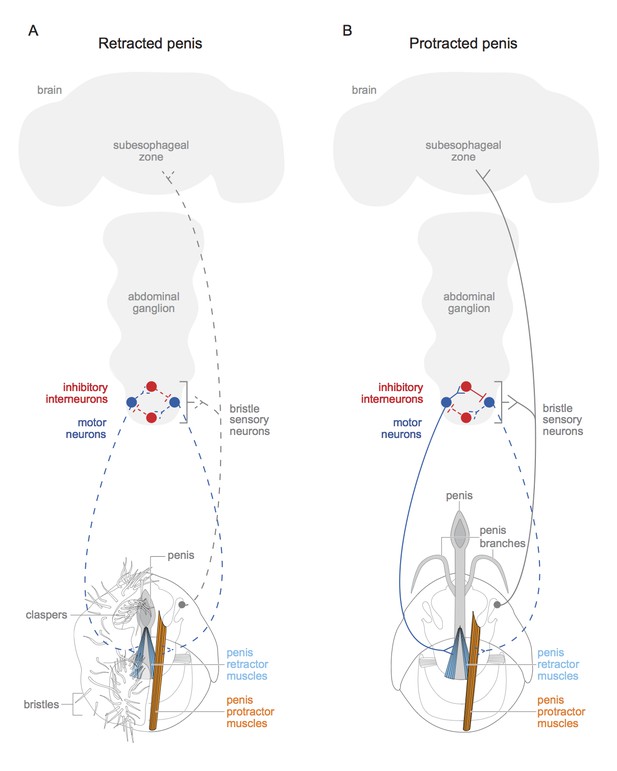
Schematic of motor circuit that controls the penis in male vinegar flies.
The penis of the male vinegar fly is controlled by protractor muscles (shown in orange) and retractor muscles (light blue). (A) The penis will be retracted if all of the doublesex-positive motor neurons (blue) are inactive (indicated by dashed lines), or if neuromuscular activity if actively inhibited (not shown). The sensory neurons of the genital bristles (dashed grey) innervate the abdominal ganglion and the subesophageal zone of the brain, and also connect with both the motor neurons and the inhibitory neurons. This likely aids the male in adopting the correct posture to copulate successfully. It is also possible that stimulation of the bristles activates the sensory neurons (dashed grey line) and may have some hedonic value for the male fly. The claspers, complete with bristles, are only shown on one side. (B) An active doublesex-positive motor neuron (solid blue line) causes the protractor muscle to contract, while an inhibitory interneuron (solid red line) inhibits the motor neuron (dashed blue line) connected to the retractor muscles. This extends the penis and its branches. The muscles with no designated colour have unknown functions, and the protractor muscles are only depicted on one side. Schematics of the genitalia were kindly provided by Janice J Ting.
Finally, Pavlou et al. also report that the circuit that controls the muscles that move the penis is independent of the one that controls ejaculation. This indicates that the control of copulation is separate from that of reproduction in male vinegar flies. It also hints that copulation should be considered behaviour in its own right, and possibly one with hedonic value (in other words, one that male vinegar flies might ‘enjoy’).
A number of outstanding questions remain. For example, does an equivalent circuit control the genitalia of female flies during copulation? Moreover, how do the results of Pavlou et al. link to recent studies that explored the neural circuitry controlling the persistence and motivation of male flies during copulation (Crickmore and Vosshall, 2013; Zhang et al., 2016)? And how do male and female flies coordinate their behaviour to increase the chances of successful reproduction?
References
-
Visualizing glutamatergic cell bodies and synapses in Drosophila larval and adult CNSThe Journal of Comparative Neurology 508:131–183.https://doi.org/10.1002/cne.21670
-
Courtship behavior of brain mosaics in DrosophilaJournal of Neurogenetics 12:205–226.https://doi.org/10.3109/01677069809108559
-
Control of male reproductive behavior by the central nervous system of Drosophila: dissection of a courtship pathway by genetic mosaicsGenetics 92:437–457.
