The gating cycle of a K+ channel at atomic resolution
Figures
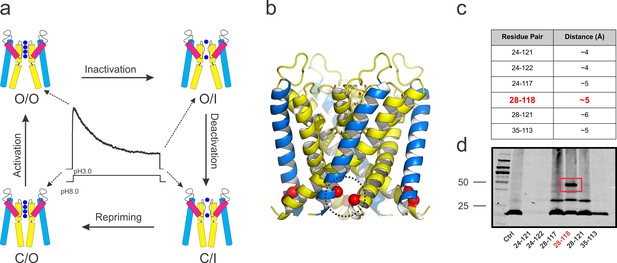
KcsA’s kinetic cycle and the biochemical strategy to trap of KcsA open gating intermediates.
(a) pH-dependent macroscopic current recorded from asolectin liposomes containing KcsA wild type, surrounded by cartoon representations of the structural equivalents for the gating intermediates. KcsA’s gating cycle comprises at least four distinct kinetic states defined by gating intermediates in which the selectivity filter is conductive (O) or collapsed (I) and the activation gate is open (O) or closed (C). Transitions between these kinetic states define four different gating processes: C/O→O/O, that is, activation; O/O→O/I, that is, C-type inactivation; O/I→C/I, that is, deactivation and C/I→C/O, that is, repriming or recovery from C-type inactivation. (b) side views of KcsA’s O/I low-resolution structure (PDB, 3F5W) displaying the region of the channel that was tested for inter-subunit disulfide-bond formation (red spheres). (c) table indicating all the putative residue-pairs tested for cysteine-bridge formation. In red, the pair 28–118 spontaneously formed disulfide-bonds. (d) A western blot showing that the residue pair 28–118 formed a covalently linked tetramer upon introduction of cysteine residues at those positions (the samples were heated in the absence of a reducing agent, non-reducing conditions).
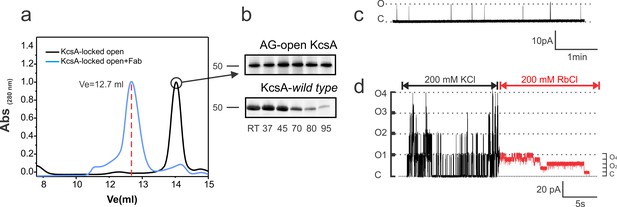
Biochemical and functional characterization of the Locked-open KcsA.
(a) Hydrodynamic properties of the disulfide-bonded KcsA-OM (Locked-open KcsA) were assessed by analytical size exclusion chromatography in a Superdex HR200 column. The chromatogram shows, in black, the elution volume for the chymotrypsin truncated Locked-open KcsA and in blue, in complex with a Fab fragment (Ve = 12.7 ml) required for crystallization trials. (b) Since the Locked-open-KcsA is covalently linked by disulfide bonds, its tetrameric state is remarkably resistant to high temperature (upper panel), when compared to KcsA wild type (lower panel). Melting experiments were conducted for 30 min at the temperature indicated in the absence of a reducing agent. (c) A representative single-channel trace of the Locked-open KcsA channel in 200 mM KCl, displays a very low-open probability, which agrees with it being an open and C-type inactivated channel. (d) An excised multi-channel patch of the Locked-open KcsA displays the characteristic low-open probability mode and large single- channel conductance when K+ was the permeant ion, 200 mM KCl (Black). After rapidly switching the bath solution to Rb+ as the permeant ion, 200 mM RbCl (Red), the channel switches to a high-open probability mode and small single-channel conductance.