-
Drosophila melanogaster's history as a human commensalCurrent Biology 17:R77–81.https://doi.org/10.1016/j.cub.2006.12.031
-
Sexual selection: lessons from hermaphrodite mating systemsIntegrative and Comparative Biology 46:349–367.https://doi.org/10.1093/icb/icj041
-
Neurogenetics of courtship and mating in DrosophilaAdvances in Genetics 62:67–184.https://doi.org/10.1016/S0065-2660(08)00603-2
Article and author information
Author details
Publication history
Copyright
© 2016, Levine
This article is distributed under the terms of the Creative Commons Attribution License, which permits unrestricted use and redistribution provided that the original author and source are credited.
Metrics
-
- 2,245
- views
-
- 184
- downloads
-
- 1
- citations
Views, downloads and citations are aggregated across all versions of this paper published by eLife.
Download links
Downloads (link to download the article as PDF)
Open citations (links to open the citations from this article in various online reference manager services)
Cite this article (links to download the citations from this article in formats compatible with various reference manager tools)
Further reading
-
- Neuroscience
The concept that dimeric protein complexes in synapses can sequentially replace their subunits has been a cornerstone of Francis Crick’s 1984 hypothesis, explaining how long-term memories could be maintained in the face of short protein lifetimes. However, it is unknown whether the subunits of protein complexes that mediate memory are sequentially replaced in the brain and if this process is linked to protein lifetime. We address these issues by focusing on supercomplexes assembled by the abundant postsynaptic scaffolding protein PSD95, which plays a crucial role in memory. We used single-molecule detection, super-resolution microscopy and MINFLUX to probe the molecular composition of PSD95 supercomplexes in mice carrying genetically encoded HaloTags, eGFP, and mEoS2. We found a population of PSD95-containing supercomplexes comprised of two copies of PSD95, with a dominant 12.7 nm separation. Time-stamping of PSD95 subunits in vivo revealed that each PSD95 subunit was sequentially replaced over days and weeks. Comparison of brain regions showed subunit replacement was slowest in the cortex, where PSD95 protein lifetime is longest. Our findings reveal that protein supercomplexes within the postsynaptic density can be maintained by gradual replacement of individual subunits providing a mechanism for stable maintenance of their organization. Moreover, we extend Crick’s model by suggesting that synapses with slow subunit replacement of protein supercomplexes and long-protein lifetimes are specialized for long-term memory storage and that these synapses are highly enriched in superficial layers of the cortex where long-term memories are stored.
-
- Neuroscience
Gamma oscillations in brain activity (30–150 Hz) have been studied for over 80 years. Although in the past three decades significant progress has been made to try to understand their functional role, a definitive answer regarding their causal implication in perception, cognition, and behavior still lies ahead of us. Here, we first review the basic neural mechanisms that give rise to gamma oscillations and then focus on two main pillars of exploration. The first pillar examines the major theories regarding their functional role in information processing in the brain, also highlighting critical viewpoints. The second pillar reviews a novel research direction that proposes a therapeutic role for gamma oscillations, namely the gamma entrainment using sensory stimulation (GENUS). We extensively discuss both the positive findings and the issues regarding reproducibility of GENUS. Going beyond the functional and therapeutic role of gamma, we propose a third pillar of exploration, where gamma, generated endogenously by cortical circuits, is essential for maintenance of healthy circuit function. We propose that four classes of interneurons, namely those expressing parvalbumin (PV), vasointestinal peptide (VIP), somatostatin (SST), and nitric oxide synthase (NOS) take advantage of endogenous gamma to perform active vasomotor control that maintains homeostasis in the neuronal tissue. According to this hypothesis, which we call GAMER (GAmma MEdiated ciRcuit maintenance), gamma oscillations act as a ‘servicing’ rhythm that enables efficient translation of neural activity into vascular responses that are essential for optimal neurometabolic processes. GAMER is an extension of GENUS, where endogenous rather than entrained gamma plays a fundamental role. Finally, we propose several critical experiments to test the GAMER hypothesis.