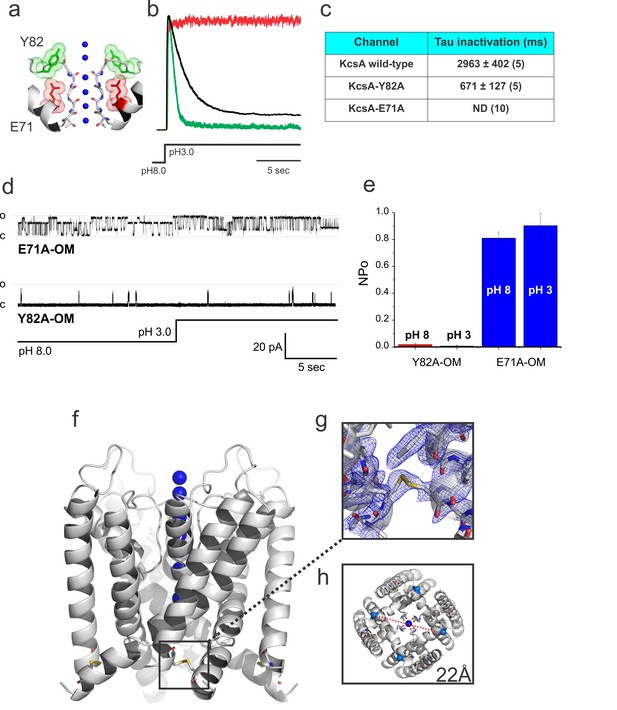
Crystal structure of the Locked-open KcsA.
(a) A cartoon representation of KcsA’s selectivity filter indicating mutated positions used in this study that either accelerates (Y82A in green) or removes (E71A in red) C-type inactivation. (b) Representative macroscopic currents recorded in symmetric 200 mM KCl during a pH fast-switching experiment for: the wild type channel (black), the fast-inactivating mutant Y82A (green) and the non-inactivating E71A mutant (red). (c) Tau for inactivation of KcsA wild type, Y82A and E71A mutants after a pH jump experiment (reported values are an average of the number (n) of independent observations indicated in parenthesis). (d) Representative 30 s. single-channel recordings of the Locked-open E71A (upper trace) and Y82A (lower trace) mutants, the pH of the bath solution was switched from 8 to 3 after 15 s. of recording by using a fast perfusion system. (e) The number of channel x open probability (NPo) of the Locked-open channel, as predicted, was pH insensitive but high for the E71A (~0.8–0.9) and low for the Y82A mutants (~0.015–0.04) Reported values are means ±SEM of 3 independent experiments. (f) A cartoon representation of the Locked-open KcsA X-ray structure highlighting the location of the inter-subunit disulfide bond between introduced cysteine residues at position 28 and 118 (g) 2Fo-Fc electron-density map (cyan, contoured at 1.0σ) of the Locked-open KcsA validating the formation of the 28cys-118cys disulfide bond. (h) An intracellular view displaying an activation gate opening of 22 Å (measured between Threonine 112 α-carbons on diagonal subunits).
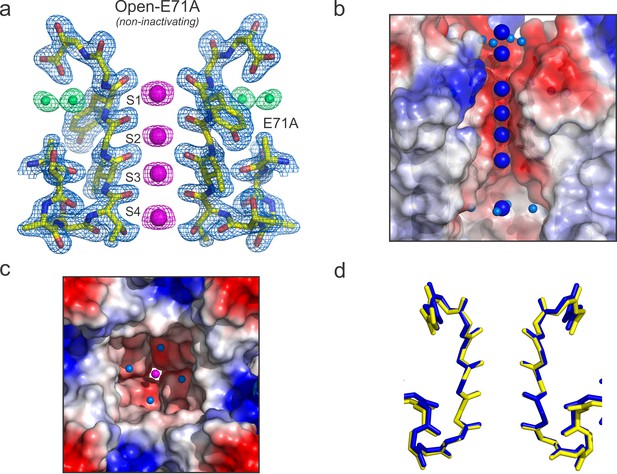
High-resolution structure of the open and conductive state of KcsA.
(a) X-ray structure of the Locked-open KcsA E71A mutant, determined @ 2.25 Å resolution. Displayed is the 2Fo-Fc electron-density map (light-blue mesh contoured at 2.5σ) for the selectivity filter residues 70–80 from two diagonally symmetric subunits, colored in yellow with oxygen atoms in red; for the K+ ions within the filter (magenta mesh contoured at 2.6σ) and for two water molecules located behind the channel’s selectivity filter that are occupying the vacancy created by the glutamate to alanine substitution (green mesh contoured @ 3σ). (b) Side view of a surface representation of KcsA O/O state (Locked-open-E71A mutant) highlighting the K+ ions within the channel pore. At the extracellular side two K+ ions are observed, one of them (the most internal) is partially dehydrated and coordinated by four water molecules. Similar to the closed KcsA structure (1K4C), the O/O state also contains a K+ ion within the channel central cavity but in contrast to the closed state in which is coordinated by eight water molecules, the ion is partially dehydrated and tetra-coordinated. (c) Intracellular view of the KcsA O/O state showing a K+ ion, inside the channel central cavity, partially dehydrated and coordinated by four water molecules (d) Structural alignment of the selectivity filters of the Locked-open KcsA E71A mutant (PDB 5VK6) in blue and the closed-KcsA in yellow (PDB 1K4C). The atomic deviation between the peptide backbones of the two filters (r.m.s.d.) is 0.19 Å.
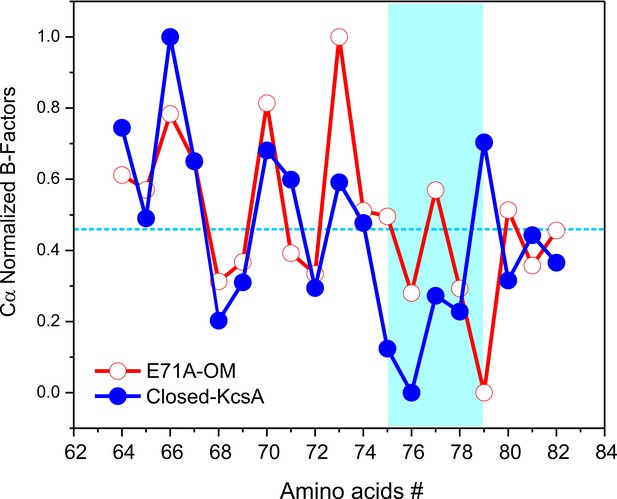
Crystallographic normalized B-factors for KcsA pore helix and selectivity filter alpha-carbons (Cα).
Changes of the normalized crystallographic B-factors of KcsA’s pore helix and selectivity filter residues (signature sequence) during activation gating are best represented by: (a) KcsA closed-primed-to-conduct or C/O state structure (new structure solved at the same resolution and using the same parameters during refining than for the O/O state structure) and KcsA open-conductive or O/O state structure (PDB 5VK6).
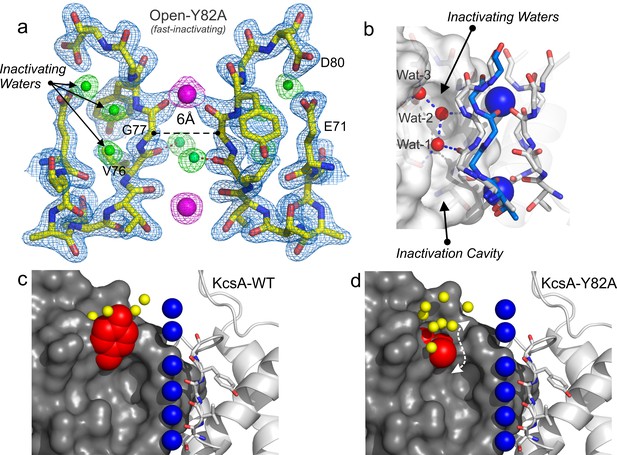
Structure of KcsA open and deep C-type inactivated.
(a) X-ray crystal structure of the Locked-open open and fast inactivating Y82A mutant @ 2.37 Å resolution (PDB 5VKE). Shown is the 2Fo-Fc electron-density map for the selectivity filter residues 71–80 from two diagonally symmetric subunits, colored in yellow with oxygen atoms in red (light-blue mesh contoured at 2.6σ); for the two remaining ions in the filter (magenta mesh contoured at 3σ) and for three water molecules, ‘inactivating waters’, located inside a cavity behind KcsA filter (green mesh contoured at 3σ). The Cα-Cα distance at position Gly 77 measured from diagonal subunits is 6 Å, narrowing KcsA’s filter ~2 Å. (b) Selectivity filters of the closed-KcsA (PDB 1K4C) colored in blue and oxygen atoms in red as a reference and of the Locked-open KcsA fast-inactivating Y82A mutant (PDB 5VKE) colored in white and oxygen atoms in red. The peptide backbone carbonyl groups from the Locked-open KcsA Y82A mutant flips away from the channel’s axis of symmetry moving sideways into a cavity, (b) ‘inactivation cavity’, which is located behind the channel’s filter of a neighboring subunit. This network of water molecules holds the filter in the inactive or flipped conformation. (c) Cartoon representation of KcsA wild type (PDB 1K4C), colored in white with oxygen atoms in red, as a reference and on the left a surface representation of a neighboring subunit in which the entryway of the inactivation cavity is guarded by Tyr 82 (in red), which is positioned to putatively regulate the rate and the extent of C-type inactivation by steric regulation and or/hydrophobic effect of the diffusion of water molecules (yellow spheres) into the ‘inactivation cavity’. (d) X-ray crystal structure of the Y82A mutant in the closed state @ 2.25 Å resolution. Substitution of the bulky and hydrophobic tyrosine at position 82 by alanine not only eliminates the steric limitation of water flow into the inactivation cavity (dashed arrow) but it solvates the entryway of the cavity, as denoted by the large number of crystallographic water molecules identified at this region of the structure (yellow spheres).
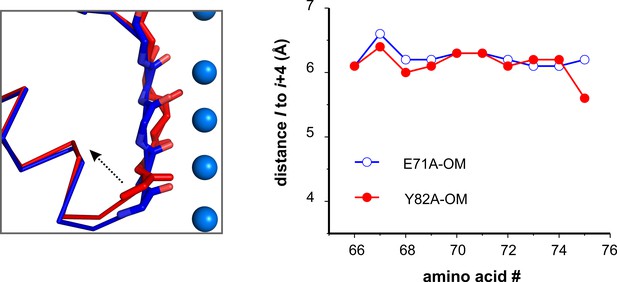
KcsA O/I state and the compression of its pore helix last turn.
Left, A cartoon representation of a structural alignment of the pore helix and the selectivity filter of the Locked-open KcsA, E71A and Y82 mutants in blue and red, respectively, a dashed arrow highlights the compression of the pore helix. Right. A plot of the distance I to i + 4 of the pore helix residues shows the compression of its last turn when KcsA is open and inactivated.
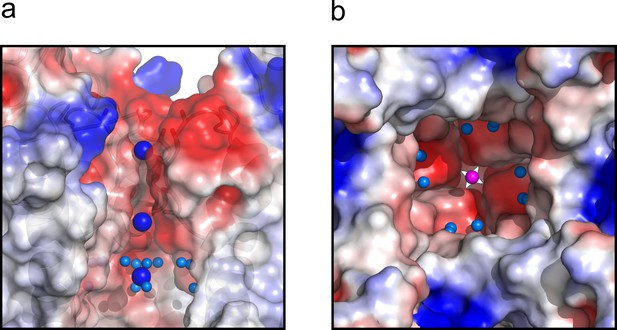
The central cavity of the KcsA O/I state.
(a) A side view of a surface representation of KcsA O/I state or Locked-open KcsA Y82A mutant crystal structure. KcsA O/I state seems to hold a K+ ion (blue sphere) with no discernible coordinating water molecules surrounding it. (b) An intracellular view of the central cavity displays a large number of crystallographic water molecules (cyan spheres) by comparison to the cavity of the O/O state or Locked-open KcsA E71A mutant.
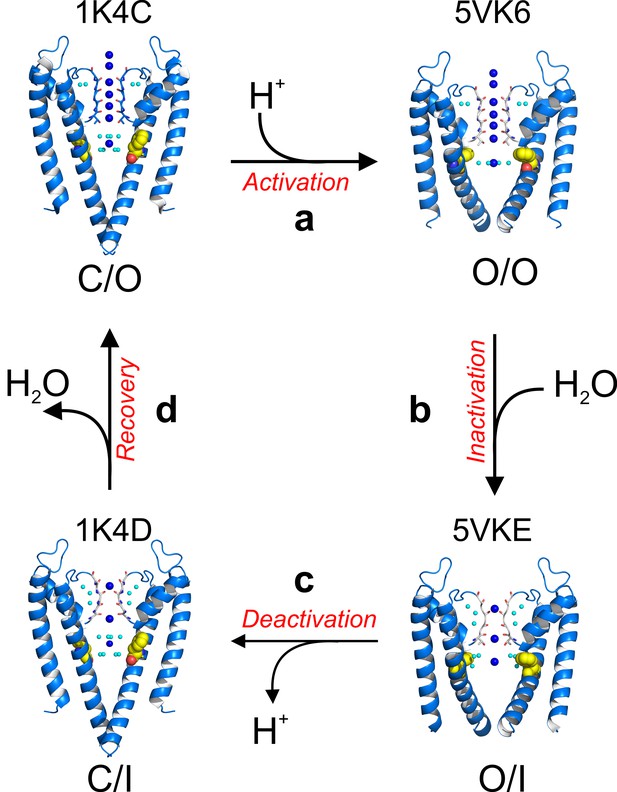
A structure-driven kinetic cycle for KcsA gating.
The simplest KcsA kinetic cycle contains at least four distinct states, closed and prime-to-conduct (C/O), open and conductive (O/O), open and C-type inactivated (O/I) and closed and C-type inactivated (C/I). KcsA resting state (C/O) is best represented by the structure of its closed state in high-potassium concentration (PDB 1K4C). (a) Upon proton activation, the channel undergoes conformational changes at its activation gate, increasing its diameter to 22 Å, which allows K+ ions to flow down their electrochemical gradient for about 200 ms, just before the onset of C-type inactivation. The Locked-open KcsA E71A mutant (PDB 5VK6) likely represents this short-lived open and conductive state (O/O). (b) A strong allosteric coupling between KcsA’s activation gate and its filter determines the duration of its O/O state. Opening of the channel activation gate is communicated to the filter by mechanical deformation that propagates along a network of interacting amino-acid residues along the second membrane-spanning segment. Among many, Phe 103 undergoes conformational changes (yellow spheres) that make it clash with Thr 75 on the channel’s pore helix, triggering a series of structural rearrangement at the channel’s filter that yield a pore with two consecutive ion vacancies and collapsed or deep C-type inactivated. The Locked-open KcsA Y82A mutant structure (PDB 5VKE) recapitulates the most important features of KcsA’s O/I state. (c) Decreasing intracellular proton concentration triggers KcsA deactivation gating, which involves structural rearrangements that close the channel’s activation gate. For a brief period of time, KcsA exists with a collapsed filter and its activation gate closed. The structural model that best describes KcsA’s C/I state is the closed structure in low K+ concentration (PDB 1K4D). (d) Once the KcsA activation gate is closed, the filter remains collapsed for an undetermined period of time, though computational calculations have suggested that the exit of the ‘inactivating waters’ from the ‘inactivation cavity’ determines the recovery rate of the channel’s filter.
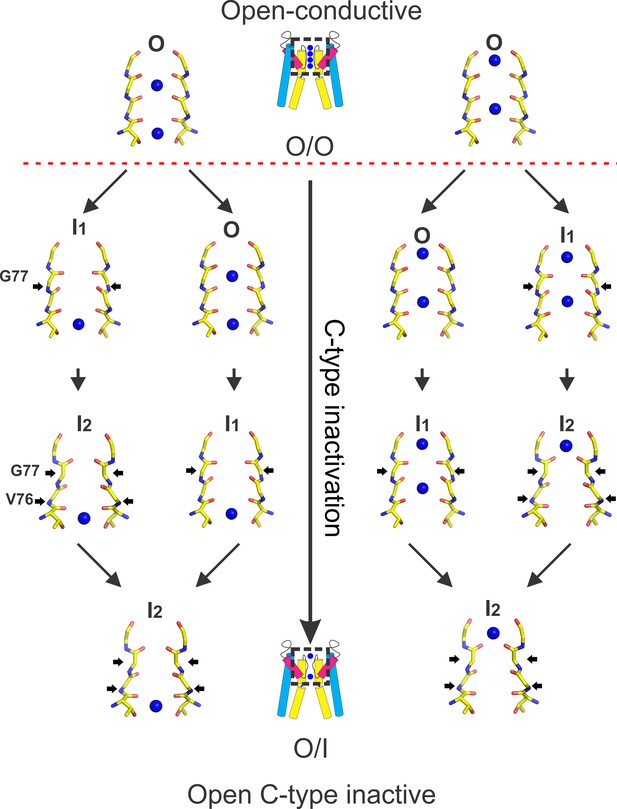
A structural explanation of C-type inactivation gating at the KcsA’s selectivity filter.
On the left panel, a cartoon representation showing the structural transition of KcsA’s selectivity filter once its activation gate is open. The right panel (top) shows a crystallographic view of KcsA’ selectivity filter in the conductive conformation (O/O) and the bottom panel in a deep C-type inactivated conformation (O/I). In between, two different inactivated states occur: I1 (an early-inactivated state) and I2 (a deep-inactivated state), which have been inferred from crystal structures of kinetic intermediates represented by KcsA’s partially-open structures (Cuello et al., 2010b). The onset of C-type inactivation is marked by the narrowing of the permeation pathway at Gly77, which is triggered by a strong allosteric coupling between KcsA’s selectivity filter and its activation gate (dashed red line). KcsA’s activation gate opening perturbs the conformational stability of the conductive selectivity filter by a conformation wave of mechanical deformation that travels along the channel inner helix (activation gate→selectivity filter)(Cuello et al., 2010a; Pan et al., 2011; Panyi and Deutsch, 2006; Zhuo et al., 2016). For channels on the left side, with ions at positions 2 and 4, an initial narrowing of the permeation pathway at G77 leads to the loss of the K+ at position 2. Further narrowing of the permeation pathway yields the deep C-type inactivated state (I2) in which the carbonyl at Val76 adopt a flip conformation with an ion bound at position 4. Channels on the right side, which have K+ at positions 1 and 3 would stay conductive during an early narrowing at G77, albeit with lower single-channel conductance, yet additional constricting at that position causes the exit of the K+ at position 3, yielding the deep C-type inactivated state I2 with an ion present at position 1.
Tables
Crystallographic table and refinement statistics.
https://doi.org/10.7554/eLife.28032.005Statistic | E71A-OM | Y82A-OM | Y82A-F103A closed |
---|---|---|---|
Data Collection | |||
Space Group | I4 | I4 | I4 |
Cell Dimension | |||
a = b, c (Å) | 156.54, 74.61 | 156.19, 74.17 | 155.73, 76.225 |
α=β=γ (°) | 90 | 90 | 90 |
Resolution (Å) | 24.75–2.25 (2.33–2.25) | 36.82–2.37 (2.45–2.37) | 42.91–2.25 (2.33–2.25) |
Rmerge | 0.052 (0.38) | 0.059 (0.46) | 0.079 (0.26) |
I/σI | 29.3 (3.9) | 29.53 (2.9) | 15.8 (2.9) |
Completeness (%) | 95.11 (99.70) | 99.76 (99.24) | 98.43 (91.97) |
Redundancy | 4.6 (5.0) | 4.5 (4.4) | 4.5 (4.4) |
Refinement | |||
No. reflections | 42598 (4255) | 36377 (3605) | 42773 (3985) |
Rwork | 0.186 (0.228) | 0.194 (0.239) | 0.174 (0.219) |
Rfree | 0.213 (0.262) | 0.226 (0.265) | 0.203 (0.234) |
No. atoms | 4088 | 4054 | 4386 |
Protein | 3939 | 3926 | 4036 |
Ligand/ion | 7 | 3 | 6 |
Waters | 101 | 84 | 218 |
Other ligands | 48 | 44 | 47 |
Protein residues | 529 | 528 | 535 |
Bond lengths (Å) | 0.003 | 0.003 | 0.01 |
Bond angles (°) | 0.64 | 0.65 | 1.02 |
Wilson B-factor | 50.05 | 51.10 | 30.63 |
Average B-Factor, Å2 | 69.09 | 67.94 | 39.39 |
Protein | 69.22 | 68.08 | 38.93 |
Ligands | 79.42 | 76.41 | 49.72 |
Water | 59.26 | 75.05 | 45.37 |
Ramachandran Favored (%) | 97.35 | 95.97 | 97 |
Ramachandran outliers (%) | 0.19 | 0.38 | 0.19 |
-
*Highest resolution shell is show in parenthesis.
†Data sets were collected from a single crystal.
Additional files
-
Transparent reporting form
- https://doi.org/10.7554/eLife.28032.013